1 Background based on morphological studies
1.1 Squamate evolution
The order Squamata includes lizards (∼4900 sp.), snakes (∼3070 sp.), and amphisbaenians (∼200 sp.) (Fig. 1). Together with the two extant species of tuataras from New Zealand, they form the Lepidosauria [1–5]. Previously, squamates have been divided into two major clades based on morphology: the Iguania (Iguanidae, Agamidae, Chamaeleonidae) and the Scleroglossa (Dibamidae, Gekkota, Amphisbaenia, Serpentes, Scincidae, Cordylidae, Gerrhosauridae, Xantusiidae, Lacertidae, Teiidae, Gymnophthalmidae, Anguidae, Anniellidae, Diploglossidae, Xenosauridae, Shinisauridae, Helodermatidae, Lanthanotidae, Varanidae) [6–15]. According to this arrangement, which has been widely accepted, a major event in squamate evolution was the switch from tongue prehension of food used by the muscular-tongued tuataras and iguanians to the teeth and jaw prehension of prey used by the hard (keratinized) tongued scleroglossans, freeing the tongue for chemoreception. This presumably allowed the scleroglossans to exploit a variety of habitats and foraging modes unavailable to iguanians and to dominate in squamate assemblages throughout the world [16–20]. Historically, three groups of squamates having limb reduction or loss (snakes, amphisbaenians, and dibamids) have been the most difficult to classify, because of their specialization and loss of characters. Nevertheless, most morphological studies support the inclusion of snakes within the anguimorphs, a group also comprising the anguids, anniellids, diploglossids, xenosaurids, shinisaurids, helodermatids, lanthanotids and varanids (see reviews by Evans [2] and Lee et al. [19]), while the dibamids and amphisbaenians are generally considered as Scleroglossa incertae sedis [6–15].
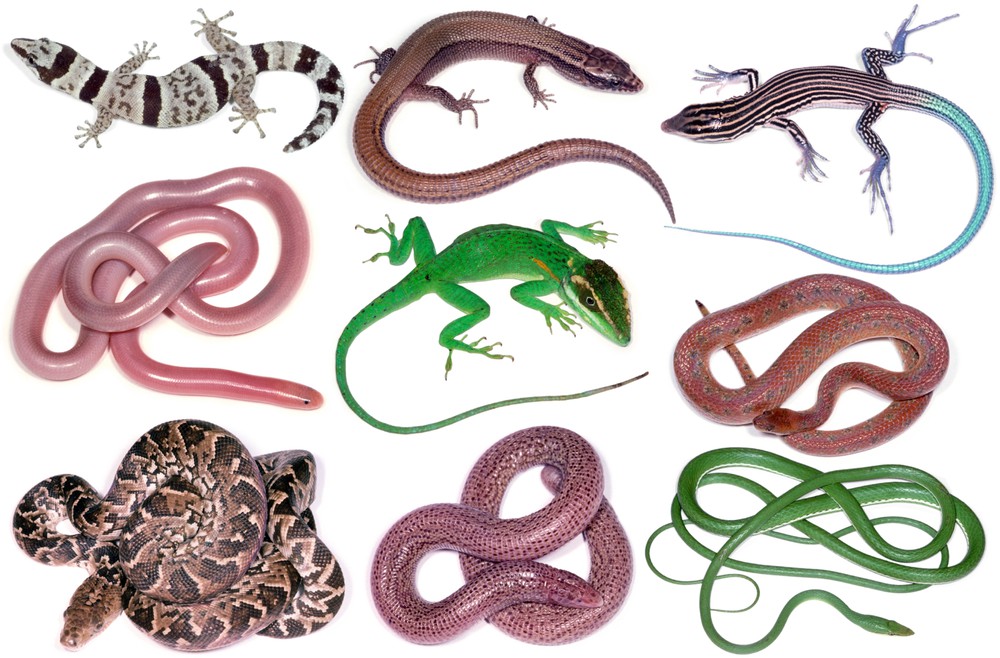
Representative squamates: top row (left to right), Sphaerodactylus cinereus (Sphaerodactylidae); Cricosaura typica (Xantusiidae); Ameiva lineolata (Teiidae); middle row, Typhlops anousius (Typhlopidae); Anolis noblei (Iguanidae); Tropidophis maculatus (Tropidophiidae); bottom row, Epicrates angulifer (Boidae); Cadea blanoides (Cadeidae); and Uromacer oxyrhynchus (Dipsadidae). Photos by S.B. Hedges.
1.2 Snake evolution
Snakes are among the most successful groups of reptiles, numbering about 3070 extant species [5]. They are divided into two main groups. The fossorial scolecophidians (∼370 sp.) are small snakes with a limited gape size and which feed on small prey (mainly ants and termites) on a frequent basis. The alethinophidians, or typical snakes (∼2700 sp.), are more ecologically diverse and most species feed on relatively large prey, primarily vertebrates, on an infrequent basis [21,22]. According to most morphological studies, a distinctive evolutionary trend within living snakes is the increase of the gape size from fossorial scolecophidians (Typhlopidae, Leptotyphlopidae and Anomalepididae) and fossorial alethinophidians (“Anilioidea” i.e. Aniliidae, Cylindrophiidae, Uropeltidae, Anomochilidae) to ecologically diverse macrostomatan alethinophidian snakes such as boas, pythons, and caenophidians (advanced snakes) ([21] but see [23]). The macrostomatan condition permits the ingestion of very large prey, sometimes greater in diameter than the predatory snake itself [24], and its monophyly is supported by several unambiguous synapomorphies [25]. All venomous snakes are found within the Caenophidia, which include the great majority of extant snakes (∼2500 sp.) [5]. Caenophidians comprise the aquatic acrochordids, the atractaspidids (some of them with a front-fanged venom system), the elapids and the viperids (all of them with a front-fanged venom system), and the huge and paraphyletic ‘colubrid’ family (defined by the absence of a front-fanged venom system), which includes the vast majority of caenophidians (∼1900 sp.) [26–31]. Since the end of the nineteenth century, the most commonly cited evolutionary trend of the venom apparatus is a progressive evolution culminating with the sophisticated solenoglyph apparatus displayed by vipers (long hollow fangs on a short and mobile maxillary) [32–38].
1.3 Amphisbaenian evolution
Amphisbaenians (∼200 sp.) are small, burrowing, and limbless squamates (small front limbs are present in three species) that differ significantly in terms of habits and morphology from snakes and lizards [39–41]. Amphisbaenian bodies are cylindrical and covered with smooth, square scales arranged in rings. Their skin moves independently of the trunk, facilitating rectilinear locomotion that is used for forward thrust in conjunction with head movements to widen their burrows [41]. Three of the five extant families have restricted geographic ranges and contain only a single genus: the Rhineuridae (genus Rhineura, 1 species, Florida), the Bipedidae (genus Bipes, three species, Baja California and mainland Mexico), and the Blanidae (genus Blanus, four species, Mediterranean region) [39,42]. Species in the Trogonophidae (4 genera, 6 species) are sand specialists found in the Middle East, North Africa, and the island of Socotra, while the largest and most diverse family, the Amphisbaenidae (∼185 sp.), is found on both sides of the Atlantic, in sub-Saharan Africa, South America and the Caribbean [39,42]. According to morphological data, the front-limbed Bipedidae is the sister-group to all other (limbless) amphisbaenians, which implies that loss of limbs occurred only once [39]. Given their peculiar habits, the distribution of amphisbaenians has been assumed to be primarily the result of two landmass fragmentation events: the split of the supercontinent Pangaea starting 200 million years ago (Myr), separating species on the northern landmass (Laurasia) from those on the southern landmass (Gondwana) and the subsequent split of South America from Africa 100 Myr [39,41,43,44].
2 The molecular novelties based on protein-coding nuclear genes
2.1 Squamate evolution
The first phylogenetic studies using a nuclear gene (C-mos) and comprehensive taxonomic coverage resolved the monophyly of most families but not interfamilial relationships [45–47]. In early 2004, the first study using multiple nuclear genes (C-mos and RAG1) and covering all major squamate lineages [48], discovered some unconventional relationships among squamates. It was shown with statistical support that snakes were not nested within anguimorphs. In addition, a close relationship was found between lacertid lizards and amphisbaenians. The classical association of snakes with either the varanid lizards or amphisbaenians was therefore rejected. Later in 2004, a second molecular study [49] reached similar conclusions with additional sequence data (C-mos and RAG1) and support. In both studies, the classical squamate dichotomy between Iguania and Scleroglossa was not supported. Instead, Iguania (one-fifth of all living species of squamates) was found to be a derived lineage, clustering high in the tree, together with snakes and anguimorphs. Nonetheless, several major nodes in both studies were weakly supported or unresolved. In 2005, a study using nine nuclear protein-coding genes (C-mos, RAG1, RAG2, R35, HOXA13, JUN, α-enolase, AMEL and MAFB) resolved with strong support all basal squamate splits but one [50] (Fig. 2). The limbless dibamids are the most basal lineage, followed by the Gekkota. The next higher-level group comprises xantusiids, cordylids, gerrhosaurids and scincids, with scincids in a basal position [48–51]. This group is the closest relative of a large clade which includes other lizards as well as amphisbaenians and snakes, and which is divided into two major groups. The first group comprises amphisbaenians, lacertids, teiids and gymnophthalmids; within this clade, the teiioids (teiids and gymnophtalmids) are the most basal lineage, and amphisbaenians and lacertids form a monophyletic group [48–50,52]. The second major group comprises iguanians, anguimorphs, and snakes. The presence of toxin secreting oral glands is a shared derived trait of this clade (∼4700 sp.), demonstrating a single early origin of the venom system in squamates, instead of two independent origins (one among caenophidian snakes and one among helodermatid anguimorphans) [52].
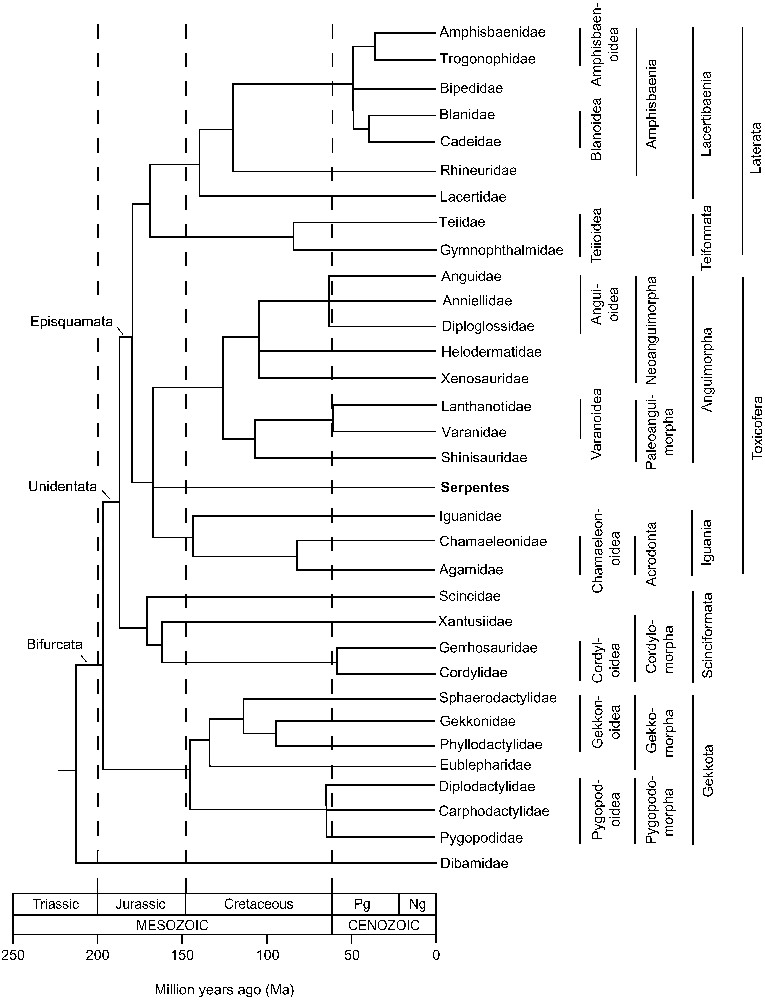
A time tree of squamates from Hedges and Vidal [60], based on time estimates from Vidal and Hedges [48], Wiens et al. [55], and Hugall et al. [56] with additional data from Vidal et al. [53] (amphisbaenians), Gamble et al. [54] (geckos), and Amer and Kumazawa [71] (iguanians). Pg, Paleogene; Ng, Neogene.
New morphological characters were identified that were consistent with the molecular phylogeny and used in the new classification [50]. Because all squamate families except Dibamidae have a bifurcated tongue, this large clade was named Bifurcata. The presence of one egg tooth (as opposed to two) defines the next most inclusive clade, Unidentata, which excludes dibamids and gekkotans. Scinciformata includes Scincidae, Xantusiidae, Gerrhosauridae, and Cordylidae. Teiformata includes the superfamily Teiioidea (Teiidae and Gymnophthalmidae). The venom clade [52] was named Toxicofera and the lacertid-amphisbaenian group was named Lacertibaenia. Lacertibaenians and teiformatans were grouped into Laterata, most of which have tile-like ventral scales. Toxicoferans and lateratans were grouped into Episquamata (“top squamates”).
However, molecular phylogenetic studies in recent years have resolved more of squamate phylogeny than these large clades. Detailed relationships of families are now well-supported [48–50,53–55]. Although they differ often in major ways from existing classification based on morphology, no effort has been made to adjust the remainder of squamate classification to reflect these new relationships. To facilitate further discussion of the evolution and biogeography of squamates, we make those additional adjustments in the classification here, focusing on nodes that have significant support in molecular analyses. Most of the changes are redefinitions of the content of existing taxa, although a few taxa are new, and noted as such.
Within Anguimorpha, the monophyly of the previously-defined Varanoidea (Varanidae, Lanthanotidae, and Helodermatidae) has not been supported by molecular evidence [48–50,55,56]. Also, the monophyly of Anguidae has been difficult to obtain because the anguid subfamily Diploglossinae has a similar level of molecular divergence as the family Anniellidae [49,55,57]. Therefore we restrict Anguidae to the subfamilies Anguinae and Gerrhonotinae, and recognize Diploglossidae as a family as has been done in the past [58]. Two clades of anguimorph families are defined that correspond to geography [48–50,55,56]. The first is a mostly New World (ancestrally North American) clade composed of Anguidae, Anniellidae, Diploglossidae, Helodermatidae, and Xenosauridae. The second is an Old World (ancestrally Asian) clade composed of Lanthanotidae, Shinisauridae, and Varanidae. These clades are so different from previous morphological groupings (e.g., Shinisaurus was usually placed in the Xenosauridae and helodermatids were usually associated with varanids and lanthanotids) that we give them new names: Neoanguimorpha for the New World clade and Paleoanguimorpha for the Old World clade. Within the Neoanguimorpha, the superfamily Anguioidea is here restricted to the three closely related families Anguidae, Anniellidae, and Diploglossidae, with the remaining families placed in their own superfamilies, Helodermatoidea (Helodermatidae) and one named here, Xenosauroidea (Xenosauridae). Within the Paleoanguimorpha, the superfamily Varanoidea is now restricted to the two closely related families Lanthanotidae and Varanidae, and Shinisauridae is placed in its own (new) superfamily, Shinisauroidea.
Within geckos (Gekkota), two recent molecular phylogenetic studies have recognized seven families and defined several well-supported groups [54,59]. For these we recognize the following taxa: Eublepharoidea (Eublepharidae), Gekkonoidea (Gekkonidae, Phyllodactylidae, and Sphaerodactylidae), and Pygopodoidea (Carphodactylidae, Diplodactylidae, and Pygopodidae), all pre-existing taxa. We place the first two superfamilies in the now redefined Gekkomorpha and the third in the new unranked taxon Pygopodomorpha. Considering the well-supported amphisbaenian relationships [53], we revise content for existing superfamilies Amphisbaenoidea (here Amphisbaenidae and Trogonophidae), Rhineuroidea (Rhineuridae) and erect the new superfamilies Bipedoidea (Bipedidae) and Blanoidea (Blanidae and Cadeidae). We place Rhineuroidea in a newly erected unranked taxon Rhineuriformata and place the other three superfamilies in another new unranked taxon Amphisbaeniformata. The scinciformatan families Cordylidae and Gerrhosauridae have always been found to be close relatives and are placed here in the existing superfamily Cordyloidea, and together with Xantusioidea (Xantusiidae) in the new unranked taxon Cordylomorpha. Scincomorpha is redefined to include only the Scincoidea with its single family, Scincidae. Finally, within Iguania, the Agamidae and Chamaeleonidae are placed in the Chamaeleonoidea, which in turn is placed in Acrodonta, all existing taxon names. Iguanidae is placed in Iguanoidea, which in turn is placed in the new taxon Neoiguania.
One of the most striking results of this new squamate phylogeny lies in the clustering of iguanians with anguimorphs and snakes. The monophyly of Scleroglossa is rejected, because iguanians are in a highly nested position among Squamata. The two lepidosaurian lineages using tongue prehension of food, the tuataras and the iguanians, have therefore acquired their feeding modes independently (Fig. 3). As the iguanians are the only squamate lineage using tongue prehension of food, and are highly nested within squamates, they have lost the jaw prehension trait used by all other squamate lineages, and have secondarily acquired their tongue prehension trait [49,50] (Fig. 3). In parallel, iguanians have switched from visual and vomeronasal prey discrimination to visual (only) prey discrimination (Fig. 3). Iguanians thus do not represent a primitive state of evolution in squamates but are a species-rich (ca. 1470 sp.) and specialized lineage combining lingual prehension, dependence on visual cues, and ambush foraging mode, and which feeds mainly on low energy and noxious prey avoided by other squamates such as ants, other hymenopterans, and beetles [20].
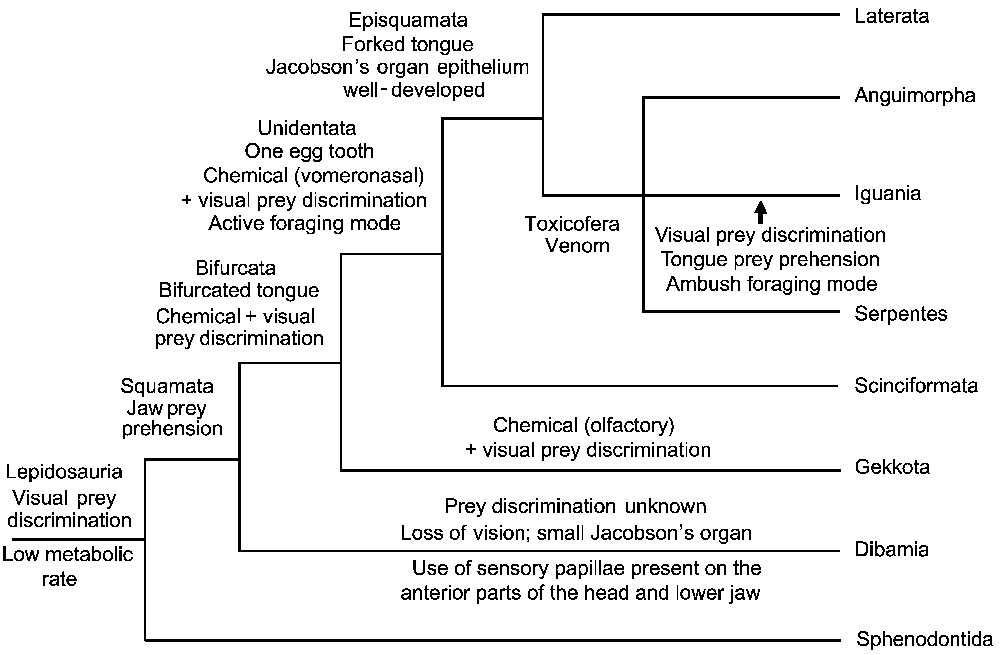
Evolution of prey discrimination and prehension in squamates based on Vidal and Hedges [48].
Three studies based on nuclear genes have estimated divergence times among the major lineages of squamates in a comprehensive manner [60]. The first study used nine nuclear protein coding genes and a Bayesian method [50]. The other two studies used RAG1 sequences and a penalized likelihood method [55,56]. Although estimates in the RAG1 studies are younger than those in the 9-gene study, all three studies show that most major groups diversified during the Jurassic and Cretaceous, 200–66 million years ago (Fig. 2) [60]. Moreover, according to the squamate timetree, one-third (ten) of all families of lizards and amphisbaenians diverged within a few million years of the Mesozoic/Cenozoic boundary (Fig. 2). This suggests a possible relationship with the asteroid impact at 66 million years ago and the resulting extinctions and ecological changes [60].
2.2 Snake evolution
Several higher-level snake phylogenies using nuclear genes (in addition or not to mitochondrial genes) have been published since 2002 [48,61–68]. They agree on the monophyly of alethinophidians and on the paraphyly of the macrostomatan condition. The fossorial small-gaped Aniliidae (South American genus Anilius) and the terrestrial large gaped (macrostomatan) Tropidophiidae (South American and West Indian genera Trachyboa and Tropidophis) cluster together, and form the most basal alethinophidian lineage (Fig. 4) [48,61,64,66,67]. The genus Anilius is therefore only distantly related to the remaining anilioids, which are all Asian and form a monophyletic group including Cylindrophiidae, Uropeltidae and Anomochilidae [69]. The alethinophidians were therefore primitively macrostomatan, and this condition was secondarily lost twice by Aniliidae and Asian anilioids, in connection with burrowing [61,64,67]. From a biogeographic point of view, the deep split between the Aniliidae/Tropidophiidae clade, which is of South American origin, and all remaining alethinophidians represents a vicariant event: the separation of South America from Africa in the mid-Cretaceous. Accordingly, those two clades were named Amerophidia and Afrophidia according to their geographic origin [67] (Fig. 4).
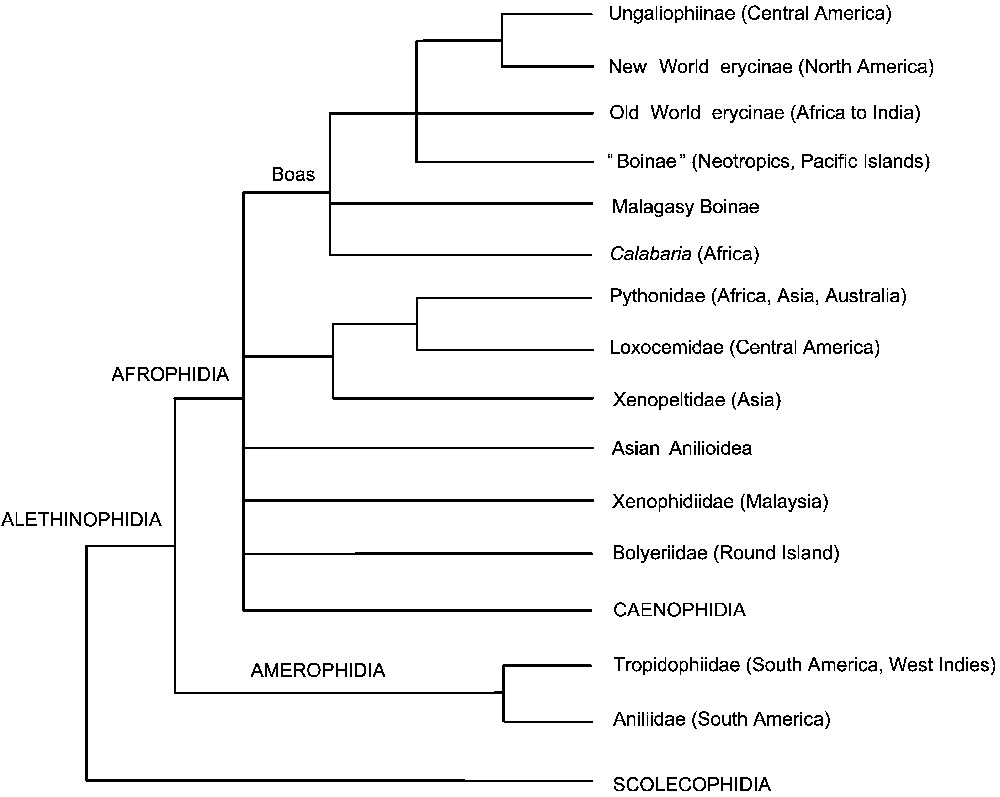
Higher-level phylogeny of snakes based on Vidal et al. [67], with additional data from Noonan and Chippindale [66] (boas). Boinae from the Neotropics are monophyletic while the phylogenetic position of the Pacific Island endemic genus Candoia remains uncertain.
Among alethinophidians, the monophyly of the group including the Pythonidae, the Xenopeltidae, and the Loxocemidae is found in most molecular studies [48,61,63,64,67], with Loxocemidae as the closest relative to Pythonidae. Another large group includes Calabaria, “boines”, “erycines”, and ungaliophiines (genera Ungaliophis and Exiliboa), with North American erycines and ungaliophiines as closest relatives [61,64,66,67].
Unfortunately, several higher-level alethinophidian relationships are still unresolved (Fig. 4), a situation contrasting with our state of knowledge of the interfamilial relationships among caenophidian snakes.
As recently as 2007, a study using seven nuclear protein-coding genes (C-mos, RAG1, RAG2, R35, HOXA13, JUN, and AMEL) resolved with strong support all major caenophidian splits [68] (Fig. 5). Caenophidians devoid of a front-fanged venom system were traditionally lumped into a huge (∼1900 sp.) family named “Colubridae”, including several subfamilies. As this family was shown to be paraphyletic, most of the subfamilies were elevated to a familial rank in order to reflect their evolutionary distinctiveness, and the name Colubridae was restricted to a monophyletic group [68] (Fig. 5).
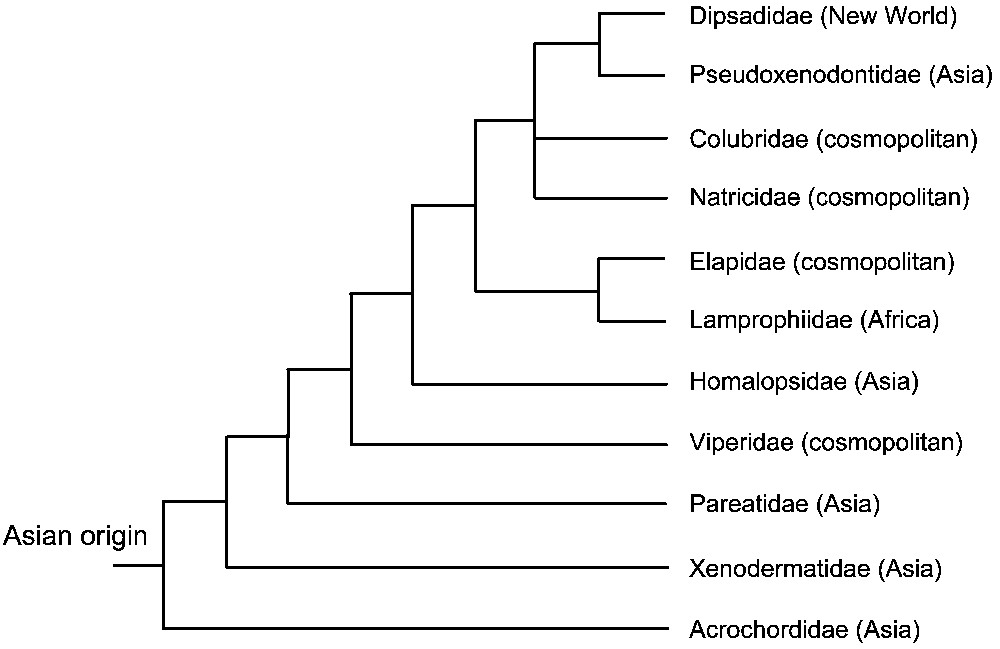
Higher-level phylogeny of caenophidian snakes based on Vidal et al. [68].
The caenophidian venom apparatus has experienced extensive evolutionary tinkering, with all variables (ranging from the biochemical variation and specialization of the venoms to the dentition and glandular morphology) changing independently, resulting in many kinds of toxins associated with diverse delivery systems [31,62,70]. Non front-fanged caenophidian snakes possess complex venoms containing multiple toxin types, while the front-fanged venom system appeared three times independently: once early in caenophidian evolution with viperids, once within atractaspidines (a lamprophiid subfamily), and once with elapids. Further a reduction in the size and complexity of the venom system is observed in species in which constriction has been secondarily evolved as the preferred method of prey capture (American ratsnake colubrids) or dietary preference has switched from live prey to eggs or to slugs/snails (colubrid genus Dasypeltis; elapid genera Aipysurus and Brachyurophis; all pareatids; some dipsadids) [31,62,70].
2.3 Amphisbaenian evolution
Only two molecular studies focusing on amphisbaenians and using nuclear genes have been published, one in 2004 using 2 genes (C-mos and RAG1) [42], and one in 2008 using 10 genes (C-mos, RAG1, RAG2, R35, HOXA13, JUN, AMEL, BDNF, NT3, and SIA) in addition to two mitochondrial ones (12S and 16S rRNA) [53]. Both studies confirmed the basal position of Rhineuridae, also obtained in higher-level squamate studies [48–50,52,55] (Fig. 6). This implies three independent events of limb loss among amphisbaenians, and further supports the widespread occurrence of reductions and losses of limbs among squamates [58]. Another striking result is the identification of a cryptic family of amphisbaenians endemic to Cuba, named Cadeidae [53], that is unrelated to the Amphisbaenidae, a family also present in the West Indies (Fig. 6). Amphisbaenians have therefore colonized the West Indies twice independently. Moreover, the New World (South America and West Indies) amphisbaenids form a clade nested within a paraphyletic group formed by African amphisbaenids and trogonophids [42,53] (Fig. 6). The interfamilial divergences among amphisbaenians have been dated using nuclear or mitochondrial genes and various calibration points [50,53,55]. All three studies show that with the exception of the basal split between Rhineuridae and the remaining Amphisbaenia estimated at 109 (154–76) Myr ago, all other interfamilial divergences took place in the Cenozoic, less than 66 Myr ago (Fig. 2). In particular, the split between African and South American amphisbaenids was estimated as 40 (54–29) Myr ago (Eocene) [53]. Because Africa broke from South America 100 Myr ago, and in the absence of any Laurasian fossil amphisbaenid [39], only transatlantic dispersal (Africa to South America) can explain this relatively recent divergence. Therefore, while amphisbaenians have always been considered as vertebrates with very limited dispersal abilities due to their burrowing lifestyles, oceanic dispersal played a significant role in their current distribution on both sides of the Atlantic Ocean. This result adds support to the growing evidence that oceanic dispersal should not be dismissed as a possible biogeographic mechanism for organisms that otherwise appear to be poorly adapted for an overseas journey [53].
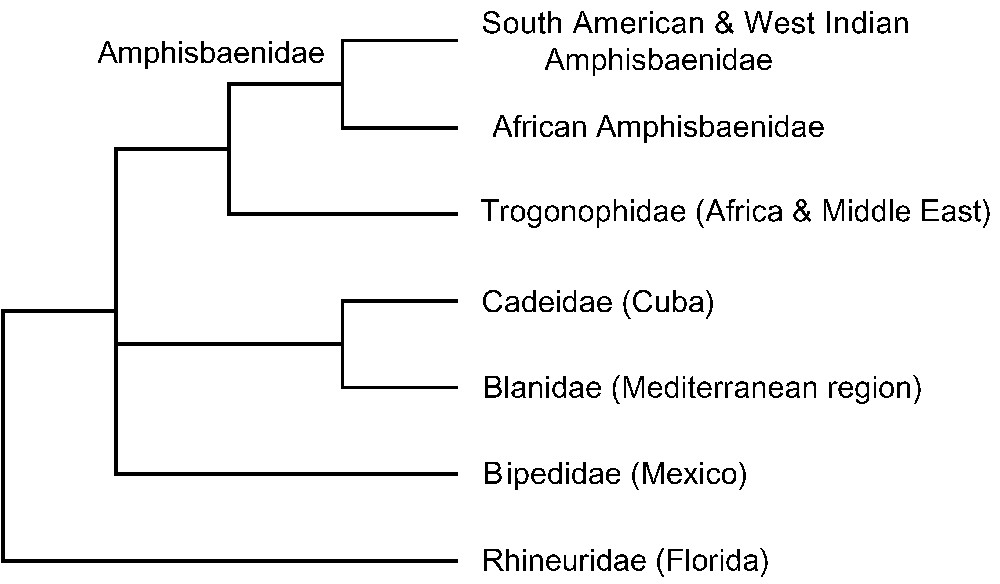
Higher-level phylogeny of amphisbaenians based on Vidal et al. [53], with additional data from Kearney and Stuart [42] (amphisbaenids). African amphisbaenids are paraphyletic.
Acknowledgements
We thank J.-C. Rage for help with paleontological issues, and R. Bour and P. David for help with taxonomic issues. This work was funded by the Service de Systématique moléculaire du Muséum National d'Histoire Naturelle to N.V. and by grants from the NASA Astrobiology Institute and National Science Foundation to S.B.H.