Abridged English version
Resistance of a plant species to a micro-organism is called non-host resistance, which is the most common situation. Major elements of this type of resistance are physical and chemical barriers like peptides, proteins and metabolites.
Other general mechanisms are activated if plants receive a stress signal. Systemic Acquired Resistance (SAR) is described as a general level of resistance towards any pathogen, following a first infection or a stress signal. SAR is correlated with the apparition of so-called PR proteins for ‘Pathogenesis Related’. Salicylic acid (SA) is an essential signal for the implementation of SAR. Induced Systemic Resistance or ISR is similar to SAR, but is specifically induced by rhizobacteria, which are not pathogenic. SA and PR proteins seem not to be involved in ISR.
A specific resistance mechanism is triggered by the recognition of a pathovar by a cultivar. It is based on a simple genetic mechanism: a dominant R allele in plants and an Avr allele are necessary to induce a cascade of signals resulting in (i) a local hypersensitive reaction that tends to limit pathogen growth, and (ii) the activation of general resistance to any pathogen. In the last ten years, several genes for R proteins have been isolated and cloned.
R proteins present homologies even among distant plant species. They can be classified into five classes. The most common class contains domains homologous to (i) proteins involved in programmed cell death among eucaryotes (NBS or Nucleotide binding Site), (ii) a motif involved in peptide–ligand interactions (LRR or Leucine Rich-Repeat) and (iii) a motif implicated in pathogen recognition among animal cells (Drosophila and human) the TIR domain. Two classes of R proteins have a transmembrane peptide: the exposed part of the protein outside the plant cell could constitute a detection system.
Avr proteins are very diverse. The only general feature is that they are generally hydrophilic. It may seem strange that micro-organisms possess proteins devoted to limit their own growth, but Avr proteins also play a key role in virulence.
Once R and Avr have interacted, a cascade of signals is triggered. It has been shown recently that in Arabidopsis thaliana, the precocious defence mechanism is relayed by three MAP (Mitogen-Activated Protein) kinases as has also been observed for the regulation of defence genes in Drosophila. Some chemical effects that have been shown to play important roles in signalling include influx of Ca2+ and H+, efflux of Cl− and K+, and bursts of H2O2 and probably nitric oxide, NO.
Some observations have been made that tend to confirm the hypothesis of a concerted evolution between R and Avr genes: (i) there is mega-clustering of R genes in the Arabidopsis genome, (ii) orthologs are more similar than paralogs, (iii) a series of detoxification enzymes from pathogens exist in response to toxic enzymes from the plants. These observations suggest that R genes duplicate, but also keep ancient alleles and that Avr and R are racing to circumvent each other, under the limitation of other constraints.
Selection for cultivars resistant to pathogens is a major challenge. Genetic resistances exist in wild ecotypes or in closely related species. Resistances exist in current cultivars where they have been introduced recently, since in older varieties the pathovar has had time to overcome the resistance. A number of resistance genes in wheat have their origin in related species like Aegilops ventricosa and Agropyron elongatum. In tomato, about 10% of the genome come from related species like Lycopersicon hirsutum, L. peruvianum and L. pimpinellifolium as a result of the introduction of resistance genes from these species. The direction of selection is very different among species: (i) in tomato, fruit quality is a major objective and not resistance; (ii) in lettuce, resistances to Bremia are quickly overcome and release of a new variety with a new resistance gene is a marketing scheme that may be detrimental to long-term resistance. A number of long-term resistances are documented: resistance to eyespot disease and yellow and brown rusts in wheat, resistance to virus X in potato... The environment can influence resistance: a stable resistance to anthracnose exists in bean in Europe, which is circumvented in Latin America.
With the recent progress in molecular genetics, we may start to consider applying bioengineering to the field of plant resistance to pathogens. One can introduce a resistance gene precisely into a variety without disturbing the whole genetic background that has been fixed for production features. Similarly, multiple resistances can be easily constructed: multiple lines each carrying a different resistance gene can be tested individually and then introduced sequentially.
Knowledge of the alleles of resistance and their sequences, which may be patented, will allow accurate record, keeping on resistance genes as well as follow-up on introduced resistances.
Some strategies already have been implemented in order to engineer resistance: (i) plants can be manipulated to constitutively express SAR, (ii) a gene coding for an anti-microbial protein (chitinase...) can be introduced into the plant genome, (iii) the isolation of a promoter inducible by any pathogen can be used, linked to a gene coding for an inductor of defence response. Another promising approach is to engineer plants to destroy the factor of pathogenicity of the pathogen (for example, oxalate oxydase/oxalic acid).
The induction of resistance requires quite a large number of genes between the recognition and the downstream effects of resistance. One can consider resistance as the expression of quantitative trait loci (QTL). Such QTLs have been isolated for mildew resistance in A. thaliana, anthracnose in bean... In A. thaliana, it is estimated that 1 to 2% of the genome-coding capacity could be involved in resistance.
Molecular markers should facilitate the selection for resistances, because recessive genes can be followed and large-scale test can be accomplished, without the implementation of field tests.
Our current knowledge on the mechanisms of resistance should permit the selection of cultivars with new resistances using biotechnology. On the other hand, the public rejection of biotechnology, particularly as regards food, is increasing, even though the controls in place are very strict. Biotechnology may also contribute to meeting another public demand: the lowering of chemical inputs and the durable management of the environment. Biologists still have to improve the durability of resistance. Monitoring resistance with molecular markers will facilitate this aspect.
1 Introduction
Quand sont évoquées les méthodes de lutte contre les maladies des végétaux cultivés, viennent d'abord à l'esprit les progrès considérables amenés par l'usage des produits phytosanitaires. Les pratiques agronomiques employées pour limiter l'impact des maladies, ainsi que les avancées permises grâce à la génétique sont déjà moins connues.
Un état des lieux des connaissances sur les mécanismes développés par les végétaux pour se protéger contre les maladies montre qu'ils agissent de la même manière vis-à-vis de la plupart des microorganismes. Certains sont constitutifs, d'autres sont induits plus ou moins rapidement par rapport à la progression du pathogène et seront donc plus ou moins efficaces. Pour l'essentiel le travail de sélection végétale, en matière de lutte contre les maladies, a porté sur les interactions spécifiques entre le pathogène et son hôte, qui conduisent à l'activation de ces mécanismes. En conséquence, le pathogène peut contourner ce type de résistances. Les progrès réalisés dans la protection contre les pathogènes grâce aux méthodes de la génétique sont loin d'être négligeables, et nous illustrerons les perspectives ouvertes à la fois par une connaissance approfondie des bases moléculaires de ces interactions et par les techniques du génie génétique.
2 Les relations plantes–pathogènes : état des connaissances
2.1 Résistance non hôte
Un premier constat trivial peut-être fait : la plupart des plantes sont résistantes à la plupart des pathogènes potentiels. Malgré la formidable diversité des micro-organismes, une faible fraction des interactions avec les plantes conduit à une pathologie plus ou moins avancée, allant parfois jusqu'à la mort de la plante.
La résistance de l'ensemble des populations ou variétés d'une espèce à un organisme par ailleurs pathogène est nommée « résistance non hôte » [1]. Elle met en jeu divers mécanismes constitutifs ou parfois même inductibles. Les barrières physico-chimiques constituent un élément essentiel de ce type de résistance, en empêchant le micro-organisme de pénétrer dans les organes de la plante ou dans ses cellules. Des composés préformés ou induits sont aussi impliqués. Ils peuvent être de diverses natures, comme des peptides, des protéines, des métabolites (composés terpéniques, phénoliques, alcaloı̈des, etc.) [2]. Par exemple, les saponines, qui dégradent les stérols membranaires des micro-organismes, sont ubiquitaires dans le monde végétal [3].
2.2 Résistance générale induite
Certains mécanismes généraux sont activés dans les plantes si elles perçoivent un signal de stress. Ce signal peut être physique, s'il s'agit d'une blessure, ou chimique, s'il s'agit de composés sécrétés par les pathogènes eux-mêmes ou générés, par exemple, par la digestion des parois végétales. Cette reconnaissance active différents mécanismes, pouvant aboutir à une résistance ou une tolérance lors d'une infection ultérieure par le même pathogène ou un pathogène différent. Les régulations et les imbrications de ces voies sont complexes et de nombreux travaux restent à faire afin de mieux les comprendre. Elles seront brièvement décrites ci-dessous.
2.2.1 La résistance systémique acquise ou SAR
Le phénomène de résistance systémique acquise [4] est connu depuis le début du XXe siècle. Alors qu'une infection est en cours, qu'elle se généralise dans la plante ou qu'elle conduise à la formation de lésions nécrotiques (voir § 2.3), un mécanisme de résistance général est induit, appelé SAR pour « résistance systémique acquise ». La SAR apparaı̂t de 30 min à plusieurs heures après l'infection initiale et entraı̂ne la résistance, lors d'une infection ultérieure, à un grand nombre de pathogènes potentiels aussi différents que des virus, des bactéries ou des champignons [5]. Elle se traduit parfois par la synthèse de phytoalexines, métabolites à activité antimicrobienne. Elle est accompagnée de l'apparition de protéines, dont la présence et l'activité sont corrélées avec le maintien de cette résistance : protéines PR (Pathogenesis Related), qui avaient été identifiées initialement lors de l'infection de plantes de tabac par le virus de la mosaı̈que du tabac (TMV), défensines, thionines... Le rôle précis des protéines PR dans l'acquisition de la résistance reste à démontrer, bien que nombre d'arguments y contribuent. Par exemple, la classe des PR-3 ou PR-Q est constituée de chitinases, alors qu'il n'existe pas de chitine dans le monde végétal, mais qu'on en trouve chez les pathogènes fongiques des végétaux et chez les insectes. Leur rôle direct dans la résistance générale est donc plausible. Une molécule importante pour la mise en place de la SAR est l'acide salicylique (SA), qu'il suffit d'administrer à la plante pour provoquer cette résistance. Cependant, l'acide salicylique n'est probablement pas le signal moléculaire qui est transporté de façon généralisée dans la plante depuis la lésion locale jusqu'au site d'infection.
2.2.2 La résistance systémique induite ou ISR
La résistance systémique induite est stimulée spécifiquement par des rhizobactéries de type Pseudomonas [6]. Le mécanisme est similaire à celui de la SAR : une première infection de la racine par Pseudomonas, qui n'est pas pathogène, stimule les capacités de défense de la plante à une infection ultérieure par un pathogène (bactérie et champignon) sur un organe qui peut être différent de la racine. Cependant, il semble que les voies d'induction de la SAR et de l'ISR soient différentes. En effet, l'ISR peut advenir dans des plantes dans lesquelles la synthèse de SA et par conséquent la SAR sont bloquées [7]. De plus, l'ISR n'est pas accompagnée de la synthèse des PR protéines. Les régulateurs de croissance végétale, jasmonates et éthylène, sont impliqués dans la mise en place de l'ISR. Notons que l'éthylène et les jasmonates sont synthétisés dans les plantes suite à une blessure. Il a été montré que chez Arabidopsis thaliana, l'activation simultanée de la SAR et de l'ISR conduisait à une résistance plus importante que chacune d'elle prise séparément [8].
2.3 Résistance spécifique induite
La résistance évoquée ici met en jeu un système de reconnaissance entre la plante et le micro-organisme. Bien que ce mécanisme conduise in fine à l'activation du phénomène de résistance systémique déjà évoqué, la spécificité de l'interaction est particulière.
La résistance spécifique aux agresseurs représente un phénomène naturel qui a été reconnu très tôt dans l'histoire de la génétique : dès 1905, Biffen [9] met en évidence, dans certaines variétés de blé, une résistance à la rouille jaune, Puccinia striiformis, qui présente un déterminisme génétique de type Mendélien, monogénique et dominant.
C'est l'étude du couple Melampsora lini, champignon agent de la rouille et du lin, qui a donné un support à H. Flor [10,11] pour l'énoncé du concept « gène pour gène », qui s'est depuis vérifié pour de nombreuses autres interactions et a été confirmé au niveau moléculaire. Ce concept est le suivant : un mécanisme de résistance est induit chez une plante hôte si et seulement si le produit d'un gène de résistance R reconnaı̂t le produit d'un gène d'avirulence Avr (Tableau 1).
Illustration de la base génétique d'une interaction simple entre un hôte diploı̈de et un pathovar
Génotype du pathogène | Génotype de l'hôte | |
R/− | r/r | |
Avr/− ou Avr | Incompatibilité ou résistance de la plante | Susceptibilité ou virulence du pathogène |
avr/avr ou avr | Susceptibilité ou virulence du pathogène | Susceptibilité ou virulence du pathogène |
Dans la plupart des cas, les symptômes d'une résistance s'observent localement autour du site d'infection : il apparaı̂t des lésions nécrotiques, limitées parfois à quelques cellules. Ce phénomène est qualifié de réaction d'hypersensibilité. Bien que la signification exacte de cette mort cellulaire ne soit pas totalement élucidée, il est admis que la nécrose circonscrit le pathogène, et entrave sa dissémination dans l'organisme infecté [12]. La réponse hypersensible est un processus actif de la plante qui n'est pas dû à l'attaque du pathogène lui-même, puisque des éliciteurs peuvent la provoquer. La réponse hypersensible s'apparente à la mort cellulaire programmée chez les animaux (voir Fig. 1).
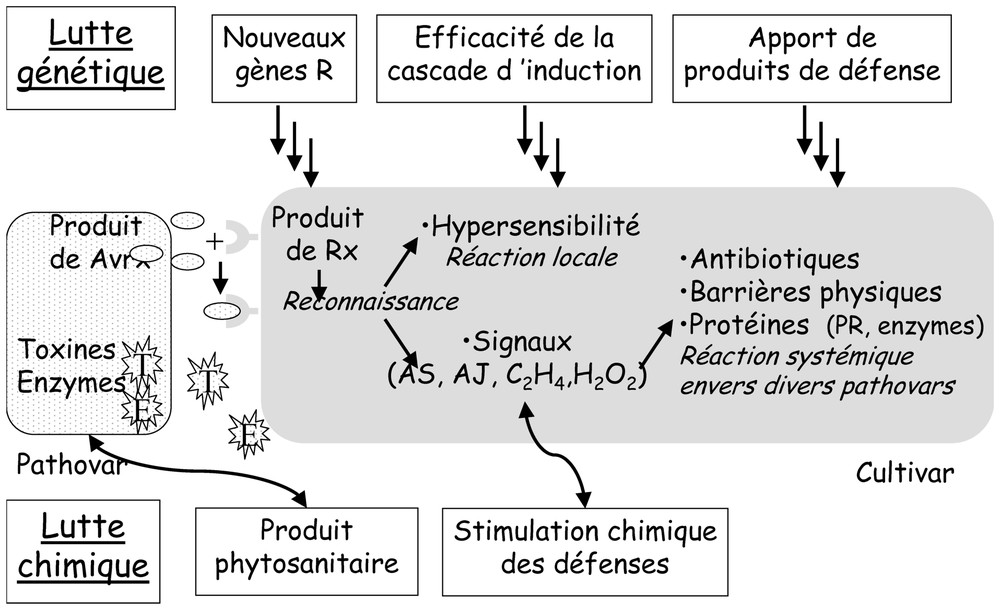
Place des luttes génétique et chimique dans la biologie de la plante.
2.3.1 Gènes d'avirulence chez les agresseurs
Plus d'une trentaine de gènes d'avirulence ont été isolés chez différents micro-organismes. D'une espèce à l'autre, les protéines Avr, qui sont en général des protéines hydrophiles solubles, ne présentent pas entre elles de similitudes de séquence.
La question de la reconnaissance entre les protéines R et les produits des gènes Avr n'a pas été complètement résolue. Le produit du gène Avr lui-même peut être reconnu par R, par exemple pour le couple Avr–Pto de Pseudomanas syringae et Pto de la tomate [13]. Dans quelques cas des molécules issues d'une hydrolyse spécifique pilotée par le produit du gène Avr sont reconnues [14]. Il est aussi envisagé que le produit du gène Avr s'associe à un ligand et que le complexe formé soit reconnu par le produit du gène R correspondant. L'hypothèse admise aujourd'hui dans le cas de certaines bactéries (Erwinia, Pseudomonas, Xanthomonas et Ralstonia) est celle de la sécrétion des protéines Avr directement dans la cellule de la plante hôte à l'aide d'un appareil de sécrétion similaire à celui de bactéries pathogènes animales Gram− [15].
Certaines protéines Avr possèdent des séquences consensus d'adressage vers des compartiments cellulaires particuliers : membrane cytoplasmique ou noyau cellulaire. La destruction de ces motifs d'adressage annihile la fonction d'avirulence [16].
Il peut sembler curieux qu'un pathogène possède un gène d'avirulence, limitant son propre développement. Si l'avirulence était leur seule finalité, ces gènes désavantageux auraient dû disparaı̂tre. La pérennité des gènes Avr réside dans leur ambivalence : il a en effet été démontré qu'ils avaient un effet positif sur la virulence chez des plantes hôtes qui ne possèdent pas le gène R correspondant. De plus, l'expression de gènes Avr chez des hôtes sensibles provoque des symptômes de la maladie identiques à ceux induits par l'agent infectieux [13].
2.3.2 Gènes de résistance des végétaux
Des gènes R ont été clonés à partir d'espèces végétales diverses, entraı̂nant une résistance vis-à-vis d'organismes aussi différents que des bactéries, des virus, des champignons, des nématodes et même des insectes. Les protéines codées par les gènes R peuvent se structurer en cinq classes [17,18], qui sont illustrées sur la Fig. 2.
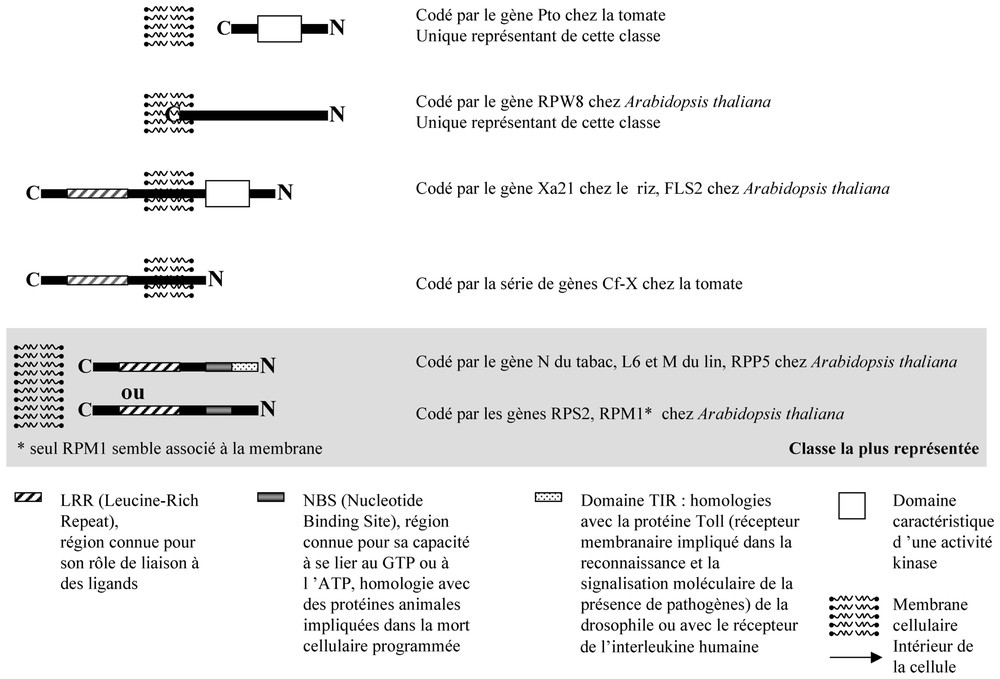
Structure des protéines R chez les végétaux.
La classe la plus représentée est constituée de protéines possédant un domaine de liaison à un nucléotide et un motif répété, riche en leucine. C'est la classe NBS–LRR (pour Nucleotide Binding Site–Leucine-Rich Repeats). Le domaine NBS présente des homologies avec des protéines impliquées dans la mort cellulaire chez d'autres eucaryotes. Le domaine LRR est connu comme étant le siège d'interactions peptide–ligand ; il pourrait être impliqué dans la reconnaissance de l'agresseur, grâce à une liaison spécifique avec le produit du gène Avr.
Les expérimentations menées sur les gènes de résistance L à la rouille du lin restent une référence en matière d'étude du siège de la spécificité. Il a en effet été montré que la région LRR était nécessaire mais non suffisante à la reconnaissance des protéines d'avirulence, la région N-terminale des protéines L étant aussi importante dans cette fonction. Notons qu'un nouveau profil de spécificité de résistance a pu être créé par réarrangement des gènes codants pour ces régions [19].
Deux classes de protéine comportent un domaine trans-membranaire, le domaine LRR étant exposé à l'extérieur de la cellule. Cette architecture pourrait constituer un système de surveillance externe des agresseurs.
2.3.3 Co-évolution des gènes R et Avr
Un modèle intuitif sous-tendant la co-évolution des gènes d'avirulence des micro-organismes et des gènes de résistance correspondants chez les plantes hôtes est celui de la course à l'armement, qui devrait être matérialisée par une escalade dans le nombre des gènes impliqués et donc par une signature moléculaire [20].
L'analyse des génomes végétaux révèle que les gènes codants pour des motifs conservés NBS–LRR sont situés sur les mêmes régions chromosomiques, constituant des « méga-regroupements » [21]. Chez Arabidopsis thaliana, cette classe de gènes comporterait environ 165 membres [22]. Il est probable que ce schéma d'organisation reflète une série de duplications et de recombinaisons non homologues, dont les produits ont été soumis à une sélection positive pour la reconnaissance d'une souche de pathogène. Notons que les gènes NBS–LRR possédant un motif TIR (voir Fig. 2) semblent totalement absents chez les céréales et peut-être chez les monocotylédones [23]. La diversité des gènes observée serait apparue postérieurement à la divergence entre monocotylédones et dicotylédones, il y a environ 100 millions d'années.
Il a aussi été observé que les séquences de gènes R orthologues correspondant à un même allèle au sein d'espèces différentes se ressemblaient plus entre elles que des séquences de gènes R paralogues correspondant à des séquences dupliquées au sein d'un même génome. Ce fait a été interprété comme la trace d'un phénomène de sélection divergente, créant de nouvelles spécificités pour la reconnaissance de produits Avr, parallèlement à la nécessité de conserver les reconnaissances Avr acquises [24]. La réponse évolutive des pathogènes vis-à-vis de la défense des plantes est signalée par la mise en place d'enzymes de détoxication. Par exemple, Phytophtora sojae synthétise des protéines, inhibiteur d'une béta-1,3-endoglucanase de son hôte, le soja. Chaque inhibiteur est spécifique d'une isoforme de l'enzyme. D'autres exemples impliquant des couples enzymes/inhibiteurs ont été rassemblés, confirmant l'hypothèse d'une sélection concertée entre un pathogène et son hôte [20].
2.4 Signaux transmis
Il est prouvé que des gènes autres que les gènes R et Avr sont nécessaires à l'expression de la résistance spécifique. Nombre de mutations engendrent la suppression totale ou partielle de l'expression de la résistances en présence du couple de gènes R–Avr. Parallèlement, ces mutations entraı̂nent une susceptibilité accrue à d'autres pathogènes. Cette observation suggère que des signaux communs existent pour le déclenchement de la résistance spécifique et celui de la résistance systémique acquise [25].
Récemment, il a été prouvé chez Arabidopsis thaliana que les mécanismes précoces de défense déclenchés par un éliciteur mettaient en jeu l'activité consécutive de trois MAP (Mitogen-Activated Protein) kinases [26]. La famille des MAP kinase est impliquée dans la traduction de stimulus extracellulaires en signaux intracellulaires par une série de phosphorylations aboutissant in fine à l'activation et/ou à la répression de facteurs de transcription [27]. L'activation des MAP kinases est similaire à ce qui a été observé chez la drosophile dans la régulation des gènes à effet anti-microbiens [28]. Par ailleurs, d'autres voies de signalisation, spécifiques de telle ou telle classe de gènes ont été mises en évidence et montrent bien que les chemins pour engager la réaction de défense de la plante sont multiples et complexes : EDS1 et PAD4 pour les gènes de type NBS-LRR-TIR, NDR1 pour la plupart des autres gènes NBS-LRR [29]...
Notons que la perception d'une agression biotique peut emprunter les mêmes voies de signalisation que la perception d'agressions physiques, par exemple des stress osmotiques ou salins ou une simple blessure.
D'autres facteurs interviennent dans la signalisation de l'agression, quelques secondes à quelques minutes seulement après l'attaque. Il s'agit de flux ioniques trans-membranaires : influx de Ca2+ et de H+, efflux de K+ et de Cl−. L'application de chélateurs du calcium abolit l'activation des défenses. La mort cellulaire programmée (ou PCD) est aussi corrélée à un accroissement de la production de formes très réactives de l'oxygène telles que H2O2. Il a été montré que H2O2 chez Arabidopsis thaliana activait l'enchaı̂nement de phosphorilation des kinases, conduisant à la mise en place des réactions de défense [30]. La molécule a aussi un effet toxique direct sur l'agent pathogène. L'oxyde nitrique pourrait avoir un rôle dans l'induction de la PCD végétale, comme chez les animaux [31].
2.5 Résistance croisée aux virus
Le mécanisme de résistance croisée a été observé lors d'infections virales. Dès les années 1920, il avait été constaté qu'une plante infectée par une souche virale atténuée était résistante à l'attaque ultérieure par une souche virulente du même virus ou d'un virus proche. Le mécanisme qui sous-tend ce phénomène commence à être élucidé [32].
Une avancée des connaissances a été faite grâce à l'étude approfondie de plantes transgéniques, chez lesquelles un phénomène de gene silencing a été observé : certaines lignées de plantes transgéniques présentent un gène étranger fortement exprimé, dont l'expression tend à disparaı̂tre au fil des générations. Il a maintenant été prouvé que ce phénomène est dû à la formation d'ARN double brin, probablement synthétisé à la suite de remaniements du transgène lors de l'événement de transformation. Il suffit d'un ARN double brin de 21 nucléotides associé à un complexe protéique pour diriger la dégradation de l'ARN messager ou de l'ARN viral homologue.
Ce mécanisme serait identique à celui de la protection croisée. Le signal transmis aux parties distales de la plante, qui lui conférera une résistance totale ou partielle lors d'une infection ultérieure, n'est pas encore clairement identifié.
3 Apport de la génétique dans les méthodes de lutte
Les plantes cultivées, par les modifications introduites au cours de leur domestication, comme l'hypertrophie de certains organes, par l'étendue des surfaces de culture et souvent leur uniformité variétale, ainsi que par leur introduction dans des biotopes nouveaux, présentent une fragilité particulière aux attaques de certains parasites, qui peuvent entraı̂ner des pertes considérables.
Des résistances génétiques de différents types existent dans des écotypes sauvages ou des espèces apparentées. Elles sont surtout présentes dans les variétés récentes, à la suite des travaux d'amélioration génétique, mais relativement moins dans les variétés cultivées anciennes, auxquelles les pathogènes se sont adaptées [33]. En effet, de nombreuses recherches ont été menées afin d'introduire, dans les variétés cultivées, des résistances à partir de sources variées (Tableau 2). Ces introductions impliquent d'abord des croisements avec des plantes d'une autre origine. Il peut s'agir de la même espèce ou d'une espèce apparentée, ce qui se réalise assez facilement, voire spontanément, dans le règne végétal. On sait aussi contourner l'incompatibilité sexuelle entre deux espèces en usant d'artifices comme la culture d'embryons, la fécondation in vitro ou même l'hybridation somatique par fusion de protoplastes.
Quelques exemples de résistances génétiques introduites par hybridation
Espèce | Caractère |
Ail | Virus de la jaunisse nanifiante de l'oignon |
Betterave | Rhizomanie, nématodes, cercosporiose, rhizoctone brun, Aphanomyces |
Blé | Piétin-verse, rouilles (jaune, brune, noire), septoriose, fusariose, virus de la jaunisse nanifiante de l'orge, oı̈dium |
Caféier | Trachéomycose |
Canne à sucre | Charbon, échaudement |
Carotte | Alternariose |
Colza | Phoma |
Courgette | Oı̈dium, virus de la mosaı̈que jaune du zucchini, virus de la mosaı̈que du concombre, virus de la mosaı̈que 2 de la pastèque |
Dactyle | Rouilles |
Fétuque élevée | Rouilles |
Féverole | Anthracnose |
Haricot | Anthracnose, virus I, tache bactériennes |
Igname | Anthracnose |
Laitue | Oı̈dium, pucerons, virus de la mosaı̈que de la laitue |
Lin | Fusariose, brûlure |
Luzerne | Verticiliose, anthracnose, nématodes des tiges |
Melon | Oı̈dium, fusariose, pucerons, virus de la mosaı̈que jaune du zucchini, virus nécrotique du melon |
Noisetier | Anthracnose |
Orge | Mosaı̈que, jaunisse nanisante |
Palmier à huile | Fusariose |
Peuplier | Brûlure des pousses, rouille, tache foliaire |
Pois | Anthracnose, oı̈dium |
Piment | Virus de la striure du tabac, virus de la marbrure bénigne du piment, virus de la mosaı̈que du concombre, virus de la mosaı̈que du tabac |
Pyracantha | Tavelure, feu bactérien |
Ray-grass | Rouille |
Tomate | Verticiliose, fusariose, nématodes, virus de la mosaı̈que du tabac, virus de la Mosaı̈que bronzée de la tomate, mildiou, flétrissement bactérien, oı̈dium, chancre bactérien, racines liégeuses |
Tournesol | Mildiou, Phomopsis, Sclerotinia |
L'hybridation interspécifique tient une place importante dans l'introduction de ces résistances. Dans le cas du blé, il faut citer les croisements avec Aegilops ventricosa, réalisés dès 1957 par Simonet et qui ont conduit à des variétés présentant une très bonne résistance au piétin verse, grâce au gène Pch1 [34], mais également un bon niveau de résistance à l'oı̈dium, aux rouilles, à la septoriose et à des nématodes à kystes. En fait, les résistances aux rouilles (conférées par les gènes Yr17, Lr37, et Sr38, vis-à-vis respectivement des rouilles jaune, brune et noire) sont physiquement liées sur un fragment chromosomique provenant de cette espèce. Il est présent dans plus de 40 % des variétés de blé inscrites en France depuis 1996. Une situation analogue résulte du transfert du bras court du chromosome 1 de seigle sur le chromosome 1 du génome B du blé (gènes Yr9, Lr26 et Sr3). D'autres gènes de résistance proviennent d'Agropyron elongatum comme Sr26.
Dans le cas de la tomate, un grand nombre de croisements interspécifiques ont été réalisés pour introduire des résistances à divers types de pathogènes : virus, bactéries, champignons. On estime que de ce fait, près de 10 % du génome des nombreuses variétés de tomate cultivées proviennent d'espèces apparentées, comme L. hirsutum, L. peruvianum, L. pimpinellifolium [35].
La sélection pour la résistance aux maladies est assez lourde et délicate à mettre en œuvre et, suivant l'espèce, la résistance à telle ou telle maladie peut devenir, pour des raisons « économiques » à court terme, un objectif prioritaire ou non. Chez la laitue, les résistances au bremia (mildiou de la laitue) sont très vite surmontées, à tel point que plus de 13 gènes de résistance ont été utilisés successivement dans les variétés. La résistance à une nouvelle race fait l'objet d'une recherche incessante, car elle constitue une nécessité commerciale. Cependant, la réponse à court terme (introduction d'un nouveau gène) prime sur la stratégie à long terme (introduction de plusieurs déterminants à la fois). La seule façon économique et rapide d'introduire plusieurs gènes de résistance à la fois résiderait, comme nous le verrons plus loin, dans l'utilisation d'outils moléculaires permettant de suivre l'introduction de ces gènes sans avoir recours à des tests d'infection artificielle. En revanche, chez la tomate, la priorité de sélection actuelle porte sur des caractères qualitatifs, d'aspect et de tenue et, en conséquence, la création variétale s'intéresse peu aux maladies.
La durabilité des résistances génétiques à des pathogènes connus est sans doute la question primordiale. Facilité de sélection pour un seul gène de résistance est souvent associée à facilité de contournement par le pathogène. Des exemples de résistances à un déterminisme génétique simples, mais durables, existent néanmoins [36]. Parmi les résistances durables chez les céréales, citons les résistances aux rouilles et au piétin-verse issues d'A. ventricosa, la résistance au piétin portée par le chromosome 1A de la variété Cappelle Desprez, les résistances contrôlées par les gènes Lr34, Lr46, Yr18. Le gène récessif mlo confère la résistance au mildiou chez l'orge. Des cas de résistance durable vis-à-vis de virus existent chez la pomme de terre (cumul des gènes Nb et Nx pour la résistance au virus X de la pomme de terre ou PVX), ou chez la tomate (le gène Tm-22 de résistance au TMV). Il y a d'autres exemples plus connus dans le domaine des insectes parasites, comme la résistance aux pucerons chez certaines variétés de pommiers ou la résistance au phylloxera des porte-greffes issus d'espèces d'origine américaine. La notion de durabilité est, bien entendu, relative. Dans le cas du haricot, par exemple, la résistance au virus de la mosaı̈que commune conférée par le gène d'hypersensibilité I dure depuis plus de 50 ans. Chez cette espèce également, alors que la résistance à l'anthracnose, due au gène Co2, est stable dans nos régions, les variétés européennes, qui le possèdent à peu près toutes, sont immédiatement attaquées en Amérique latine.
4 Aspects prospectifs apportés par la connaissance en génétique moléculaire
4.1 Le génie génétique
La connaissance des séquences des gènes et les techniques de transformation permettent de s'assigner un double objectif : aboutir à une résistance durable vis-à-vis d'un large spectre de pathogènes.
Quand un gène de résistance a été isolé chez une espèce, il est possible, par génie génétique, de le transférer dans le génome d'une autre espèce de manière plus précise que par hybridation. En effet, ce gène, et seulement celui-ci, pourra être transmis, et non plus un fragment de chromosome d'une taille indéfinie, porteur de plusieurs centaines, voire plusieurs milliers, d'autres gènes, souvent associés à des caractères indésirables.
Cependant, comme nous l'avons vu, une résistance s'appuyant sur un seul déterminant, en particulier dans le cas d'une résistance par hypersensibilité, est souvent contournée. Néanmoins, on peut imaginer construire plus rapidement que par les méthodes traditionnelles des lignées d'un même cultivar possédant chacune un gène de résistance distinct. La culture de variétés, « multi-lignées » ou « multi-hybrides-F1 », présentant différents gènes ou combinaisons de gènes de résistance, éventuellement à l'origine au même locus, devient plus réaliste. De plus, cette stratégie peut s'appliquer aussi à des espèces à reproduction végétative.
On peut concevoir une meilleure gestion de ces gènes de résistance, car ils seront facilement repérables, par des tests moléculaires, parmi les variétés, dans l'espace et dans le temps en fonction des évolutions des pathogènes. La connaissance moléculaire, associée ou non à un titre de propriété industrielle si la variété est transgénique, permet une traçabilité. Ceci n'est pas le cas des variétés classiques, pour lesquelles les résistances ne sont pas toujours définies en fonction de leur origine.
Le génie génétique permet de construire de nouvelles résistances.
Un premier exemple concerne l'activation de certaines voies de signalisation, comme par exemple l'expression constitutive du facteur de transcription NPR1 chez Arabidopsis, qui active la voie de l'acide salicylique. Les plantes transformées sont alors tolérantes à plusieurs pathogènes [37]. Cette approche présente en général un coût physiologique, à la fois en termes de réduction de vigueur de la plante et de susceptibilité vis-à-vis d'autres pathogènes. Il s'agira donc d'un compromis, en privilégiant, bien entendu, la lutte contre les agresseurs les plus importants.
Une autre possibilité est la surproduction de protéines antifongiques ou bactériennes. C'est le cas, par exemple, des chitinases [38], qui interviennent pour hydrolyser la paroi des champignons, ou des enzymes de biosynthèse de terpènes anti-bactériens. Plus généralement, il s'agira de faire produire à la plante des métabolites antagonistes des pathogènes.
Un autre exemple consiste à faire produire un éliciteur par les cellules de la plante en réponse à une attaque quelconque. Il est nécessaire, dans ce cas, de disposer d'un promoteur inductible par une infection, quelle qu'elle soit, qui va contrôler la production par la cellule végétale de l'éliciteur, qu'elle reconnaı̂tra grâce à la présence du gène R correspondant, ce qui aura pour conséquence la stimulation des réactions de défense. Cette stratégie a été appliquée avec succès au tabac [39] et à la tomate [40] et permet même dans ce dernier cas d'induire une résistance à des virus.
La stratégie la plus prometteuse consiste sans doute à priver le parasite de facteurs essentiels à sa pathogénicité. Par exemple, le Sclerotinia sclerotiorum, qui est capable d'attaquer une centaine d'espèces appartenant à 64 familles botaniques différentes, produit de l'acide oxalique, qui est indispensable à son pouvoir pathogène en agissant comme cofacteur dans l'hydrolyse des parois végétales et en affaiblissant les défenses. Une plante transgénique qui produit une oxalate oxydase en présence du champignon va dégrader cet acide en CO2 et H2O2, cette dernière molécule agissant comme activateur des systèmes de défense [41].
Il est clair que de la connaissance des gènes du pathogène comme de l'hôte qui interviennent dans leur relation pourront émerger d'autres possibilités, peut-être plus générales, s'appuyant sur cette stratégie.
4.2 Recherche de locus à effet quantitatifs (ou QTL) et sélection assistée par marqueurs (SAM)
Les gènes R interviennent dans la reconnaissance du pathogène, étape préalable à la transmission du signal qui déclenchera, par diverses voies qui s'imbriquent, la résistance locale et à la résistance systémique. Or, le signal transmis à la plante, l'expression de la résistance systémique et probablement la résistance locale font intervenir de nombreux autres gènes. Il est donc justifié de traiter la résistance à un pathogène comme un caractère complexe, en définitive déterminé par de nombreux gènes. Il est possible, grâce à l'exploitation de la génétique combinée à la biologie moléculaire, de localiser sur les chromosomes d'une espèce des locus à effet quantitatifs ou QTL (Quantitative Trait Loci). Ainsi, par exemple, des QTL de résistance au mildiou ont été isolés chez Arabidopsis thaliana, et à l'anthracnose chez le haricot [42,43] Certains de ces QTL, comme le gène RPW8, intervenant dans la résistance au mildiou chez Arabidopsis, sont impliqués dans une résistance à un large spectre d'espèces différentes de pathogènes [42,44]. Cette observation confirme le fait que la résistance dans sa globalité fait intervenir plusieurs gènes. La connaissance de la structure des gènes R de type NBS–LRR et la totalité de la séquence du génome de l'espèce Arabidopsis thaliana ont permis de repérer 200 à 300 gènes candidats impliqués dans la résistance aux pathogènes, soit 1 à 2 % de la capacité codante du génome [21].
Est appelé marqueur un caractère aisément identifiable et d'autant plus lié au caractère recherché qu'il est déterminé par un gène physiquement proche sur le chromosome. Un marqueur M doit être polymorphe au sein d'une population, avec une forme allélique qui sera associée à la forme allélique recherchée du caractère. Le caractère et M seront donc associés dans la descendance d'un croisement.
Il y a peu, les marqueurs pouvaient être des caractères visibles, comme la couleur ou la forme d'un organe, par exemple. La sélection assistée par marqueurs a gagné en puissance avec les marqueurs moléculaires, en particuliers ceux qui font appel à des séquences nucléotidiques. La séquence d'un marqueur est repérable grâce aux techniques de la biologie moléculaire, qui mettent en jeu, soit l'hybridation moléculaire avec une séquence connue puis les diverses méthodes de synthèse d'ADN in vitro, suivies de l'analyse des fragments issus de cette synthèse, soit la mise en évidence de variations discrètes par spectrométrie de masse...
Nous avons vu plus haut que les gènes de résistance R étaient conservés dans leur structure. Il est alors possible de repérer des « gènes candidats » pour la résistance à des pathogènes en utilisant des molécules marqueurs synthétiques correspondant à des parties hautement conservées de la séquence codante des gènes R. Ainsi, des gènes candidats ont été isolés chez le soja, en utilisant des séquences issues de gènes R de tabac, du lin et d'Arabidopsis [45]. Ce premier crible va permettre de caractériser plus finement un nombre restreint de gènes et, peut-être, d'identifier de nouveaux gènes jouant un rôle dans la résistance.
Ces marqueurs permettent d'analyser une grande quantité d'individus de manière précoce dans leur développement, et de suivre également des gènes récessifs. Une telle méthode est particulièrement intéressante pour ce qui concerne la sélection de cultivars résistants à des pathogènes, car il devient possible de s'affranchir momentanément de tests à grande échelle, ce qui est avantageux d'un point de vue sanitaire et pour la fiabilité des expérimentations.
5 Conclusion
La résistance génétique représente une valeur économique importante. La sélection de variétés végétales résistantes aux pathogènes a fait l'objet de travaux incessants au cours du siècle dernier, avec des succès et des échecs. Les mécanismes de résistance, particulièrement complexes, commencent seulement à être compris. Ils conduisent les chercheurs à envisager diverses stratégies nouvelles pour améliorer les cultivars quant à leur comportement face à leur cortège de pathogènes. La sélection assistée par marqueurs, permet d'accélérer l'obtention par croisement de cultivars résistants et de caractériser plus précisément les spécificités de ces résistances. Le génie génétique peut agir à différents niveaux : cumul des gènes R de résistance/reconnaissance, amplification des réactions de défense, suppression de fonctions indispensables au pathogène, etc. L'utilisation du génie génétique, pourtant bien encadrée par des instances de contrôle indépendantes, est dévalorisée dans l'esprit du public, au sein d'un scepticisme plus large englobant les biotechnologies dans leur ensemble. Pourtant, ces dernières permettent de proposer des réponses à la demande d'une réduction des intrants dans les pratiques agronomiques et d'une gestion durable de l'environnement. La permanence de la résistance reste une inconnue qu'il convient d'intégrer dans les programmes de sélection de variétés. La connaissance moléculaire des déterminants de résistance ou des marqueurs qui leur sont liés ouvre la possibilité d'une réelle gestion à long terme de ces résistances, depuis le sélectionneur jusqu'au territoire de culture.
Remerciements
Les auteurs remercient J. Aubert, A. Basley, R. Berthomé, M. Bougot, D. Levy et H. Mc Khann pour leur aide au cours de la rédaction du manuscrit.