1 Introduction
Girolline was extracted from the marine sponge Cymbastela cantharella (Pseudaxinyssa cantharella), collected in New Caledonia [1]. Determination of its chemical structure and biological properties revealed that girolline is a 2-aminoimidazole derivative with cytotoxic activity [2]. Girolline affected the survival of exponentially growing leukaemic cells and had a significant antitumour activity in several models of grafted murine tumours in vivo [3]. However, girolline did not exhibit any antitumour activity in a phase-I clinical trial, but had severe cardiovascular toxicity [4].
Studies on the incorporation of radiolabelled DNA, RNA and protein precursors in cultured cells indicated that girolline at 1.5 μM concentration had no effect on DNA and RNA synthesis, but inhibited protein synthesis in leukaemic cells [3]. Further in vitro studies using rabbit reticulocyte lysate showed that girolline at high concentration (100 μM) did not affect initiation and elongation of translation, but interfered with the release of the nascent polypeptide, suggesting an effect on translation termination [5].
Recently, Tsukamoto et al. [6] have shown that cells treated for 24 h with 50 μM of girolline exhibit a G2/M cell-cycle arrest and an intracellular accumulation of polyubiquitinated p53.
To go further into the mechanism of action of girolline in vivo, we have evaluated its effect on translation and on cell cycle progression. We show that girolline neither induced an increase in translational readthrough nor affected the polysome profile of treated cells. Cell-cycle analysis shows that girolline induced a dose- and time-dependent arrest of cells in G2 phase. Altogether, these results suggest that girolline does not act on translation in vivo, but interferes with the control of the G2 checkpoint.
2 Materials and methods
2.1 Cell culture and drug treatment
The 559C cell line is a derivative of human 293 cell line (ATCC N° CRL-1573) stably expressing a lacZ gene in which the coding sequence is interrupted by a TAG stop codon [7]. 559C cells were maintained in Dubelcco modified Eagle's minimum essential medium (DMEM; Invitrogen) supplemented with 10% foetal calf serum, 100 μg/ml streptomycin and 100 units/ml penicillin. Girolline was extracted from the marine sponge Cymbastela cantharella (Pseudaxinyssa cantharella) and purified as described [2]. Solutions of girolline were prepared in sterile water. The stock solution (10 mM) was stored at −20 °C and other dilutions were used immediately. For drug treatment, 100-mm-diameter plates of 559C cells at 50% confluency were washed with 10 ml of PBS and incubated at 37 °C with 10 ml of DMEM supplemented with 10% foetal calf serum (lacking streptomycin and penicillin) and, either girolline, or gentamicin (Sigma) at concentration indicated in the text and figure legends. After treatment, the medium was removed and the cells were collected by scraping in 10 ml of PBS, and pelleted.
2.2 Readthrough assay
The readthrough assay was described in [7]. Briefly, cell pellets resuspended in 300 μl of 100 mM sodium phosphate buffer, pH 8, were lysed by four cycles of freezing, and thawing and cell lysates were centrifuged at 12,000 g for 30 min at 4 °C. Supernatants were assayed for total proteins using the Micro BCA Protein Assay Reagent Kit (Pierce) and β-galactosidase activity using the luminescent beta-galactosidase Detection Kit II (Clontech) following the manufacturer instructions. The β-galactosidase activity was expressed as relative light units (RLU)/μg of total proteins, and the readthrough efficiencies were calculated as sample activity relative to negative control activity.
2.3 Polysome analysis
Isolation of polysomes was performed according to [8]. Either four (control cells) or eight (girolline treated cells) 100-mm cell plates were used for the gradients. After 48 h of treatment, cells were incubated for 2 h with 10 ml of fresh medium without antibiotic or drug, and cycloheximide was added at 100 μg/ml for 5 min. Cells were then collected by trypsinization and pelleted. The cell pellet was resuspended in 500 μl of lysis buffer (50 mM Tris-HCl [pH 7.4], 300 mM KCl, 10 mM mg-acetate, 1 mM DTT) containing 130 units of RNAse inhibitor (Amersham Pharmacia Biotech) and 100 μg/ml of cycloheximide, and lysed by adding ∼200 μl of glass beads and vortexing on ice for 30 s. Nuclei and cell debris were removed by centrifugation at 1,000 g for 10 min and an aliquot fraction of supernatant corresponding to 25 units of optical density (OD) at 260 nm was layered onto a 12 ml 15–50% (w/v) sucrose gradient. The gradient was centrifuged at 39,000 rpm in a SW41 Beckman rotor for 3.25 h at 4 °C. Absorption at 254 nm was recorded by pumping the gradient through a single-path UV-1 monitor system (Pharmacia).
2.4 BrdU labeling and flow cytometry analysis
For BrdU labeling, cells were incubated with 9 μg/ml BrdU for 30 min in complete culture medium. After labelling, cells were rinsed twice with PBS and further cultured in complete medium. For flow cytometry analysis, cells were trypsinized, resuspended in media, spun down, and resuspended in 100 μl of complete medium. While vortexing, 2 ml 70% ethanol were added and cells were stored at −20 °C. Non-labelled fixed cells were pelleted by centrifugation, resuspended in PBS and stained at 37 °C for 30 min in 40 μg/ml propidium iodide and 100 μg/ml RNAseA. BrdU-labelled cells were pelleted by centrifugation, gently resuspended in 1 ml 2N HCl, 0.5% Triton X100 and incubated for 30 min at room temperature. Cells were then pelleted by centrifugation, resuspended in 1 ml 0.1 M sodium tetraborate pH 8.5, pelleted again and washed with PBS containing 0.5% Tween 20, 1% BSA. Then, cells were incubated with anti-BrdU monoclonal antibodies (Beckton Dikinson) for 30 min at room temperature, washed twice with PBS containing 0.5% Tween 20, 1% BSA, and incubated with Alexa Fluor 488 goat anti-mouse IgG (Molecular Probes) for 30 min at room temperature. Cells were resuspended in PBS and stained at 37 °C for 30 min in 40 μg/ml propidium iodide and 100 μg/ml RNAseA. Finally, stained cells were analyzed using a Coulter Elite-ESP flow cytometer system (Beckman-Coulter, France) and percentages of cells in the G1, S, and G2/M phases of the cell cycle were determined using the MultiCycle program. Figures were processed using the WinMDI 2.8 program.
3 Results and discussion
3.1 Girolline treatment did not increase translational readthrough and did not affect polysome distribution
Factors acting on the ribosome decoding site such as aminoglycosides [9] and factors that decrease translation termination efficiency [7,10,11] can promote translational readthrough in cultured cells. As it has been described that in vitro, girolline inhibited protein synthesis by interfering with the termination step [3], we have tested the effect of girolline on translational readthrough in human cultured cells. In a first set of experiments, we compared girolline with gentamicin, which is commonly used for studies on aminoglycosides-mediated translational readthrough. The efficiency of stop codon readthrough was measured in human cells stably expressing a lacZ reporter gene interrupted by a premature nonsense TAG codon and encoding a truncated inactive β-galactosidase-559C cell line. The synthesis of full-length active β-galactosidase required stop codon readthrough and thus, the level of β-galactosidase activity reflected readthrough efficiency. Parallel cultures of 559C cells were treated for 48 h with either girolline at 0.1 and 1 μM or gentamicin at 1 mg/ml, and the β-galactosidase activity was measured in cell extracts. The relative readthrough efficiency was calculated by dividing the β-galactosidase activity in each sample by the β-galactosidase activity in the extract of non-treated cells (Fig. 1). A clear increase in readthrough was observed for cells treated with gentamicin (1 mg/ml), whereas girolline used at both concentrations had no effect. Moreover, the concomitant treatment of cells with gentamicin (1 mg/ml) and girolline (1 μM) reduced the readthrough level obtained with gentamicin alone. This is probably due to a toxic effect of girolline on cells. As shown in Fig. 1, this toxic effect is likely also responsible for the decrease in readthrough observed for cells treated with 1 μM girolline when compared to control non-treated cells. Girolline at higher concentrations (5 and 10 μM) induced a rapid arrest of cell growth and a massive loss of cell adherence (not shown). The absence of increase in readthrough suggested that, in vivo, girolline did not reduce the efficiency of translation termination and probably did not interfere with the release of the nascent polypeptide, as previously proposed [5].

Girolline does not promote readthrough of stop codons in human cells. Parallel cultures of 559C cells stably expressing a lacZ gene containing a premature UAG stop codon were treated for 48 h with 0.1 μM girolline (Giro 0.1 μM), 1 μM girolline (Giro 1 μM), 1 mg/ml gentamicin (Genta 1 mg/ml), and 1 μM girolline plus 1 mg/ml gentamicin (Genta + Giro); non-treated cells (NT) served as negative control. The β-galactosidase activity (relative light units/μg of protein) was measured in cell extracts. The relative readthrough efficiency was calculated by dividing the β-galactosidase activity in each sample by the β-galactosidase activity in the extract of non-treated cells. Results were expressed as the mean of four experiments; error bars show the standard error of the mean.
The sedimentation profile of polysomes is a good reflect of the translational status of cells. Translation arrest at initiation and elongation steps induces a decrease in the number of ribosomes per mRNA molecule and an increase in free ribosomes and ribosomal subunits. In addition, we have observed that, in human cells, translation termination inhibition induced by depletion of release factor eRF3a resulted in an extensive breakage of polysomes into ribosomes (unpublished observation). Thus, to evaluate the potential effect of girolline on global translation, we compared the polysomal distribution of non-treated and girolline-treated cells. Due to the inhibition of cell growth induced by two days of treatment with 1 μM of girolline, eight plates of girolline-treated cells versus four plates of non-treated cells were used for the gradients. Cell extracts corresponding to 25 O.D. at 260 nm were fractionated by sucrose gradient centrifugation, as described in Section 2. As shown in Fig. 2, girolline at 1 μM did not modify the sedimentation profile of polysomes, neither decreasing the number of peaks in the polysome fractions, nor affecting the intensities of polysome and free 80S ribosome peaks. This result strongly suggested that, despite a clear inhibition of cell growth, girolline did not block translation and even more did not affect the translatability of mRNAs in vivo.
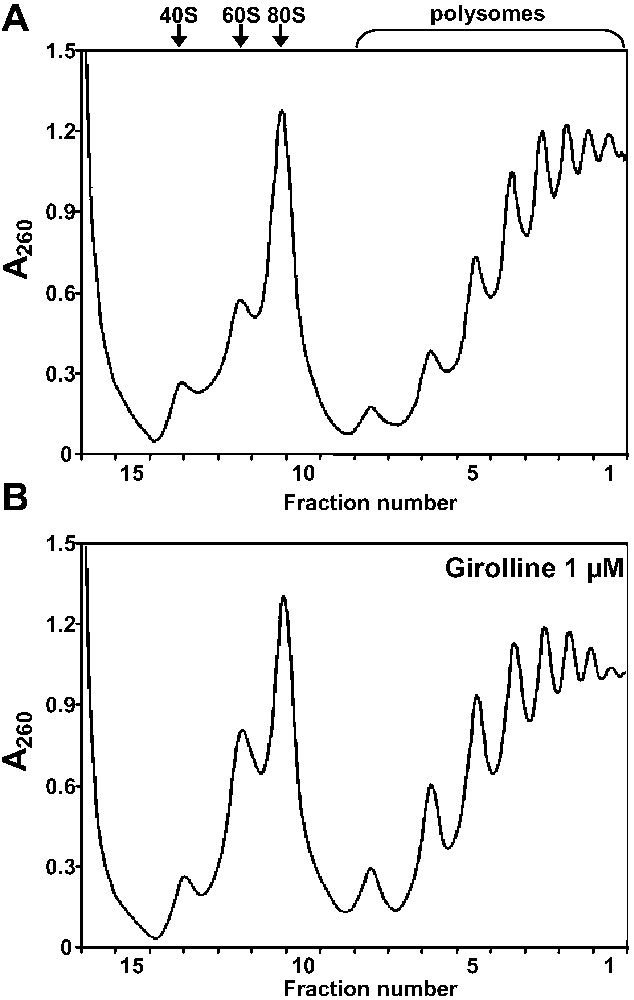
Polysome distribution in girolline-treated cells. Cytoplasmic extracts of non-treated 293 cells (A) or 293 cells treated with 1 μM girolline for 48 h (B) were fractionated by 15–50% sucrose gradient centrifugation, as described in Section 2. The absorbance profile of the gradient is shown (sedimentation was from left to right), the collected fractions are indicated in x-axis. The sedimentation positions of polysomes, 80S ribosomes and 60S and 40S ribosomal subunits are indicated above the absorbance profile.
This discrepancy between the results of Lavelle et al. [3], showing an inhibition of translation by girolline, and our results could be explained by the difference in the experimental systems used. Indeed, most of the experiments of Lavelle et al. [3] were performed in rabbit reticulocyte lysate with higher concentrations of girolline than those we used in our in vivo experiments. However, other experiments using rabbit reticulocyte lysate [5] have shown that 100 μM girolline did not modify the polysome profile, whereas the elongation inhibitors bruceantine caused extensive polysome breakage. This latter observation is in good agreement with the result of our in vivo experiments. Thus, it is hardly conceivable that, in vivo, girolline inhibited translation termination without affecting ribosome recruitment into polysome. Therefore, the conclusion of our in vivo experiments is that girolline does not directly affect one of the steps of the translation process. In their report, Lavelle et al. [3] have described an inhibition of methionine incorporation in girolline-treated cells and suggested that this inhibition of protein synthesis could be due to a defect in the translation process. However, the inhibition of protein synthesis could also be an indirect consequence of the alteration of cellular processes other than translation.
3.2 Girolline blocks cell-cycle progression at the G2 phase
It has been shown that cells treated for 24 h with 50 μM of girolline exhibit a G2/M cell-cycle arrest [6]. However, the precise phase of the cell-cycle arrest (G2 or M phase) and the dose and time dependency of this inhibition were not assessed. Here, we investigated in details the effect of low concentrations of girolline on cell-cycle progression. 293 cells were treated for 48 h with increasing concentration of girolline and cell-cycle analysis was performed as described in Section 2. Minor changes were observed in cell cycle for cells treated with 0.5 μM of girolline, whereas cells treated with 1.0 μM of girolline exhibited an increased trend of cells in G2/M (Fig. 3A). Cell-cycle analysis revealed a progressive increase in G2/M cells from 7.8% to 32.1%, when girolline concentration increased and a concomitant decrease in cells in G0/G1 phase from 49.5% to 24.5%—the percentage of cells in S phase remained at the same level (Fig. 3B).

Girolline-treated cells are arrested in the G2/M phase of the cell cycle. (A) Flow cytometry analysis of 293 cells treated for two days with increasing concentrations of girolline as indicated. Cells were fixed, stained with propidium iodide, and analyzed by flow cytometry. Event count (y-axis) versus DNA content (x-axis) are shown. (B) Percentages of the cells in the G1, S and G2/M phases of the cell cycle in the experiment shown in (A). Percentage of cells analyzed with the MultiCycle program (y-axis) versus concentration of girolline (x-axis) are shown. (C) Flow cytometry analysis of 293 cells treated with 1 μM girolline for 1 to 4 days as indicated in z-axis. (D) Flow cytometry analysis of 293 cells treated with girolline at the concentration indicated in z-axis for 24 h, labelled with BrdU for 30 min, and further treated with girolline for 24 h. BrdU-labeled cell profiles are shown.
We next examined the time course of the girolline effect on cell cycle. Exponentially growing cells were treated with 1 μM of girolline and analyzed by flow cytometry at days 1, 2, 3, and 4 following the addition of the drug. As shown by the increase of 4N DNA peak, cell arrest in G2/M phase was more prominent (27.1%) by day 2 after addition of girolline (Fig. 3C). Then, the peak of G2/M cells decreased to 18.1 and 13.1% after three and four days of treatment, respectively. Concomitantly, an increase in pre-G0/G1 peak of apoptotic cells, from 1.9% at day 1 to 18.1% at day 4, was also noted (Fig. 3C). The percentage of cells in the G0/G1 phase decreased after two days of treatment and remained at the level of 30–35% at days 3 and 4. These results suggested that a fraction of cells arrested in the G2/M phase progressed toward cell death, whereas the cell cycle of the remaining fraction was significantly slowed down. However, the S phase did not seem to be modified, suggesting that DNA synthesis was not affected by girolline treatment, as previously observed [3].
To confirm these observations, cells were treated with girolline for 24 h, labelled with BrdU for 30 min, and further treated with girolline for 24 h. The percentage of labelled cells was roughly the same for non-treated cells (62.5%) and cells treated with 1 μM of girolline (64.5%). This further confirmed that girolline did not affect DNA synthesis. Twenty-four hours after BrdU labeling, most of the non-treated labelled cells have experienced a complete round of the cell cycle, and returned to the S phase (Fig. 3D), whereas labelled cells treated with 0.5 μM of girolline have progressed more slowly than non-treated cells, most of them being in the G1 phase, and a small portion being stalled in G2/M phases. The cell-cycle progression of 1 μM girolline-treated cells was even slower with almost 40% of labelled cells arrested in G2/M phases, 24 h after labelling (Fig. 3D). The results of BrdU labelling clearly showed that girolline induced an arrest of cell progression at the G2/M phases. To decide whether girolline induced cell arrest during mitosis or at the G2 stage, preventing the transition to mitosis, we have examined girolline-treated cells stained with anti-tubulin antibodies by fluorescence microscopy. The observation of girolline-treated cells revealed a drastic decrease of mitotic cells when compared to non-treated cells (data not shown). However, the scarce mitotic cells exhibited a normal mitotic spindle. The very low amount of mitotic cells strongly suggested that the cell cycle was not arrested during mitosis, but that girolline treatment prevented cells from passing the G2 checkpoint. The G2 checkpoint prevents cells from entering mitosis when DNA is damaged, providing an opportunity for repair and stopping the proliferation of damaged cells. Proper repair of DNA damage is critical for protecting genomic stability and cellular viability [12]. Both p53-dependent and p53-independent pathways have evolved to coordinate the cellular response following DNA damage. The 293 cells used in this study are defective in p53 and thus the p53-dependent response to DNA damage is probably not involved in the G2 arrest induced by girolline treatment. This is confirmed by the fact that girolline treatment of Hela cells that are also defective in p53 induced a G2/M arrest [6]. The regulation of G2/M checkpoint involves multiple overlapping pathways that could be targets of girolline.
In fine, our results showed that girolline probably does not interfere with the translation process. Taking in account that cap-dependent translation is slowed down at the G2 and M phases of the cell cycle [13], the G2 arrest of girolline-treated cells could explain the 34% decrease in the incorporation of radiolabelled methionine, as observed by Lavelle et al. [3]. Moreover, the dose- and time-dependent arrest of the cell cycle at the G2 phase induced by girolline indicates that its effect could be connected with a p53-independent mechanism controlling G2/M checkpoint regulation.
Acknowledgements
This work was supported by the ‘Association française contre les myopathies’ and by the ‘Association pour la recherche sur le cancer’ (grant No. 3784). Dialo Diop held a fellowship from the ‘Association française contre les myopathies’. Céline Chauvin held fellowships from the French ‘Ministère de la Recherche et de l'Enseignement supérieur’, from the ‘Fondation pour la recherche médicale’ and from the ‘Société française du cancer’. We thank Olivier Ganier for help with flow cytometry analysis and Jérôme Bignon for helpful comments.