[Approche théorique pour les défauts ponctuels dans une monocouche de dichalcogénure de métal de transition : calculs de conductance et de force dans le MoS
We present here a small review on our exhaustive theoretical study of point defects in a MoS
Nous présentons ici une mini-revue de nos différents travaux sur l’étude théorique des défauts dans une monocouche de MoS
Publié le :
Mots-clés : Structure électronique, Défauts, MoS
César González 1, 2 ; Yannick J. Dappe 3
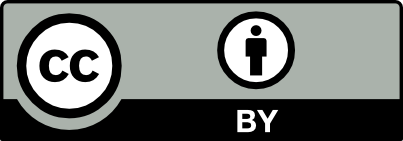
@article{CRPHYS_2021__22_S4_23_0, author = {C\'esar Gonz\'alez and Yannick J. Dappe}, title = {Theoretical approach to point defects in a single transition metal dichalcogenide monolayer: conductance and force calculations in {MoS}$_{{2}}$}, journal = {Comptes Rendus. Physique}, pages = {23--41}, publisher = {Acad\'emie des sciences, Paris}, volume = {22}, number = {S4}, year = {2021}, doi = {10.5802/crphys.72}, language = {en}, }
TY - JOUR AU - César González AU - Yannick J. Dappe TI - Theoretical approach to point defects in a single transition metal dichalcogenide monolayer: conductance and force calculations in MoS$_{{2}}$ JO - Comptes Rendus. Physique PY - 2021 SP - 23 EP - 41 VL - 22 IS - S4 PB - Académie des sciences, Paris DO - 10.5802/crphys.72 LA - en ID - CRPHYS_2021__22_S4_23_0 ER -
%0 Journal Article %A César González %A Yannick J. Dappe %T Theoretical approach to point defects in a single transition metal dichalcogenide monolayer: conductance and force calculations in MoS$_{{2}}$ %J Comptes Rendus. Physique %D 2021 %P 23-41 %V 22 %N S4 %I Académie des sciences, Paris %R 10.5802/crphys.72 %G en %F CRPHYS_2021__22_S4_23_0
César González; Yannick J. Dappe. Theoretical approach to point defects in a single transition metal dichalcogenide monolayer: conductance and force calculations in MoS$_{{2}}$. Comptes Rendus. Physique, Recent advances in 2D material physics, Volume 22 (2021) no. S4, pp. 23-41. doi : 10.5802/crphys.72. https://comptes-rendus.academie-sciences.fr/physique/articles/10.5802/crphys.72/
[1] Electric field effect in atomically thin carbon films, Science, Volume 306 (2004) no. 5696, pp. 666-669 | DOI
[2] Graphene on Rh(111): scanning tunneling and atomic force microscopies studies, Appl. Phys. Lett., Volume 100 (2012) no. 24, 241606
[3] Scanning tunneling microscopy characterization of the electrical properties of wrinkles in exfoliated graphene monolayers, Nano Lett., Volume 9 (2009) no. 12, pp. 4446-4451 | DOI
[4] The electronic properties of graphene, Rev. Mod. Phys., Volume 81 (2009), pp. 109-162 | DOI
[5] Effects of hexagonal boron nitride encapsulation on the electronic structure of few-layer MoS
[6] Hysteresis-free hexagonal boron nitride encapsulated 2D semiconductor transistors, NMOS and CMOS inverters, Adv. Electron. Mater., Volume 5 (2019) no. 2, 1800419
[7] 2D transition metal dichalcogenides, Nat. Rev. Mater., Volume 2 (2017), 17033 | DOI
[8] A review of molybdenum disulfide (MoS
[9] Conductive atomic force microscopy of semiconducting transition metal dichalcogenides and heterostructures, Nanomaterials, Volume 10 (2020) no. 4, 803 | DOI
[10] Two-dimensional MoS
[11] Probing magnetism in 2D van der Waals crystalline insulators via electron tunneling, Science, Volume 360 (2018) no. 6394, pp. 1218-1222 | DOI
[12] Measurement of lateral and interfacial thermal conductivity of single- and bilayer MoS
[13] Strong interfacial interactions induced a large reduction in lateral thermal conductivity of transition-metal dichalcogenide superlattices, RSC Adv., Volume 9 (2019), pp. 1387-1393 | DOI
[14] Van der waals heterostructures, Nature, Volume 499 (2013) no. 7459, pp. 419-425 | DOI
[15] Van der Waals heterostructures for optoelectronics: progress and prospects, Appl. Mater. Today, Volume 16 (2019), pp. 435-455 | DOI
[16] Devices and applications of van der Waals heterostructures, J. Semicond., Volume 38 (2017) no. 3, 031005
[17] et al. One-dimensional van der waals heterostructures, Science, Volume 367 (2020) no. 6477, pp. 537-542 | DOI
[18] Stability and electronic structures of native defects in single-layer MoS
[19] Intrinsic structural defects in monolayer molybdenum disulfide, Nano Lett., Volume 13 (2013) no. 6, pp. 2615-2622 | DOI
[20] et al. Exploring atomic defects in molybdenum disulphide monolayers, Nat. Commun., Volume 6 (2015) no. 1, 6293 | DOI
[21] Unusual reactivity of MoS
[22] Degradation behaviors and mechanisms of MoS
[23] Atomic adsorption of Sn on mechanically cleaved WS2 surface at room temperature, Surf. Sci., Volume 701 (2020), 121685 | DOI
[24] Structure and electronic properties of in situ synthesized single-layer MoS
[25] Impact of intrinsic atomic defects on the electronic structure of MoS2 monolayers, Nanotechnology, Volume 25 (2014) no. 37, 375703
[26] Electronic states of sulfur vacancies formed on a MoS2 surface, Japanese J. Appl. Phys., Volume 49 (2010) no. 8, 08LB01 | DOI
[27] Selective adsorption of thiol molecules at sulfur vacancies on MoS
[28] Native defects in bulk and monolayer MoS
[29] Low temperature nanoscale electronic transport on the MoS
[30] Gas adsorption on MoS
[31] Direct versus indirect band gap emission and exciton-exciton annihilation in atomically thin molybdenum ditelluride (MoTe
[32] Theoretical characterisation of point defects on a MoS2 monolayer by scanning tunnelling microscopy, Nanotechnology, Volume 27 (2016) no. 10, 105702 | DOI
[33] et al. Advances and applications in the FIREBALL ab initio tight-binding molecular-dynamics formalism, Phys. Status Solidi (b), Volume 248 (2011) no. 9, pp. 1989-2007 | DOI
[34] Multicenter approach to the exchange-correlation interactions in ab initio tight-binding methods, Phys. Rev. B, Volume 71 (2005), 235101 | DOI
[35] Ab initio multicenter tight-binding model for molecular-dynamics simulations and other applications in covalent systems, Phys. Rev. B, Volume 40 (1989), pp. 3979-3995 | DOI
[36] Simplified method for calculating the energy of weakly interacting fragments, Phys. Rev. B, Volume 31 (1985), pp. 1770-1779 | DOI
[37] Tight-binding models and density-functional theory, Phys. Rev. B, Volume 39 (1989), pp. 12520-12536 | DOI
[38] Optimized atomic-like orbitals for first-principles tight-binding molecular dynamics, Comput. Mater. Sci., Volume 39 (2007) no. 4, pp. 759-766 | DOI
[39] Theoretical study of carbon-based tips for scanning tunnelling microscopy, Nanotechnology, Volume 27 (2016) no. 10, 105201 | DOI
[40] WSXM: A software for scanning probe microscopy and a tool for nanotechnology, Rev. Sci. Instrum., Volume 78 (2007) no. 1, 013705 | DOI
[41] Helium bubble clustering in copper from first principles, Model. Simul. Mater. Sci. Eng., Volume 22 (2014) no. 3, 035019 | DOI
[42] Ab initio molecular dynamics for liquid metals, Phys. Rev. B, Volume 47 (1993), pp. 558-561 | DOI
[43] Efficient iterative schemes for ab initio total-energy calculations using a plane-wave basis set, Phys. Rev. B, Volume 54 (1996), pp. 11169-11186 | DOI
[44] From ultrasoft pseudopotentials to the projector augmented-wave method, Phys. Rev. B, Volume 59 (1999), pp. 1758-1775 | DOI
[45] Reactivity enhancement and fingerprints of point defects on a MoS
[46] Adsorption of small inorganic molecules on a defective MoS
[47] et al. Weakly trapped, charged, and free excitons in single-layer MoS
[48] et al. Charge density wave order in 1D mirror twin boundaries of single-layer MoSe2, Nature Phys., Volume 12 (2016) no. 8, pp. 751-756 | DOI
[49] Remarkably high thermal-driven MoS
[50] Forces and currents in carbon nanostructures: are we imaging atoms?, Phys. Rev. Lett., Volume 106 (2011), 176101 | DOI
[51] Atomic force microscopy as a tool for atom manipulation, Nat. Nanotechnol., Volume 4 (2009) no. 12, pp. 803-810 | DOI
[52] Complex patterning by vertical interchange atom manipulation using atomic force microscopy, Science, Volume 322 (2008) no. 5900, pp. 413-417 | DOI
[53] Adsorption of common solvent molecules on graphene and MoS
[54] Electronics and optoelectronics of two-dimensional transition metal dichalcogenides, Nat. Nanotechnol., Volume 7 (2012) no. 11, pp. 699-712 | DOI
[55] Large current modulation and spin-dependent tunneling of vertical graphene/MoS
[56] Theoretical insights into CO
[57] Molecular detection on a defective MoS
Cité par Sources :
Commentaires - Politique