1 Introduction
One of the most fundamental challenges in ecology and conservation biology is the understanding of the spatial distribution of animals and long-term fluctuations in their density [1]. The spatial distribution often reflects the behaviour of individuals (i.e. searching behaviour of a suitable habitat) within the population as well as demographic changes. Thus, a diverse array of habitat types can occur even within a small area, and animals use this diversity in complex ways. The individual's ‘choice’ of particular habitat attributes presumably relates to advantages and disadvantages associated with each alternative [2].
In coral ecosystem, most marine organisms (e.g., invertebrates, fish) have stage-structured life histories with two distinct stages, a relatively sedentary benthic stage (usually juveniles and adults) and a pelagic larval stage capable of long-distance dispersal [3]. Having dispersed, a larva has the choice of where to settle, and the behaviour involved in the process of settlement is usually described as habitat selection [4]. Marine larvae often show marked selectivity in the habitats they choose based on the presence of specific benthic substrata and/or the presence of conspecifics or other species [5,6]. The literature suggests that these initial choices at settlement play a fundamental role in determining the number and spatial distribution of their later life stages [7]. However, patterns in habitat use established at settlement may be extensively modified at the local scale if marine organisms exhibit movement after settlement and/or are subject to differential mortality related to habitat type [8].
As an individual grows, its morphology and behaviour changes. For some species, this means that they can no longer obtain the resources they require for life processes and they are faced with one of two choices; they can either die or move to a location that supports their life processes (reproduction, growth and survival). Ontogenetic shifts have been documented for many diurnal marine invertebrates and fishes with respect to diet and resources, and microhabitat [8]. But, little attention had been paid to the spatial patterns and dynamics of nocturnal organisms, in spite of their important role as key-species in marine communities [9,10]. Consequently, we decided to conduct a study at Moorea Island (French Polynesia) to investigate how widespread ontogenetic shifts in the habitat use are in nocturnal coral reef fish between recently settled juvenile and adult stages. Our study will allow us to compare the ontogenetic shift patterns of nocturnal fish to those observed in diurnal fish.
2 Material and methods
The present study was conducted in the north coast of Moorea lagoon (Matautia sector), French Polynesia (, ). This location was characterised by 12 distinct reef zones based on depth, wave exposure, and substratum composition (Fig. 1). Counting of recently settled juveniles was conducted in April, May, and June 2001. Counting of adults was conducted in May 2001. Nocturnal underwater visual counting of fish was carried out along five transects (bands perpendicular to the coast and continuous from the coast to the reef crest), 1 km long and 1 m wide. Each of the five transects was counted once every month with a rhythm of one transect per night (8 to 12 pm) every second night (the ten days of counting were centred on new moon of each sampling month). The abundance of each species was recorded for each shelter use of each reef zone of each transect. The different categories of shelter were sand, coral rubble, coral slab, macro-algae and coral (with distinction between the coral genus, the coral form, and its state – dead or live).

(A) Schematic view of the position of different reef zones and of five transects sampled in the north Coast of Moorea Island (Matautia sector). (B) Schematic view of cross-reef profile showing the relative position of reef zones with the mean width and the standard error calculated on the five transects. Rz1: Front barrier reef (made up of only coral rubble–reef zone of 62 m wide), Rz2: mid-barrier reef with high living coral cover (>30%), Rz3: mid-barrier reef with low living coral cover (<30%), Rz4: deep-barrier reef (low-living coral cover and depth >2 m), Rz5: Mid-barrier reef with high dead coral cover (>80%), Rz6: Huge Porites rus colony (31 m wide, 80 m long, and 2 m high), Rz7: deep lagoonal slope on barrier reef, Rz8: Channel, Rz9: deep lagoonal slope on fringing reef, Rz10: mid-fringing reef with high living coral cover (>30%), Rz11: micro-atoll area, Rz12: Sand area. MSL: Mean Sea Level.
3 Results and discussion
A total of 2709 recently settled juveniles belonging to 21 species and 2784 adults belonging to 24 species were recorded in the Matautia sector. Seventeen species have been recorded at both juvenile and adult stages (Table 1). The comparison between spatial distributions of recently settled juveniles and adults demonstrated that nine out of the 17 species showed similar patterns of habitat use at both life stages (see Table 1, p-value of ANOVA tests >0.05). Eight species showed then ontogenetic shifts in habitat use (p-value <0.05). For Apogon novemfasciatus, Cheiliodopterus quiquelineatus, Mulloidichthys sp. and Neoniphon sammara, the ontogenetic shifts resulted in increasing the number of reef zones used during the adult stage (Table 1). Mulloidichthys sp. juveniles settled on fringing reef (total wide of life area in Moorea lagoon = 453 m) and as adults they lived on fringing and barrier reef (total width of life area = 528 m). For Apogon angustatus and Platybelone sp., the ontogenetic shifts resulted in decreasing of the number of reef zones adults used compared to recently settled juveniles (Table 1). A. angustatus juveniles settled on eight reef zones (total width of life area = 590 m) and as adults lived on four reef zones (total width of life area = 431 m). For Apogon coccineus, the ontogenetic shifts resulted in using of different reef zones (Table 1). Lastly, for Sargocentron microstoma, the ontogenetic shifts resulted in using the same reef zones between juveniles and adults but with relative densities different (Table 1). These ontogenetic shifts at reef zone scale were accompanied by shifts at shelter scale (i.e. between coral morphologies) for three species (A. angustatus, A. coccineus, and N. sammara). A. angustatus juveniles used living coral colonies of Porites sp. in massive shape and as adults they used living coral colonies of Porites sp. in massive and branched shape (Fig. 2).
In the Matautia sector, 17 nocturnal coral reef fish species were recorded both at recently settled juvenile and adult stages by underwater visual counting from April to June 2001. The data correspond to densities (number of fish per 1000 m2) of each species at two life stages on the reef zones. Statistical tests (two factors – ANOVA: life stages, * reef zones) were conducted on the relative density of each species per reef zone to highlight ontogenetic shifts in habitat use between recently settled juveniles and adults (if the p-value <0.05, we decide on an ontogenetic shift). We highlighted five distinct patterns: (1) increase in the number of reef zones used during the adult stage (↗); (2) decrease in the number of reef zones adults used compared to recently settled juveniles (↘); (3) use of different habitat types (); (4) use of the same habitat types, but with different relative densities between the two life stages (=≠=); and (5) no change in habitat use (===)
Ontogenetic stages | RZ1 | RZ2 | RZ3 | RZ4 | RZ5 | RZ6 | RZ7 | RZ8 | RZ9 | RZ10 | RZ11 | RZ12 | Ontogenetic shift pattern | |
APOGONIDAE | ||||||||||||||
Apogon angustatus | Juvenile | 2.6 | 5.4 | 9.1 | 0.4 | 1.8 | 0.5 | 0.7 | 4.6 | p<0.001 | ||||
Adult | 2.5 | 4.1 | 11.2 | 9.1 | ↘ | |||||||||
Apogon coccineus | Juvenile | 0.6 | 3.3 | 0.4 | 1.6 | 5.1 | p=0.04 | |||||||
Adult | 2.9 | 2.1 | 4.6 | 1.5 | 1.4 | |||||||||
Apogon exostigma | Juvenile | 2.9 | 2.1 | p=0.53 | ||||||||||
Adult | 3.4 | 5.0 | 23.3 | 16.6 | === | |||||||||
Apogon fraenatus | Juvenile | 1.5 | 0.6 | p=0.06 | ||||||||||
Adult | 2.1 | 1.9 | === | |||||||||||
Apogon fuscus | Juvenile | 9.6 | 11.4 | 1.3 | 15.5 | 11.3 | 58.2 | 12.2 | p=0.42 | |||||
Adult | 4.8 | 6.8 | 15.8 | 8.3 | 63.5 | 22.4 | 18.3 | 7.9 | === | |||||
Apogon novemfasciatus | Juvenile | 9.9 | 12.1 | 3.6 | 1.7 | 3.2 | 1.2 | p=0.02 | ||||||
Adult | 12.1 | 8.1 | 23.0 | 11.8 | 13.5 | 12.2 | 12.7 | 6.4 | 5.0 | ↗ | ||||
Cheiliodopterus quiquelineatus | Juvenile | 7.9 | 2.3 | 6.0 | p=0.01 | |||||||||
Adult | 1.0 | 1.2 | 2.8 | 2.4 | 0.7 | ↗ | ||||||||
MURAENIDAE | ||||||||||||||
Gymnothorax javanicus | Juvenile | 0.9 | 0.2 | 0.2 | 0.3 | 0.2 | p=0.80 | |||||||
Adult | 8.1 | 2.5 | 1.7 | 2.7 | 7.1 | === | ||||||||
MULLIDAE | ||||||||||||||
Mulloidichthys sp. | Juvenile | 27.2 | 4.4 | 12.4 | 22.4 | p=0.006 | ||||||||
Adult | 18.1 | 6.8 | 25.3 | 51.1 | 17.3 | 25.7 | ↗ | |||||||
HOLOCENTRIDAE | ||||||||||||||
Myripristis pralinia | Juvenile | 2.7 | 12.1 | 2.3 | 45.0 | 29.0 | p=0.61 | |||||||
Adult | 25.8 | 33.5 | 7.8 | 42.9 | 8.1 | 16.7 | === | |||||||
Myripristis violacea | Juvenile | 35.3 | 4.7 | 2.6 | p=0.90 | |||||||||
Adult | 23.7 | 23.7 | === | |||||||||||
Neoniphon operculatis | Juvenile | 2.1 | 0.3 | 14.0 | p=0.13 | |||||||||
Adult | 10.2 | 6.3 | 5.5 | 11.3 | === | |||||||||
Neoniphon sammara | Juvenile | 1.1 | 0.6 | 1.2 | 3.2 | p=0.01 | ||||||||
Adult | 3.0 | 7.4 | 1.7 | 1.5 | 0.9 | 1.9 | ↗ | |||||||
Sargocentron diadema | Juvenile | 1.7 | 9.2 | 0.8 | 18.0 | 7.0 | 15.3 | 6.7 | 11.6 | 7.4 | p=0.16 | |||
Adult | 7.1 | 1.7 | 8.3 | 5.3 | 7.2 | 11.6 | 5.3 | 5.6 | 9.5 | 4.8 | === | |||
Sargocentron microstoma | Juvenile | 4.6 | 20.2 | 51.5 | 40.4 | 31.6 | 3.6 | 34.4 | 26.1 | 22.4 | 3.3 | p=0.03 | ||
Adult | 33.4 | 35.4 | 35.7 | 21.0 | 19.2 | 18.0 | 15.7 | 5.1 | 1.6 | 2.6 | =≠= | |||
BELONIDAE | ||||||||||||||
Platybelone sp. | Juvenile | 5.8 | 8.9 | 11.2 | 2.2 | 5.9 | 6.3 | 1.2 | 3.8 | p=0.005 | ||||
Adult | 5.3 | 2.5 | 1.7 | 5.4 | 0.9 | ↘ | ||||||||
SCORPAENIDAE | ||||||||||||||
Scorpaenodes guamensis | Juvenile | 1.1 | 2.5 | 0.4 | 0.8 | 0.8 | 0.3 | 0.7 | p=0.15 | |||||
Adult | 2.0 | 6.6 | 15.1 | 5.7 | 1.9 | === |
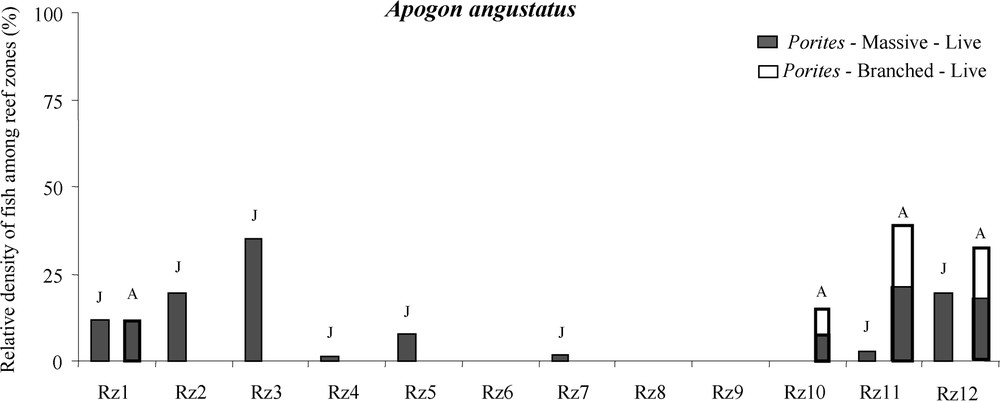
Patterns of spatial distribution and relative density of Apogon angustatus sampled on Matautia sector to document ontogenetic shifts in habitat use [comparison of reef zone and shelter use by recently settled juveniles (J) vs. adults (A)]. The shelters were characterized by the coral genus (Porites), the coral form (branched or massive), and its state (live or dead).
During the early post-settlement period, fish juveniles suffer the greatest mortality rate of the benthic portion of the life cycle, mostly via predation [7]. Recently settled juveniles must find habitat with sufficient refuge, yet be able to forage efficiently in order to grow to a size that is less vulnerable to predation [7]. The present study allowed us to highlight five distinct patterns in habitat use between recently settled juveniles and adults. One pattern reinforced spatial distributions establish at settlement: no change in habitat use (nine species). The four others disrupted them: an increase in the number of habitats used during the adult stage (four species); a decrease in the number of habitats adults used compared to recently settled juveniles (two species); the use of different habitat types (one species); and the use of same habitat types between juveniles and adults but with relative densities different (one species). Habitat utilisation is a trade-off between growth rate and predation risk [11]. The reinforcement of settlement signal would occur where growth and/or survival of recently settled juveniles are better in habitats attracting high settlement [12]. However, where growth and/or survival are inversely or unrelated to settlement patterns, these variables may be sufficiently important to cause disruption of the initial pattern [13]. Higher survivorship and/or growth in habitats receiving lower settlement may partially compensate for the lower input, and then could explain the disruption of the settlement signal for eight out of the 17 nocturnal species recorded at Moorea Island.
The five ontogenetic patterns that we highlighted in nocturnal fish have already been shown in diurnal fish [8]. A wide variety of diurnal fish would also maximize the trade-offs between growth rate and predation risk by undergoing size-specific ontogenetic niche shifts. In a typical example (i.e., increase in the number of habitats used during the adult stage), juvenile Pseudolabrus celidotus (Labridae) are closely associated with macroalgal shelter, but their shelter-dependence appears to decrease with growth. At the same time, they switch from feeding on micro-crustaceans present in macroalgae to those associated with bare rock-coralline turf areas, where the adults are found [14]. Ontogenetic habitat shifts can thus facilitate the partitioning of food resources between conspecifics or among similar species [8]. On the other hand, one ontogenetic pattern commonly observed in diurnal fish has not been illustrated in our data: use of nursery areas by recently settled juveniles followed by extensive movement to an adult habitat [15,16]. Indeed, no nursery areas (i.e. reef zones with high density of recently settled juveniles and low density of adults) could be highlighted in nocturnal fish communities at Moorea Island. Similarly, some diurnal species show ontogenetic changes in aggregation behaviour when the coasts and benefits associated with group-living depend on individual size (shoaling behaviour at settlement and individualized behaviour at adult phases) [17]. No such aggregation patterns have been highlighted in our data. However, we highlighted that social interactions could drive ontogenetic shifts in habitat use, with adults excluding recently settled juveniles from some habitat types (e.g., Apogon novemfasciatus, Mulloidichthys sp., Neoniphon sammara).
Overall, the present study is the first to explore the use of space by a broad range of nocturnal fish taxa between recently settled juvenile and adult life stages. This multi-species perspective offers a broader survey of the varied ways in which fish can redistribute themselves post-settlement than has been done in previous work [8]. Similar nocturnal studies are now required to establish the spatio-temporal generality of the five ontogenetic patterns highlighted at Moorea Island and allow thus to better understand differences observed in ontogenetic patterns between nocturnal and diurnal organisms.