1 Introduction
Agronomists have long been interested in crop diseases: they have known since historical times that epidemics caused by pathogenic agents are a major cause of annual variations in agricultural production and can even cause famine; the regulation of these diseases was one of the main reasons for crop rotation. However, since the 1960s, interest in this subject has tailed off considerably, because major advances in the field of pesticide science made it seem as though all such problems could be solved without having to worry about the effects of cropping practices on pathogens. Thus, agronomics tended to concentrate on optimising light interception, improving water efficiency, and managing nitrogen, as it was considered that research on diseases and their control should be carried out by phytopharmacists and plant pathologists. In parallel, the use of fungicides in agricultural practice increased substantially and each time that active ingredients became available, the fungicides became the basis for disease management strategies [1].
The renewed interest of agronomists for this question is due to the combination of:
- – the realisation of the collateral effects of the pesticide solution (air pollution, water pollution, selection of populations of fungicide-resistant pathogens);
- – economic constraints favouring a reduction in the use of inputs: farmers reduce the inputs on their crops, because the prices of agricultural products are tending to decrease (particularly those of arable crops, which were until recently strongly subsidised by European agricultural policy), and consumers are more and more interested in products guaranteeing a moderate usage or non usage of pesticides (organic or integrated farming);
- – the difficulty associated with basing the protection against diseases solely on genetic resistance, i.e. (i) efficient resistances are not available for all pathogens, (ii) to increase the useful life-span of available resistances it is necessary to decrease the selection pressure on pathogen populations by using other means as well.
The research over the last ten years, since these points became apparent, is based on close collaboration between agronomists and plant pathologists, and is aiming to understand and to predict the effects of cropping systems on plant disease. The object of these strategies is to develop methods of integrated crop protection to complement the means currently available [2,3].
Agronomists use the term ‘cropping system’ to refer to all of the techniques employed by a farmer on a plot. This covers many things including the choice of the succession of species cultivated, varieties of each species, soil tillage (ploughing, harrowing, hoeing...), dates and means of seeding, planting, pruning, thinning, the dates of application and doses of mineral or organic fertilisers, fungicides, herbicides, insecticides and growth regulators, dates and doses or irrigation, dates and means of harvesting... The word ‘system’ is used because these technical choices are not at all independent of each other [4,5]. For example, between 1975 and 1985, wheat producers brought their sowing date forward by three weeks (for example, in the Picardie region (France), the modal date changed from 25 to 5 October), for two major reasons: to increase the period of energy interception by the crop (wheat is a photoperiodic plant) and to reduce the risks of soil compaction by tillage in wet conditions at the end of the autumn. However, early sowing increases the risk of disease, because it favours autumnal infections, and this justifies the increased use of fungicides [6]; furthermore, sowing at the beginning of October corresponds to a period when aphids are still active and can transmit viruses (especially the Barley Yellow Dwarf Virus), creating a requirement for an extra insecticide treatment in the autumn. Clearly, sowing at such an early date was not possible before the development of efficient treatments against diseases and insects [1]. With the high price of wheat in the 1980s, the increased yield largely compensated the additional costs associated with extra pesticide treatments. This early sowing was one of the major causes of the decrease in cultivable area under maize, which is harvested too late to be grown before early sown wheat. Maize was replaced by peas and oil seed rape, which are harvested earlier. There is therefore a direct link between the date of sowing wheat, its protection and the crop that was harvested immediately beforehand, illustrating the coherence of the cropping system.
The cropping systems vary considerably from one plot to another and from one region to another. This is due to several factors:
- – the diversity of soils and climates: farmers adapt the choice of species and varieties according to the type of soil and climate, which also contribute to the risk of parasites;
- – the diversity requests for quality of agricultural products; for example, barley for animal feed and barley for malting are different varieties; to maximise the likelihood of having the correct protein content and the correct sized grains for malting, barley requires less nitrogen fertiliser and may require a different sowing date;
- – differences in regulations, which are becoming more and more linked to agroecological zoning and which favour some practices and may even impose others.
The role of agronomic research is to increase knowledge and to develop methods that farmers, agricultural technicians and food industry need to adapt cropping systems to these highly diverse situations. In this paper, we will discuss the effects of these practices on parasite life cycles, and then present research concerning the design of integrated crop protection strategies.
2 Effects of cropping systems on parasite life cycles
Unlike chemical methods, which essentially try to interrupt the life cycle of parasites when they could become harmful for crops, integrated crop protection targets all stages of their life cycle [3]. The effects of cropping systems on parasites have not been fully characterised, and we will therefore mainly discuss the most dramatic effects on some well-known pathosystems, particularly eyespot, take-all (which affect cereals) and grey-mould (which affects vines).
Firstly, cropping systems have a large effect on the size of the primary inoculum (coming from outside of the crop, unlike the secondary inoculum, which is produced by the diseased plants) and its localisation (i.e. its distance from plants that are susceptible to contamination). For diseases that can spread over long and medium distances in the air, the key factor is the proportion of sensitive plant in the neighbourhood. For diseases that can survive in the soil and only spread over very short distances, the primary inoculum depends on the cropping history of the field. The example of the combined effect of the crop rotation and tillage on eyespot of wheat illustrates this last point well. This disease, caused by Pseudocercosporella herpotrichoides (Fron) Deighton, leads to the necrosis of the base of wheat stalks; the inoculum remains on infected stalks; contamination occurs via the air when these stalks are at the surface, near to a young wheat plant. Although previous wheat has long been known to favour this disease, the effect of tillage, which is highly controversial, can only be understood if we consider its interaction with the type of plants previously grown [7]. Fig. 1 compares four wheat crops. In the first two cases, the crop immediately before the wheat crops have oil seed rape (which is not a host for this disease); however, the crop grown immediately before the oil seed rape was wheat. If the field is ploughed after harvesting the oil seed rape, infected wheat straw from two years previous that had been buried for one year, is brought to the surface. This makes the disease worse (see the percentage of plants presenting symptoms at heading in Fig. 1). However, when the preceding crop is wheat that was in turn preceded by a non-host culture (e.g., sunflower), the absence of ploughing means that residues carrying a fresh inoculum are left on the surface (in which case the disease would be more severe) but ploughing buries at least some of the infected residue, thus contaminating the wheat less in the study year. These results lead to the introduction of parameters linked to the cropping systems in epidemiological models; in Fig. 1, the values of the primary cycle parameter of the model of disease progression [8], follow the same logic as the level of attack observed on wheat at heading stage.
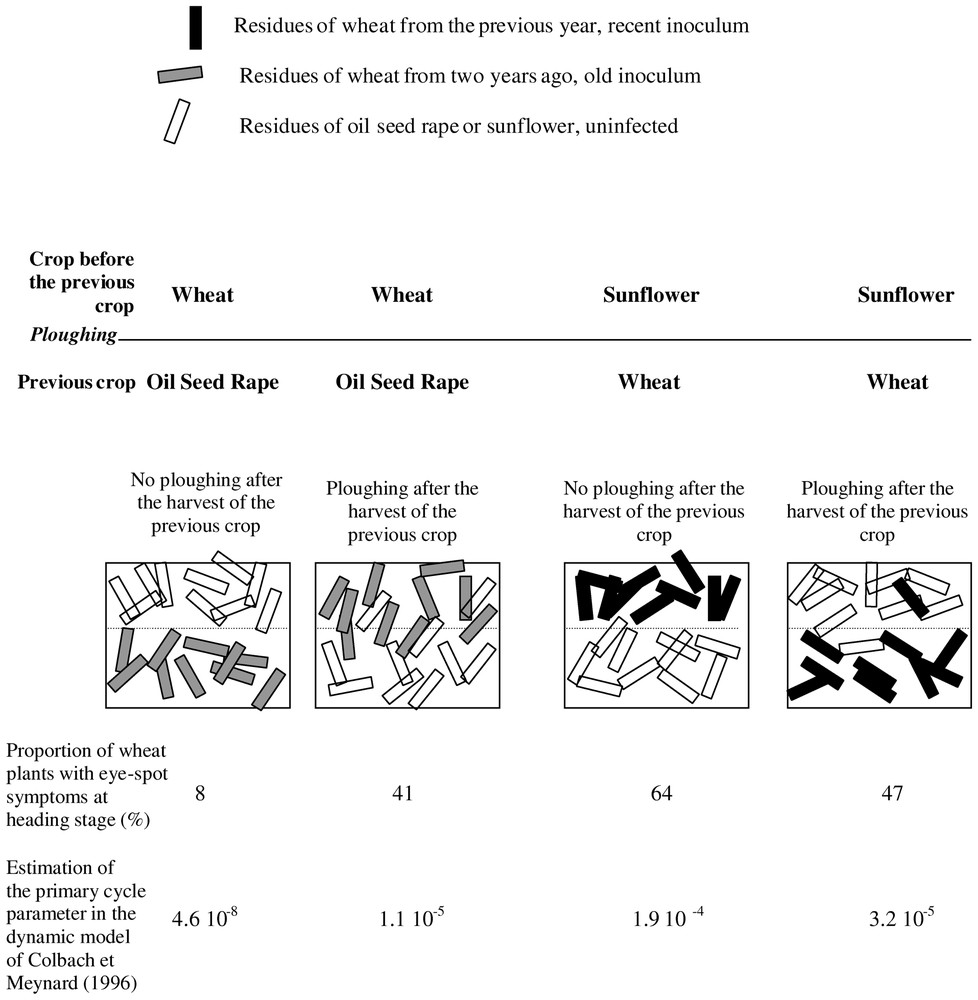
Effects of crop rotation and of soil tillage on the localisation of the inoculum of eyespot and on the development of the epidemic on a wheat crop.
The cropping system also affects the development and spread of epidemics. Studies on grey mould on vines, caused by Botrytis cinerea Pers:Fr, illustrate this point (Table 1). Thus, insecticides reduce populations of caterpillars Lobesia botrana Denis & Schiffermülmler, which are vectors of fungi and open the door to mycelium by creating plaques in grapes [9]. Tying, leaf-thinning and planting grass in the row aisles affect the arrangement of leaf surfaces and the microclimate within the cover, which conditions the length of time that aerial organs remain moist, and thus the germination of spores. Tying and leaf thinning facilitate the penetration of fungicide sprays [9–11]. However, we do not yet fully understand the interactions between these techniques in terms of their effect on the disease, even though such an understanding is necessary to evaluate whether they are complementary means of protection; this requires elucidating the modes of action, both for the determination of the microclimate of the grapes and for the physiology of the vine. For example, laying grass changes the radiative properties of the soil, and the amount of water and nitrogen available to the vine, which in turn affects the quality of the foliage, its disposition, the thickness of cuticles, the risk of grapes bursting and probably the induction of natural defences following abiotic stress [12].
Relative effects of different elements of the cropping system on the proportion of bunches of grapes affected by grey mould (following a treatment with fungicides). Adapted from [9–11]
Insecticide treatment | −50% |
Tying | −55% |
Planting grass in the row aisles | −57% |
Leaf-thinning | −60% |
Cropping systems also affect the coordination of the life cycle of cultivated plants and that of their parasites. The case of wheat diseases (for example eye-spot, and septoria), for which bringing forward the sowing date increases the frequency of autumn contamination, was discussed above: advancing the sowing date gives the parasite more time to develop in the plant and to affect sensitive organs. Inversely, advancing the sowing date of oil seed rape (to the beginning of August, instead of the end of August, which is the usual date in the Parisian area), allows the plants to reach a less sensitive stage at the time when spores of the black leg disease are likely to be released [13].
Finally, cropping systems can disrupt ecological equilibria (for example, within soil microflora), either favouring or disfavouring the pathogens. Fig. 2 summarises the results of a soil receptivity test for take-all (Gaeumannomyces graminis (Sacc.) Arx & Olivier var. tritici Walker), a disease that causes necrosis on the roots of cereals, for different ways of set-aside management. In 1992, the European agricultural policy introduced land set aside, making it obligatory for cereal producers not to sow a certain proportion of their land, with the aim of reducing production. Depending on the history of the plot and the techniques used, the set-aside plot can harbour very different flora. Three cases are compared in Fig. 2 (from [14]): bare soil, highly abundant wheat volunteers and blackgrass (Alopecurus myosuroides Huds), which is a common weed in cereal cropping systems. The test consists of adding various amounts of inoculum to earth from these three types of set aside and observing the extent of the disease on wheat plants growing in standard conditions. Without inoculum added, the disease is more severe for wheat plants growing on soil that was invaded by wheat volunteers, than for those growing on soil invaded by blackgrass, which is less sensitive and leaves less native inoculum in the soil than wheat. No disease was observed on wheat plants that were grown on bare soil. However, when inoculum is added, the severity of the disease is hardly any greater for plants grown after wheat volunteers, whereas it is very much greater for the other two cases; this is due to the development of a microflora inside the wheat rhizosphere that acts as an antagonist for take-all (in particular Pseudomonas fluorescens [15]), which slows down its development – this microflora is absent or poorly developed in the other two cases. Blackgrass is particularly ill fated, as it multiplies the inoculum of the pathogen, but does not multiply the antagonistic microflora.
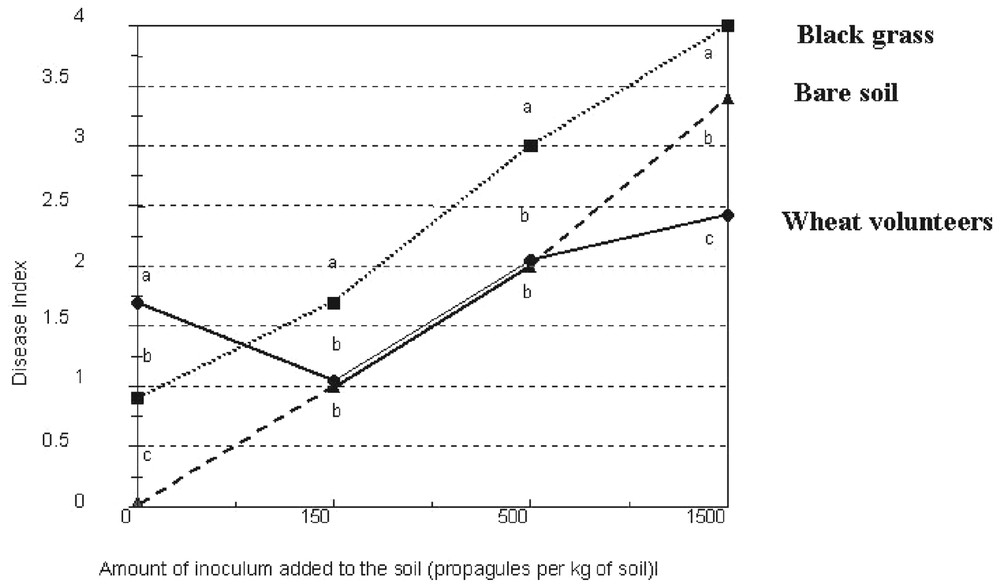
Receptivity of the soil to take-all as a function of the type of flora that preceded the wheat (from [14]). The index values for a given dose of inoculum that are followed by letters are significantly different (P<0.05; Student–Newman–Keuls test).
3 Conception of integrated crop-protection strategies
This knowledge of the effects of cropping systems on diseases is essential for the development of integrated crop-protection strategies, but is not sufficient: the techniques that influence the risk of disease also influence other processes, within the soil and plant, which sometimes have a large effect on production, both in terms of quantity and quality. Thus, the choice of a cropping system is a compromise between these different effects [16,17]. For example, tillage is currently being simplified worldwide (suppression of ploughing) with the aim of reducing costs and preventing erosion. However, when fields are not ploughed, crop residues remain on the soil surface, which favours some diseases, insects and slugs. The weed seeds, which are not sown deeply, germinate more readily. The consequence is an increased use of pesticides: fungicides, insecticides, molluscicides, and herbicides. The economic and environmental benefits of the suppression of ploughing can be ‘counterbalanced’ by prejudices, which vary according to the characteristics of the environment and the cropping systems. The identification of situations in which the benefits can be considered to outweigh the disadvantages requires an economic and environmental study for each case.
Economic studies make it necessary to translate the risk of disease into risk of loss of production. This is the aim of studies on the damage caused by fungal diseases to carbon assimilation, water uptake and plant morphogenesis [18–20]. However, such studies are rare, a consequence of the scarcity of research scientists interested in ecophysiological approaches of plant populations. Understanding the harm caused by diseases requires a dynamic approach to monitor the evolution of the epidemic and its effects on growth, development and production during the entire life cycle of the plant [21,22]. In this field, only a small number of studies have been completed all the way to modelling predictions of the damage caused by parasites to crop yield. For example, Fig. 3 (from [23]) shows that it is possible to predict the effect of take-all on the number of grains of wheat lost from an integral of the proportion of diseased plants between the beginning of stem elongation and flowering. The harmful effects of diseases can often be translated into a loss of quality of the harvest (technological or organoleptic characteristics, conservation properties, etc.). There is an urgent need to understand better the little studied consequences of agricultural practices on the development of pathogens that are toxic to men (e.g., cereal fusarium), which is an important aspect of food safety [24].
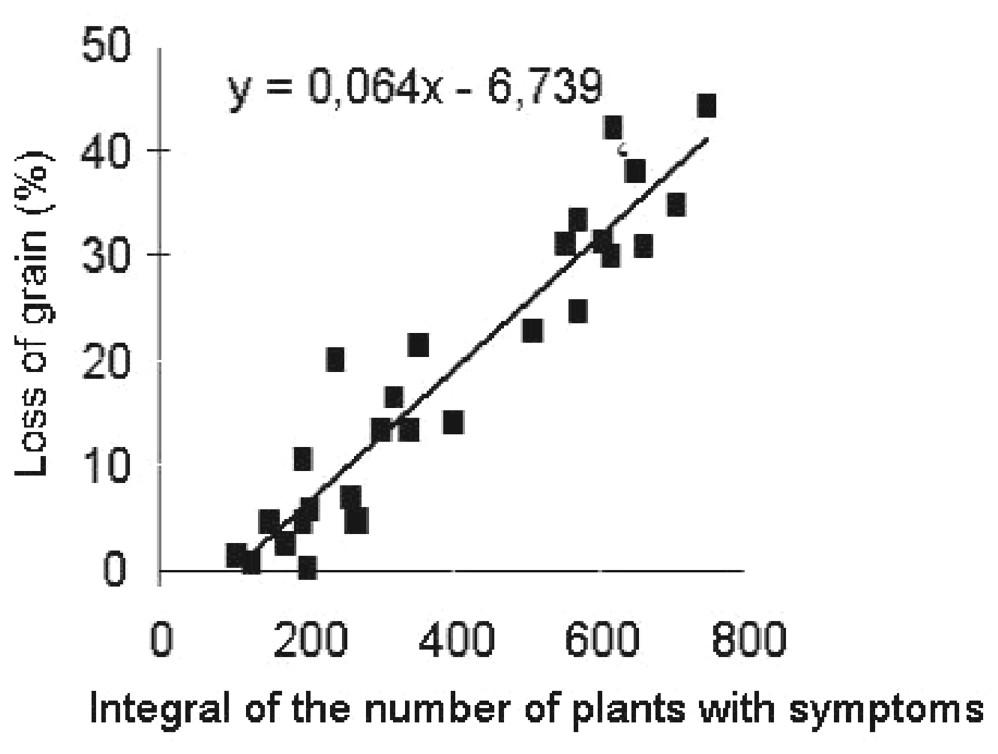
Modelling the damage caused by take-all disease on wheat: relationship between the loss of grain number and the integral of the proportion of plants presenting the symptoms of take-all between the start of stem elongation and flowering (from [23]).
The integrated protection of cereals against take-all is one of the rare case studies to be sufficiently advanced for us to envisage building a computer-based decision-support tool for the design of cropping systems. This tool, which is currently being developed by INRA, will be organised as follows (Fig. 4): input will be a climatic database and the characterisation of the cropping systems. The first module will estimate the kinetics of the progression of the disease [25]. This will then be used as input by two other models, the one outlined in Fig. 3 will calculate the loss of yield, and the other one will calculate the loss of nitrogen absorption and the protein content of the wheat grains. Simulating the disease development and production losses with the climatic data from the last thirty years, it will be possible to:
- – determine the probability that treating seeds against this disease is financially viable in a given situation;
- – decide the extent to which sowing can be delayed by comparing the increase in yield resulting from the reduction in disease with the decrease in yield due to the shortening of the cycle;
- – estimate whether, in a given year, the baking quality of the harvested grains risks being affected by disease.
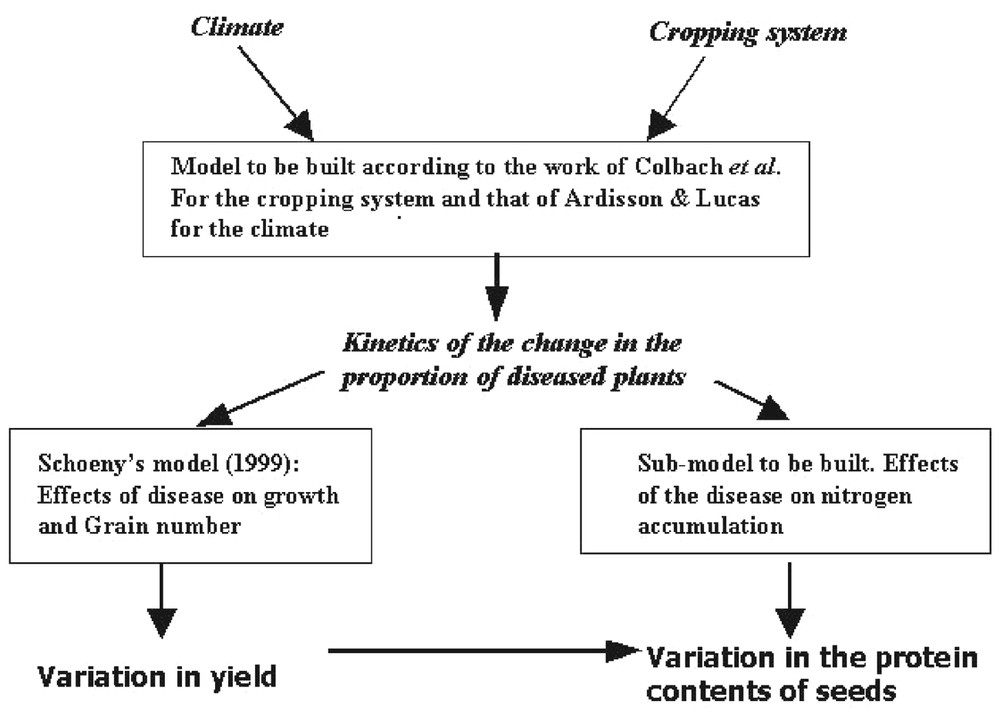
Our current state of knowledge concerning the damage caused by take-all on the wheat yield and the effects of cropping systems on the pathogen (from Ennaifar, Lucas, Meynard, work in progress).
By combining the information available for the different effects of agricultural techniques in this way, it begins to be possible to develop new cropping systems, in which non-chemical methods of preventing diseases are a priority [26]. Table 2 illustrates the development of new cropping systems for wheat, organised according to the principles of integrated crop protection [1,27]. In current cropping systems (1st column), where the primary objective is to obtain the maximum yield per hectare, the variety is chosen for its productivity. This prevents the use of the less productive varieties that may be more disease resistant. With the aim of maximising production, a high dose of nitrogen fertiliser is used, the sowing density is high and sowing is early. The high risks of disease associated with this strategy impose regular use of chemical protection (fungicides, insecticides, and growth regulators). In the innovative cropping system, a lower yield per hectare is accepted, making it possible to use disease-resistant varieties, lower the sowing density and the nitrogen requirements, and use less chemical agents to protect against eye-spot and leaf diseases. Although, as seen above, delaying the sowing date can reduce the risk of parasitism, we did not use this strategy in the integrated cropping system, given the preference of farmers for early sowing, which enables them to organise their work better and to optimise the soil structure. Numerical models simulating production and parasite damage make it possible to adapt the various techniques quantitatively and to the desired yield, and to define acceptable compromises between economic and environmental objectives [28,29].
Coherence between the reduction in the target yield and the reduction in input (from [1])
Target yield | Maximal yield () | Reduced yield () |
Sowing density and date | 240 plants m−2 (mid-October) | 160 plants m−2 (mid-October) |
Variety | High-yielding varieties, susceptible to disease | Variety chosen for resistance to diseases |
Nitrogen fertiliser | required, less supplied by soil | required, less supplied by soil |
Growth regulator | Systematic | None |
Fungicide | Preventative application of 2 or 3 treatments | Monitoring and curative application, 0 or 1 treatment |
The economic value of these new systems is currently being evaluated on a network of on-farm experiments throughout France (network led by four private wheat breeders, INRA, ITCF and several chambers of agriculture, between 1999 and 2002, supported by financing from the French Ministry of Agriculture). Table 3 summarises the preliminary results: 23 field experiments were carried out over two years in very varied conditions. In each experiment, four cropping systems using more or less input were tested with diverse varieties. Systems 1 and 3 are the same than in Table 2. System 4 is highly extensive and results in a smaller fertiliser requirement and the elimination of fungicides [30,31]. Table 3 only shows the results for two varieties: a highly productive variety, not very resistant to disease, and currently widely used in France (Isengrain) and a less productive, but highly resistant one (Oratorio). When the price of wheat is high (the price at the beginning of the 1990s), the systems that usually give the highest profit (18 or 19 times out of 23) combine a productive variety with a high level of inputs. When, like at the moment, the price of wheat is low, the best systems involve a disease-resistant variety and a low level of input. Cropping systems with a low level of input, based on integrated crop protection, also reduce the risk of polluting water with nitrate and pesticides; they do not lead to an increase in the variability of the profits or to a decrease in the quality of the products [16,30].
Economic results of a national network of trials of the reduction of input for winter wheat. Four cropping systems were compared for several varieties; the table indicates for each combination (cropping system × variety), the number of trials (out of 23) where the combination maximises the gross margin (from [30,31])
Price of wheat: 90 F/q=137 €/t | ||||
System | 1 | 2 | 3 | 4 |
Isengrain | 19 | 18 | 11 | 4 |
Oratorio | 13 | 16 | 16 | 11 |
Price of wheat: 50 F/q=76 €/t | ||||
System | 1 | 2 | 3 | 4 |
Isengrain | 11 | 12 | 14 | 10 |
Oratorio | 6 | 13 | 18 | 20 |
These studies, near to operational application, clearly show the complementarity between the pesticide, genetic and agronomic approaches for the management of diseases that affect cultivated plants. They were all carried out on the scale of the field and growing season and need to be completed by studies on other scales, integrating more completely the ‘long-term’ dimension of sustainable agriculture. Table 4 represents the crossing of spatial scales and time scales (from tactical adjustment in the short-term to long-term strategies), to which integrated protection must be added. In accordance with our current state of knowledge, the examples cited herein mostly correspond to cases 1 and 2 in this table: the adjustment of crop treatments as a function of the predicted damage caused by diseases, and the reduction of epidemics by combining resistant varieties with low risk cropping systems. Our understanding is less complete when it comes to designing long-term strategies (box 3 in the table), because of the complexity of the interactions involved, whether we are talking about perennial cultures (see the example of grey mould) or telluric diseases, due to the difficulty in applying ecological approaches to the rhizosphere.
Implementation of the integrated protection methods (from [3]).
Plot (1–10 ha) | Group of plots (100–1000 ha) | Region (104–105 ha) | |
Tactical adjustment | (1) Adjust treatments as a function of the predicted damage caused by diseases and unintentional effects. | (4) Choose ‘guide plots’ that will be monitored to identify risks. | (7) Predict the risks of parasites according to the climate. |
Yearly strategy (crop level) | (2) Reduce epidemics and the damage caused by them by choosing appropriate crop management and varieties. | (5) Control nearby contaminations and diversify the sources of genetic resistance. | (8) Diversify the sources of genetic resistance. |
Long-term strategy (cropping system level) | (3) Reduce the inoculum of the disease by choosing appropriate cropping systems.Perennial cultures, as above. | (6) Control nearby contaminations. | (9) Coordinate methods of integrated protection to preserve the duration of resistance and the efficiency of active ingredients. |
Pathologists have for many years been trying to develop a reasoned approach to the tactical adjustment of treatment in groups of plots or in regions (boxes 4 and 7 in the table) [32]; we need to improve these analyses by furthering our understanding of the harm caused by diseases, and by investigations combining social sciences and biological sciences to determine how the different actors combine the information collected on different scales to give advices concerning treatment and to make decisions (Taverne and Cerf, work in progress). Cases 5, 6 and 8 involve the prevention of contamination between neighbouring plots. These processes are currently rarely taken into account in crop protection strategies. Indeed, we need to improve considerably our understanding of both the dispersal of spores over medium and long distances and the role of cropping systems in the spatial propagation of epidemics. For example, if all farmers adopted the systems with low levels of input shown in Tables 2 and 3, would they still be effective? In particular, as diseases are less well controlled by agronomic methods than by chemical methods, would an increased inoculum in the air reduce the efficiency of agronomic methods? The ninth box in Table 4 refers to the preservation of resistance over long periods and the preservation of the efficiency of the active ingredients. It is clear that our knowledge of the effect of cropping systems on the genetics of disease populations will be a key element in the future. However, communication between population geneticists and agronomists is still in its infancy, and these questions need to be addressed in universities and research laboratories.
Studies on integrated crop protection are one of the sources of renewing the discipline of Agronomics, which, by enlarging the study aims and scope of action, will become true agrosystem engineering [5].