1 Introduction
Chickpea (Cicer arietinum L.) is a major food legume and an important source of protein in many countries in Asia and Africa. This species is the second most consumed and the third most cultivated grain legume [1]. It is cultivated on large surfaces in the world; nevertheless, many biotic and abiotic stresses limit the productivity of this legume: leaf diseases, salinity, drought, cold, and micronutrients deficiencies [2]. Several studies are involved in the exploration of stress-resistant chickpea varieties [3].
The role of Fe as an essential nutrient and its function in metabolism have been investigated in detail by many studies [4,5]. Iron is abundant in most soils; however, under alkaline or calcareous conditions, the physicochemical properties of Fe dictate the formation of highly insoluble Fe oxides and hydroxides, making Fe limiting for plant growth [5]. Under aerobic conditions, the Fe solubility in mineral soils is too low to fulfil the plant demand for Fe. According to Lindsay [6], most plants would show Fe deficiency when grown in a medium above pH 5. Even in acidic soils, iron can be decreased if it is not maintained by complexing ligands [7]. It has been shown that many reducing compounds released by roots, such as phenolic substances (caffeic acid or acid aliphatic) and natural chelates (phytosiderophores) can increase iron solubility [8]. In the same way, the soil microbial activity plays an essential role in Fe acquisition [9].
In the Mediterranean area, including Tunisia, calcareous soils are frequent. The high level of bicarbonate ions in the soil affects metabolic processes in roots and leaves, decreasing soil and plant Fe bioavailability [10], leading to the condition known as lime-induced iron chlorosis. In this region, iron chlorosis represents a major constraint for the majority of legumes, particularly those intended for the production of seeds [11–13]. It leads to both straw and seeds yield losses in several legumes [11,12,14–17].
Since soil amendment and foliar spray with iron salts are highly costing and do not always improve the iron nutrition of the plants [18,19], the exploration of phytogenetic resources to identify iron-chlorosis-tolerant species and varieties could help to improve crop productivity in calcareous soils. In fact, a large variability of response to Fe constraint has been highlighted, either among legume species, or among varieties [20–24].
When grown under Fe-limiting conditions, plants could compensate for the lack of Fe through an increased Fe uptake capacity. Fe-efficient genotypes develop controlled responses, which include physiological, biochemical and morphological changes [25].
The legumes implement several reactions to solubilize iron at the root surface, notably the extrusion of H+ protons leading to rhizosphere acidification [26], the exudation of reducing substances, accelerating the reduction of Fe3+ to Fe2+, the accumulation of organic acids and other ‘chelating’ iron compounds in the roots, as well as the expression of efficient Fe3+ reductase and Fe2+ transporters [27,28].
Iron resupply to Fe-deficient plants restores many plant functions. For instance, it increases Chl concentration and restores photosynthetic activity in sugar beet [29,30]. In addition, Fe resupply to Fe-deficient plants increases Fe concentration in roots and leaves of sugar beet [31,32] and maize [33–35], and was shown to decrease the citrate concentration in the xylem sap in sunflower [36–38], and to increase the total organic acid concentration in sugar beet leaves [32].
In this work, an attempt has been made to evaluate the effects of Fe deficiency on two hydroponically grown chickpea varieties (INRAT88 and Chetoui) using morpho-physiological and biochemical parameters. Special attention is given to the effect of Fe resupply on these parameters after a period of Fe starvation to further understand the responses of chickpea to iron deficiency.
2 Materials and methods
2.1 Plant material and cultivation conditions
Experiments were carried out on two varieties of chickpea (Cicer arietinum L.) commonly cultivated in Tunisia: INRAT88 (selected in the Tunisian National Institute of Agronomic Research: INRAT) and Chetoui (cultivated in winter in Tunisia). The seeds were disinfected in a 6% hydrogen peroxide solution during 10 min, and then abundantly rinsed in distilled water. After a 2-h imbibition phase, they were germinated in darkness at 25 °C on a suitable germination solution (CaCl2 600 μM, H3BO3 10 μM). After four days, germinated seeds were transferred into distilled water for seven days. Then, the seedlings (3–4-leaflet stage) were transferred into a continuously aerated solution (400 mL air min−1) in 500-mL glass bottles. The nutrient solution was composed of: KH2PO4 (0.125 mM), KNO3 (0.75 mM), Ca(NO3)2 (0.625 mM), MgSO4 (0.25 mM), H3BO3 (0.5 μM), MnSO4 (0.5 μM), CuSO4 (0.045 μM), ZnSO4 (0.05 μM), (NH4)6Mo7O24 (0.02 μM). Iron was added to the medium as Fe(III)–Na–EDTA. The solution was renewed every three days.
Two treatments were employed: 20 μM Fe (control, +Fe), 0 μM Fe (deficient, –Fe). After 12 days of treatment (corresponding to 19 days after sowing) when chlorosis symptoms are visible on young leaves of both Chetoui and INRAT88, a lot of deficient plants (nine plants per variety) were re-alimented with iron at the same concentration as control plants (–Fe/+Fe treatment).
All experiments were carried out in a greenhouse under controlled conditions (day/night: 16/8 h, 22/18 °C, 60/80% relative humidity and a light intensity of 250 μmol m−2 s−1 PAR). Eight harvests were carried out, respectively after 0, 3, 6, 9, 12, 15, 18 and 21 days of treatment, corresponding to 7, 10, 13, 16, 19, 22, 25 and 28 days after sowing. At each harvest, three plant per treatment were divided into leaves, stems and roots; weighed (for fresh weight determination: FW) and put in the drying oven (at 60 °C for 72 h for desiccation), then weighed again (for dry weight determination: DW).
2.2 Chlorosis score
The primary visible symptom of Fe deficiency is the development of intercostal chlorosis, principally on young leaves. The severity of Fe-deficiency symptoms is daily performed using the 0–4 index proposed by Gildersleeve and Ocumpaugh [39]: 0 for green leaves; 1 for leaves with slightly yellow margins; 2 for leaves showing distinct yellowing over most of the leaf but green mid-vein; 3 for leaves completely yellow; 4 for largely necrotic leaves.
2.3 Leaf chlorophyll concentration
Chlorophyll concentration (mg g−1 FW) was determined according to the Torrecilas et al. [40] method, slightly modified (use of pure acetone rather than acetone 80%). The sub-apical leaves (1 to 2 leave(s) per plant) were harvested, immediately weighed, crossed in discs, then used for the extraction of chlorophyll assessment. Five millilitres of pure acetone were added to fresh leaf samples (approximately 100 mg). The total extraction took place after 72 h in darkness, at 4 °C. The extract's absorbance was measured at 649 and 665 nm.
2.4 Determination of acidification capacity
The pH of the nutrient solution, initially fixed at 7–7.5, was monitored during the treatment period. The pH measurements were made using a digital pH-meter (Metrohm 663).
2.5 Iron content
Harvested plants were separated in roots, stems, and leaves. After desiccation in a drying oven at 60 °C for 72 h, the samples were finely crushed in a standard agate crusher in order to avoid powder contamination by iron traces. Total iron extraction was achieved by subjecting the powder to nitroperchloric acid attack according to Grusak [41], and Fe were quantified by atomic absorption spectrophotometry (Varian SpectrAAS 220).
2.6 Fe use-efficiency
To investigate the parameters implied in the genotypic differences between INRAT88 and Chetoui, we calculated the Fe-use efficiency for chlorophyll synthesis and plant growth, which are defined as the ratio of leaf total chlorophyll (mg) to leaf Fe concentration (μmol Fe) and the ratio of leaf or root biomass (mg) to leaf or root Fe concentration (μmol Fe).
2.7 Statistical analysis
Data were compared between varieties, treatments and treatment stage by MUSTAT software using the Fisher's LSD test at the P< 0.05 confidence level. Values were the means of three replicates.
3 Results
3.1 Chlorosis symptoms
Chlorosis appearance and expansion in –Fe plants showed differences between varieties. After three weeks of treatment, the chlorosis index was 3-fold higher in Chetoui than in INRAT88 (Fig. 1). The relative tolerance of INRAT88 as compared to Chetoui was confirmed by the behaviour of resupplied (–Fe/+Fe) plants. Indeed, after nine days of resupply, the INRAT88 chlorosis index decreased to the level of the control (+Fe) plants. In Chetoui, the chlorosis index was diminished following the restoration of Fe supply, but it remained significantly (

Changes in chlorosis score in INRAT88 and Chetoui varieties cultivated with or without 20 μM Fe during 21 days and the effect of Fe resupply after 12 days of iron deprivation. Different letters correspond to significantly different values (P<0.05, n=3).
3.2 Leaf chlorophyll concentration
Determination of chlorophyll concentration in young leaves (Fig. 2) confirmed the morphological monitoring. In control medium, the chlorophyll concentration in both varieties decreases, throughout the experiment and especially at the beginning. However, differences appeared under Fe-limiting conditions. In INRAT88, the difference between +Fe and –Fe treatments is significant after 15 days, whereas it becomes significant, for Chetoui only, after 6 days. In the former species, the chlorophyll –Fe/chlorophyll +Fe ratio decreases drastically (68%) since the 6th day of treatment; however, in INRAT88, it starts decreasing after 15 days (Fig. 3) and does not exceed 38%. The addition of iron to Fe-deficient INRAT88 plants after 12 days of deprivation restored the chlorophyll concentration of young leaves to that of control plants within 9 days. In the same conditions, the restoration was not complete in Chetoui plants.
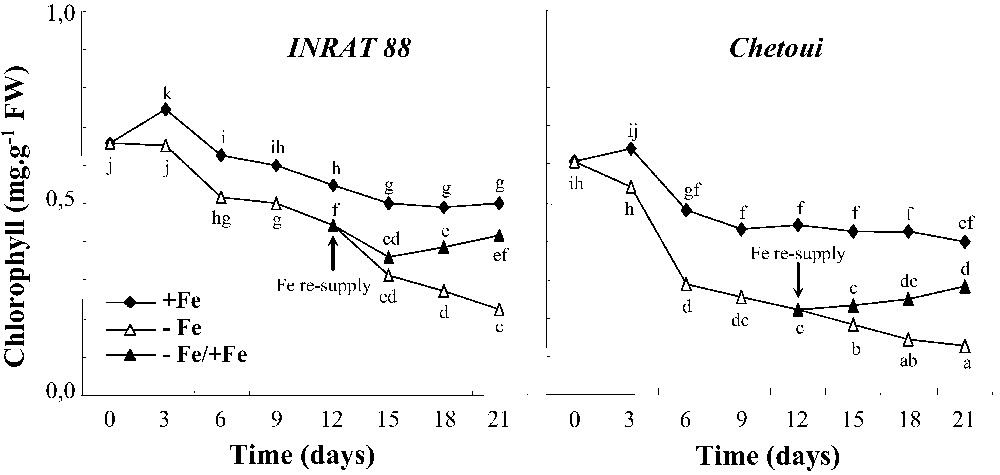
Leaf total chlorophyll concentration changes during the period of treatment, in INRAT88 and Chetoui. Different letters correspond to significantly different values (P<0.05, n=3).

Variation of Chlorophyll –Fe/Chlorophyll +Fe ratio in leaves during the period of treatment, in INRAT88 and Chetoui. Different letters correspond to significantly different values (P<0.05, n=3).
For INRAT88, at the beginning of the experiment, the Chla/Chlb ratio decreases with time in both treatments, whereas it remains steady for Chetoui (Fig. 4). Then, the ratio increases for varieties under +Fe treatment and decreases continuously in –Fe treatment in both varieties. The differences between +Fe and –Fe plants are significant after 12 days in INRAT88 and after 9 days only in Chetoui.

Variation of Chla/Chlb ratio in leaves during the period of treatment, in INRAT88 and Chetoui. Different letters correspond to significantly different values (P<0.05, n=3).
3.3 Plant growth
Under control conditions, Chetoui showed a more active growth, especially at the end of the treatment at the whole-plant (Fig. 5) and root (not shown) levels. Iron deficiency significantly inhibited whole-plant biomass deposition in both varieties (Fig. 5). However, growth reduction appeared earlier and was more pronounced in Chetoui than in INRAT88. Furthermore, the re-introduction of Fe in the medium did not significantly improve Chetoui's growth, but resulted in a large biomass augmentation of INRAT88.
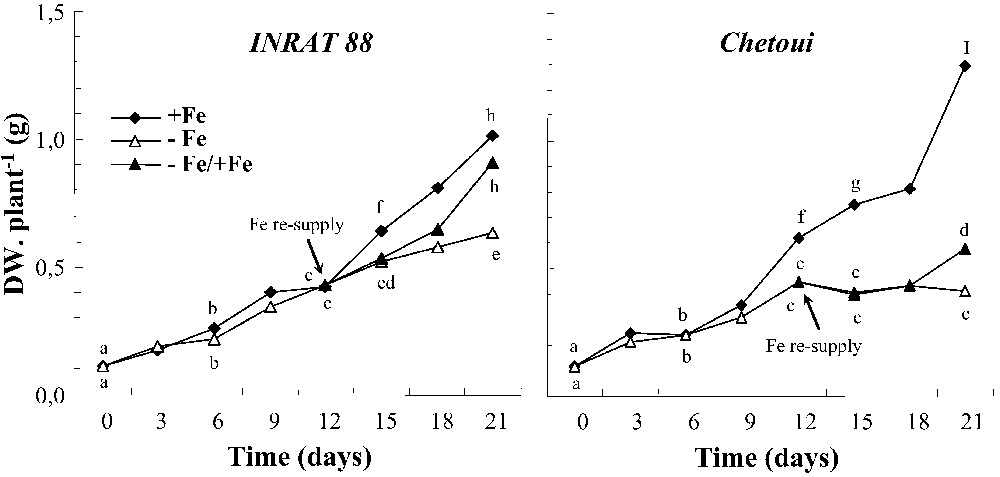
Whole-plant biomass production (dry matter) of INRAT88 and Chetoui cultivated with or without 20 μM Fe during 21 day and the effect of Fe resupply after 12 days of iron deprivation. Different letters correspond to significantly different values (P<0.05, n=3).
3.4 Acidification capacity
The root capacity to acidify the medium in response to Fe deficiency was much higher in INRAT88 than in Chetoui (Fig. 6). At the beginning of the experiment, the medium pH was decreased in both treatments for the two varieties. Then, on control nutrient solution, the pH remained steady in both varieties. In contrast, under Fe-deficient conditions, this parameter decreases significantly (three units) during a 12-day treatment in INRAT88, whereas a slight decrease was observed only after 12 days in Chetoui. Iron resupply resulted in a rapid increase of the pH of the medium.
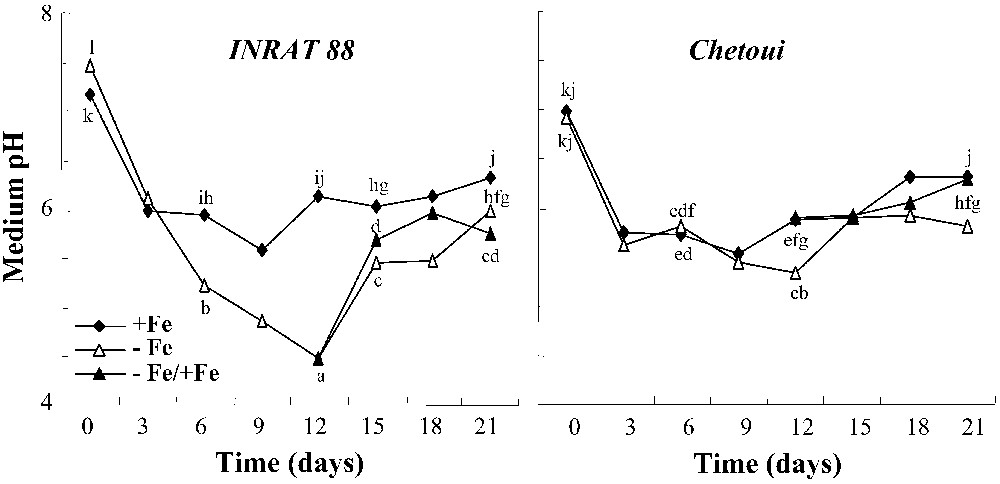
Changes in nutrient solution pH of control and deficient plants during the treatment period. Different letters correspond to significantly different values (P<0.05, n=3).
3.5 Iron content in the different subparts of the plants
Changes in whole-plant iron content (microgram per plant) in plants after 6, 12, 15 and 21 days of treatment are represented in Fig. 7. Over this period, this parameter decreased dramatically under deficient conditions. We note that Fe contents in INRAT88 were constant beyond 6 days of treatment in control as well as in deficient plants. In both varieties, Fe re-introduction into the medium led to significant increases in whole-plant Fe content. However, we note that in INRAT88, the Fe content of resupplied plants reached that of control plants at the end of the treatment, while it remains significantly low compared to control plants in Chetoui.
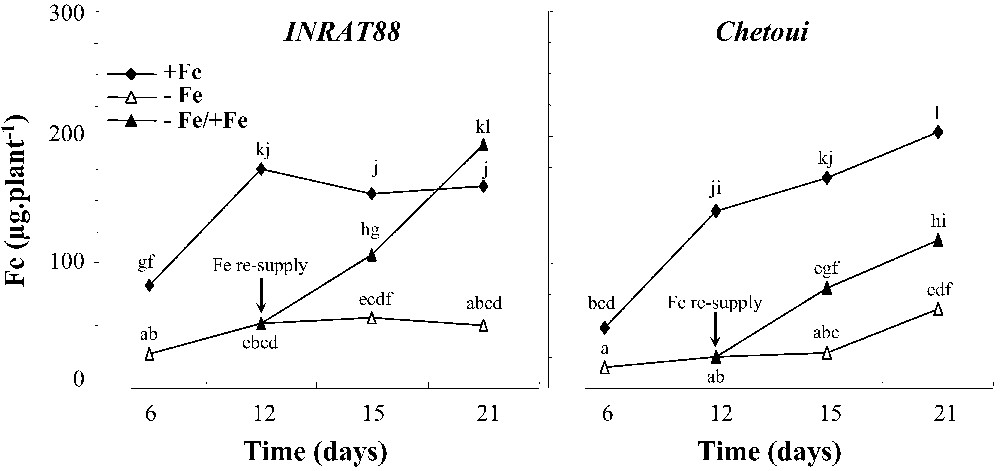
Whole-plant iron content in INRAT88 and Chetoui after 6, 12, 15, and 21 days of treatment. Different letters correspond to significantly different values (P<0.05, n=3).
In Fe-deficient roots, the decreases in the iron content (microgram per plant) were more important than in leaves (not shown). As a result, the repartition of iron in the whole plant changed; shoot Fe amounting to ca. 20–25% of the whole plant in control condition, and to ca. 50% in Fe deficiency conditions (Fig. 8). The re-addition of iron to deficient plants cancels this effect, and the resupplied plants showed the same iron distribution within plant organs as in control plants.
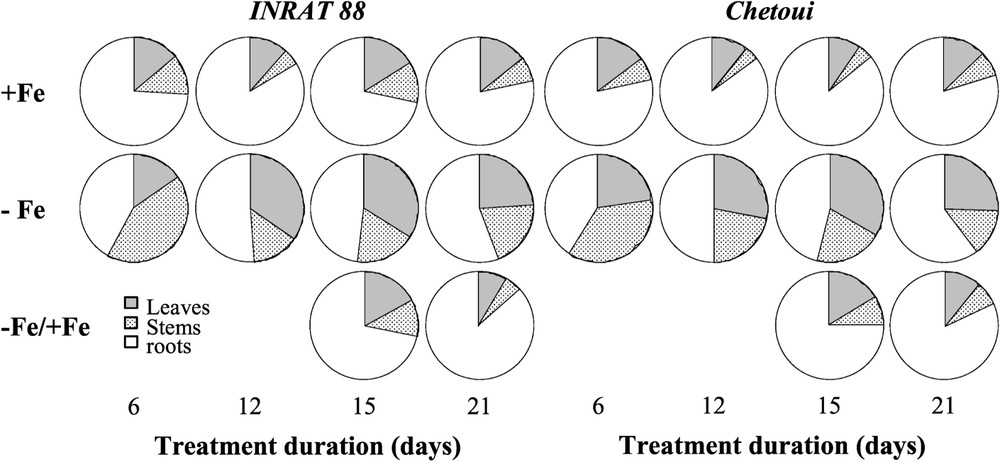
Iron repartition within plant organ in INRAT88 and Chetoui after 6, 12, 15, and 21 days of treatment. Data are means ± SE (n=3).
3.6 Fe use-efficiency
At the beginning, Fe-use efficiency is higher in INRAT88 for both treatments. After six days of Fe deficiency, this parameter was two times higher in INRAT88; then it decreased continuously, but remained higher than in Chetoui (Fig. 9). For leaf and root growth (Fig. 10), Fe-use efficiency is higher in INRAT88-deficient plants, particularly at an advanced stage of the treatment.

Fe use efficiency for chlorophyll synthesis in INRAT88 and Chetoui after 6, 12, 15, and 21 days of treatment. Different letters correspond to significantly different values (P<0.05, n=3).
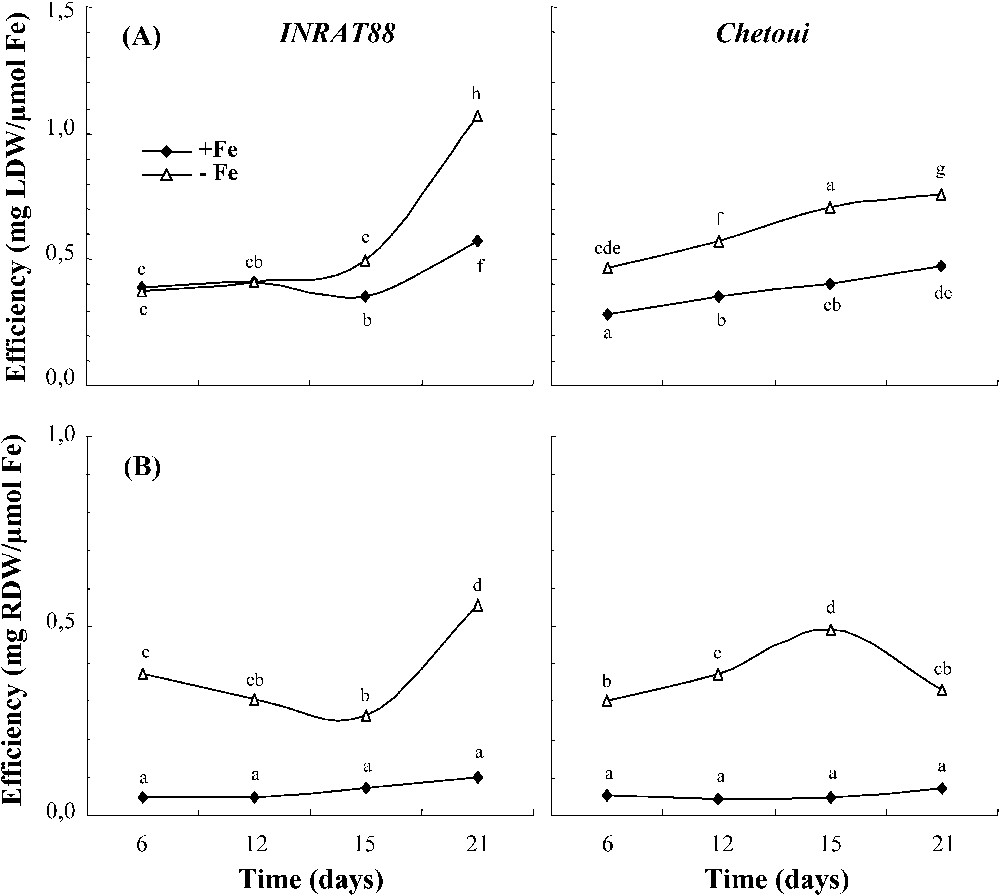
Fe use efficiency for leaf (A) and root growth (B) in INRAT88 and Chetoui after 6, 12, 15, and 21 days of treatment. Different letters correspond to significantly different values (P<0.05, n=3).
4 Discussion
Iron deficiency causes nutrient imbalances in different parts of the plant. For instance, chlorophyll status and leaf mineral composition were generally affected [42–44]. In fact, the monitoring of plant morphological aspects showed high chlorosis score in deficient plants, which is attested by a large decrease in chlorophyll concentration. These effects were shown at an early stage in Chetoui, nine days before INRAT 88. Similar chlorophyll concentration changes were reported in chickpea [11,13], pea [44], and sunflower leaves [45,46].
The large decrease of the Chla/Chlb ratio suggests that under iron starvation, Chla is more affected than Chlb. Nevertheless, several studies showed that the latter decreased more than the first chlorophyll form [47], or that both are affected, the Chla/Chlb ratio remaining stable [48]. It appears that our results are not consistent with what is described in the literature. These differences could be ascribed to the treatment duration, which is longer in the present study.
The values of chlorophyll may give information, not only about the degree of chlorosis, but also about the differential behaviour of genotypes to Fe chlorosis [49]. It seems that INRAT88 maintained a significantly higher chlorophyll concentration compared with Chetoui (Fig. 2), suggesting that the latter is more sensitive to Fe chlorosis. Indeed, when comparing plant growth, we note that Chetoui's deficient plants are much more affected than those of INRAT88, particularly at the end of the treatment (Fig. 5).
In plants subjected to iron deficiency, chlorophyll concentration was significantly higher in INRAT88 than in Chetoui, particularly at the end of the treatments. This behaviour was also concomitant with a higher growth in the first variety. This result suggests that iron requirements for growth and for chlorophyll synthesis are higher in Chetoui than in INRAT88. Furthermore, we note that Chetoui plants supplied with Fe (control) showed a more active growth especially at this stage, which can explain a higher iron requirement for this variety.
Plants' Fe content (μg plant−1) was severely decreased by Fe deficiency in Chetoui as well as in INRAT88, which led to a re-distribution within plant organs; the iron deficiency increased the shoot part in the allowance of this nutrient, at the expense of roots (Fig. 8). Despite these changes, after 12 days of treatment, the whole-plant Fe content remains stable in INRAT88 (180–190 μg plant−1 in control plants and 50 μg plant−1 in deficient plants), whereas it increased continuously in Chetoui. It seems that the latter is more exigent for iron than INRAT88. Furthermore, Chetoui plants supplied with Fe (control) showed a more active growth, especially at the end of the treatment, which could explain a higher iron requirement for this variety. In addition, INRAT88 deficient plants showed a higher Fe-use efficiency for leaf and root growth, particularly at this stage. For chlorophyll synthesis, we note a better Fe-use efficiency for INRART88 leaves during the treatment period, and especially at the beginning (Fig. 9), which suggests a better management of its iron content.
On the other hand, the best performance of INRAT88 under iron starvation could be also ascribed to its stronger acidification capacity. Indeed, it is well known that acidification and Fe3+ reduction, characteristics typical of strategy I, constitute the first means of iron mobilization under these conditions, and it has been suggested that acidification could be used for screening of iron-deficiency-tolerant plants [50].
We showed in a previous work [51] that the chlorosis resistance of chickpea is related to its capacity to preserve the integrity of its leaves against ferrous iron impoverishment, by increasing iron acquisition from the culture medium, owing to the medium acidification enhancement, which confers to these organs the capacity to conserve their chlorophyll status.
In strategy-I plants, iron mobilization is achieved by the combined action of a proton-extruding H+-ATPase and a ferric chelate reductase, both enzymes being induced by iron deficiency [26,52]. In addition, the patterning of epidermal root cells is characteristically altered by iron availability, thereby increasing the absorptive surface area of the roots. For example, root hair density is significantly increased in response to iron shortage [53], whereas mobilization of iron is achieved in grasses (strategy II) by the secretion of phytosiderophores from the mugineic acid family (MAs), synthesized from l-methionine via nicotianamine. There are large differences in both quality and quantity of MAs in different graminaeceous species. The expression of nicotianamine synthase and nicotianamine aminotransferase is increased by Fe-deficiency, leading to a higher production of MAs under such conditions [54].
Based on growth parameters, acidification capacity, chlorophyll and nutrient contents, we can estimate that Chetoui is less tolerant to Fe chlorosis than INRAT88. However, to differentiate further between these two Tunisian varieties, the effects of iron resupply on these parameters were evaluated.
In our study, we remark that, upon Fe resupply, the pools of leaf chlorophyll concentration increase, and we note a general leaf re-greening, especially in INRAT88. The same Fe resupply improves biomass productivity, mainly for INRAT88, and enhances plant Fe concentrations. Similar effects have been reported in sugar beet [31,32] and maize [36]. In Chetoui, we note that iron re-addition to deficient plants has no significant effects, neither for plant growth retaliation, nor for chlorotic status. This finding confirms the sensitivity of this variety to iron-limiting conditions.
In conclusion, we note that under iron starvation, INRAT88 showed a better Fe-use efficiency, which allows this variety to maintain plant growth and to preserve adequate chlorophyll concentration. We suppose that, in addition to some morpho-physiological and biochemical responses, the resupply of deficient plants with iron and its effects on these parameters can indicate that these plants are susceptible to support Fe starvation.
Acknowledgements
We wish to thank Professor Claude Grignon, director of the ‘Laboratoire de biochimie et physiologie moléculaire des plantes’ (ENSA/INRA, Montpellier, France) for reading the manuscript and providing helpful comments. Thanks are also due to two anonymous reviewers for additional valuable comments.