1 Introduction
Potato (Solanum tuberosum) is the world's fourth most important food crop after wheat, maize, and rice [1]. One of its more distinctive features is its tuber, which is a specialized storage organ that can accumulate large amounts of proteins, amounting to approximately 3–6% of the dry weight or 1–2% of the fresh weight of the potato tuber [2,3]. The proteins that accumulate in the tuber are also stable for several months, as demonstrated in studies of recombinant proteins in the tubers of recombinant plants [4,5]. These two properties have led researchers to use potato tubers as a plant host for the production of pharmaceutically and/or industrially valuable recombinant proteins [6–9].
The use of a strong constitutive promoter is critical to achieving increased expression levels of foreign genes in transgenic plants. The cauliflower mosaic virus (CaMV35S) [10], the opine synthase genes from Agrobacterium [11], Arabidopsis ubiquitin gene [12], the tobacco translation initiation factor 4A (NeIF-4A) promoter [13], the Arabidopsis actin gene [14], and the tobacco tCUP gene [15–17] are strong constitutive promoters that are widely used in dicot transgenic plants. In potato, the CaMV35S and patatin promoters are the most frequently used promoters in recombinant protein production systems, but the yield of recombinant proteins has not yet come close to approaching economically acceptable levels [5,6,18–20]. An alternative promoter, the granule-bound starch synthase (GBSS) promoter, has been shown to have an activity similar to that of the patatin promoter [21], and Hong et al. [22] reported that the sweetpotato sporamin promoter was highly active in different sized tubers of transgenic potato, with levels of phytase expression ranging from 3.8 to 7.4% of the total soluble proteins. These results clearly demonstrate that novel strong promoters need to be identified if the expression levels of recombinant proteins in transgenic potato tubers are to be further enhanced.
A different approach to overcoming the limited accumulation of foreign proteins in transgenic plants is the utilization of diverse signal peptides to direct the recombinant foreign proteins into cellular organelles such as plastids, including chloroplasts and amyloplasts [23–26], peroxisomes [27,28], and the endoplasmic reticulum (ER) [29]. Attempts to produce LDC (lysine decarboxylase) protein in transgenic tobacco plants [25] and tobacco hairy root cultures [26] revealed that LDC activity was higher when the protein was targeted into plastids with the aid of the rbcS transit peptide (TP). For storage organs like potato tubers, however, only a few case studies involving different TPs have been reported to date, including those on the signal sequence of proteinase inhibitor II (pinII) for ER and apoplast targeting [5,6], the granule bound starch synthase for chloroplast targeting [30] and the C-terminal KDEL sequence for ER targeting [19].
We previously cloned two ADP-glucose pyrophosphorylase (AGPase) small subunit genes from sweetpotato [31]. In a subsequent study, Kwak et al. [32] demonstrated that the promoter of ibAGP1 gene shows a strong constitutive activity in transgenic Arabidopsis and that utilization of its TP (plastid targeting peptide) enhances the accumulation of foreign protein. The activity of the ibAGP1 promoter in sweetpotato and carrot becomes stronger with increasing levels of endogenous sucrose. This is in agreement with the finding that the sucrose content in storage roots, such as that of sweetpotato and the tap root of carrot, is positively correlated with the growth of the storage roots, which is when they are actively accumulating large amounts of starch [33]. Since the potato tuber is also a storage organ that accumulates a large amount of starch, we have analyzed the activity of the sweetpotato ibAGP1 promoter in transgenic potato using the β-glucuronidase (GUS) gene as a reporter protein, with the aim of determining its potential for producing foreign proteins in plant production systems.
2 Materials and methods
2.1 Plant materials
Meristemic tissue of potato plants (Solanum tuberosum L. cv. Jowon) was initially cultured in liquid media containing 4.4 g L−1 Murashige and Skoog (MS) basic salts [34], 30 g L−1 sucrose, 0.1 mg L−1 gibberellic acid (GA3), and 0.1 mg L−1 kinetin and subsequently propagated in vitro on a solid media containing 0.8% phyto agar (Duchefa Biochemie, Haarlem, the Netherlands). The plants were sub-cultured at 4-week intervals for continuous propagation in vitro at 23 °C under a 16/8-h (light/dark) photoperiod with artificial light supplied at an intensity of 67.2 μmol m−2 s−1 as described by Goo et al. [35].
2.2 Plant transformation
Recombinant vectors of CaMV35S::GUS, ibAGP1::GUS, and ibAGP1::TP::GUS [23] were transformed into potato plants as described by Lee et al. [36] and Goo et al. [35]. Leaf explants () were co-cultured for 15 min with Agrobacterium tumefaciens GV3101 carrying each recombinant vector and 75.0 μM acetosyringone. A. tumefaciens was cultured for 36–48 h at 28 °C in YEP media (10.0 g L−1 peptone, 10 g L−1 yeast extract, and 5.0 g L−1 NaCl) containing kanamycin (50.0 mg L−1), gentamycin (15.0 mg L−1), and rifampicin (25.0 mg L−1), and the concentration was adjusted to prior to transformation. Co-cultured leaf explants were transferred to a regeneration media containing MS salts plus 30 g L−1 sucrose, 0.01 mg L−1 α-naphthalene acetic acid (NAA), 2.0 mg L−1 zeatin, 0.1 mg L−1 GA3, and 500 mg L−1 carbenicillin. Following approximately 8 weeks of regeneration, the induced shoots were transferred to a selection medium containing MS salts plus 30 g L−1 sucrose, 100 mg L−1 kanamycin, and 250 mg L−1 carbenicillin and cultured for a further 4 weeks. The regenerated plants were rooted in a rooting media containing MS salts plus 30 g L−1 sucrose, 250 mg L−1 carbenicillin, and 100 mg L−1 kanamycin and subsequently acclimatized to pots for further experiments as described by Kim et al. [37].
2.3 Purification of nucleic acid and northern blot hybridization
To quickly identify candidate transgenic plants, we purified DNA from selected shoots by the cetyltrimethyl ammonium bromide (CTAB) method [38] and conducted PCR analyses with a primer set designed for the GUS reporter gene (forward: 5′-GGGCAGGCCAGCGTATCG-3′; reverse: 5′-CCTTCACCCGGTTGCCAG-3′). For northern blot hybridization, total RNA was purified from the genomic PCR-confirmed transgenic plants as described by Goo et al. [35]. Three-week-old leaf tissue (0.1 g) and a harvested tuber (diameter: approx. 3.0 cm) from approximately 12 week old plants grown in pots were macerated with liquid N2 using a mortar and pestle. The powder was mixed with Trizol buffer (Invitrogen, Carlsbad, CA) and further purified according to the manufacturer's directions. Total RNA (20 μg) was fractionated by electrophoresis on a 1.5% formaldehyde agarose gel in 3-(N-morpholino) propanesulfonic acid (MOPS) buffer (pH 5.3). At completion of the electrophoresis, the agarose gel was stained with ethidium bromide, and the intensity of the staining of the rRNA was used as an indicator of the relative loading amount of total RNA. The fractionated RNA was then blotted onto a positively charged nylon membrane (Tropilon-plus; Tropix, Bedford, MA) by capillary transfer, UV-crosslinked at 1200 μJ cm−2, and hybridized with the GUS gene fragment amplified by PCR with the designated primer set as a probe. The probe fragment was labeled with α-[32P]dCTP using the HexaLabel DNA Labeling kit (Fermentas USA, Glen Burnie, MD) according to the manufacturer's directions. Following hybridization, the X-ray film was scanned, and the area value of each band was calculated using the Image J software program (http://rsb.info.nih.gov/ij/index.html).
2.4 Measurement of GUS activity and histochemical analyses
Tubers from 12- to 14-week-old plants grown in pots (diameter: 35 cm) were harvested and divided into six groups depending on their diameter [range in diameter: <2 mm (small developing tubers with minimal swelling at the stolon tips) to 60 mm]. To examine the effect of exogenously supplied sucrose, the internodes of the transgenic plants grown in vitro on solid media were cut, replanted on solid media either supplemented or not with 3.0% sucrose, and cultured for 14 days at 23 °C under a 16/8-h (light/dark) photoperiod (light intensity 67.2 μmol m−2 s−1).
GUS enzyme activity was quantitatively measured using the method of Jefferson et al. [39] and Jefferson and Wilson [40] with minor modifications, as described by Kwak et al. [33]. The harvested tuber tissue (1.0 g), leaf tissue (0.5 g), and the entire upper part of transgenic plants grown in vitro without or with 3.0% sucrose were macerated in liquid nitrogen using a mortar and pestle. The powder was mixed with extraction buffer (50 mM phosphate buffer, pH 7.2, 10 mM EDTA, 0.1% Triton X-100, 0.1% Sarcosyl, and 10 mM β-mercaptoethanol), and the extracts were centrifuged for 10 min at 12,000 rpm. The amount of total protein in the supernatant was then quantified at 595 nm on a UV-spectrophotometer (UV-1240, Shimadzu, Kyoto, Japan).
An aliquot (50.0 μg of total protein) of each sample was used to react with 1 mM of 4-methylumbelliferyl-β-D-glucuronide (MUG) in extraction buffer at 37 °C for 30 min to 1 h. The reaction was terminated by adding 0.2 M Na2CO3, and the fluorescence was measured at 365 nm on a fluorescence spectrophotometer (Wallac Victor 1420; Perkin Elmer, Wellesley, MA). The total soluble protein content of the extracts was determined using a spectrophotometer (UV-1240; Shimadzu) at 595 nm, as previously described [33]. Three individual transgenic plants were propagated in vitro and assayed for GUS activity, and values are given as the means ± standard deviation. Each plant tissue sample excised from transgenic potato plants was stained for histochemical analyses using the methods of Jefferson et al. [39] and Kwak et al. [33]. Samples were incubated overnight at 37 °C in 100 mM sodium phosphate buffer (pH 7.0) containing 20 mM 5-bromo-4 chloro-3 indolyl-β-D-glucuronide (X-gluc), 0.5 mM potassium ferrocyanide, 0.5 mM potassium ferricyanide, 10 mM EDTA, and 0.1% Triton X-100 (v/v). The samples were then thoroughly washed with 70% ethanol to clear chlorophyll from the tissues prior to being photographed.
3 Results
In a practical extension of our continuing research on the application of the ibAGP1 promoter isolated from sweetpotato in promoting the mass production of foreign proteins in transgenic storage root crops, we have analyzed the activity of this ibAGP1 promoter and the effect of ibAGP1 TP in transgenic potato. We consequently compared the activity of the CaMV35S promoter, a well-known and frequently used constitutive promoter in plant transformation systems with that of the ibAGP1 promoter. Several transgenic potato plants were obtained following transformation with three recombinant vectors – CaMV35S::GUS, ibAGP1::GUS, and ibAGP1::TP::GUS. As summarized in Table 1, more than 28 individual transgenic plants from each recombinant vector were initially selected by PCR analyses and GUS staining of the primary regenerated shoots.
Summary of transgenic potatoes. Leaf explants (5 × 5 mm) were co-cultured with Agrobacterium tumefaciens bearing each recombinant vector and regenerated as described in Section 2.2. The efficiency of transformation represents the ratio for the GUS-stained plants out of total number of explants.
Vector | Explants No. (A) | Regenerated plants | Survived in kanamycin | GUS stained plants (B) | Efficiency (%) (B/A × 100) |
CaMV35S::GUS | 256 | 80 | 29 | 28 | 10.9 |
ibAGP1::GUS | 255 | 117 | 58 | 42 | 16.5 |
ibAGP1::TP::GUS | 259 | 81 | 42 | 38 | 14.6 |
3.1 GUS transcript levels in transgenic potato lines
Three individual transgenic lines from each recombinant vector were randomly chosen, and their transgenic nature was further confirmed by northern blot hybridization (Fig. 1). In general, transgenic lines with the CaMV35S::GUS construct accumulated a much higher level of the GUS transcript than those with either of the other two constructs. The highest levels of GUS transcript were detected in the leaves of CaMV35S::GUS-lines, being at least twofold higher than those found in the tubers of the same lines. A number of the transgenic lines with the ibAGP1::GUS or ibAGP1::TP::GUS recombinant vector (17, 33, and 1) had higher transcript levels in the tubers than in the leaves, but the difference was not as great as that found in the CaMV35S::GUS-transgenic plants. In contrast, only trace amounts of transcript were observed in both leaf and tuber tissues of line no. 10 transformed with ibAGP1::GUS construct.
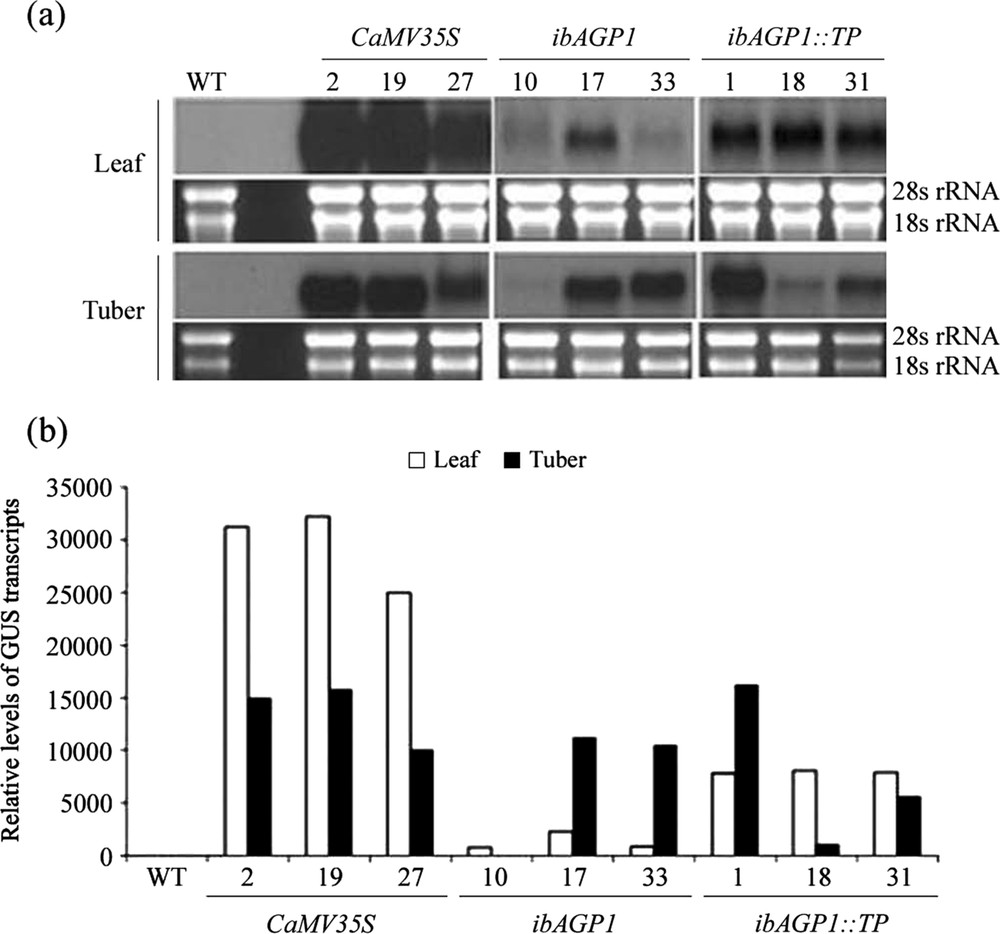
Northern blot hybridization of transgenic potato plants. (a) Aliquots (20 μg) of total RNA extracted from wild-type (WT; non-transgenic Solanum tuberosum cv. Jowon) and transgenic plants bearing CaMV35S::GUS (2, 19 and 27), ibAGP1::GUS (10, 17 and 33), and ibAGP1::TP::GUS (1, 18 and 31) constructs were fractionated on a 1.5% formaldehyde agarose gel and stained with ethidium bromide as a control for the loaded amounts of total RNA by using the intensities of the two rRNA bands (28s and 18s rRNA). The fractionated RNA was transferred onto a positively charged nylon membrane and hybridized with α-[32P] dCTP-labeled β-glucuronidase (GUS) fragment as a probe. (b) The film was scanned to obtain the relative area of each band by using the Image J software program (http://rsb.info.nih.gov/ij/index.html) and converted as the relative level of GUS transcript. The white and black histogram indicates the leaf and tuber, respectively.
3.2 GUS activity levels in transgenic potatoes
GUS enzyme activity was measured (MUG assay) to examine the tissue-specificity of the ibAGP1 promoter and the enhanced ability of its TP to accumulate foreign protein. As shown in Fig. 2a, in leaf tissues, GUS activity in the ibAGP1::GUS-lines was similar to that in the CaMV35S::GUS-lines, but it was increased – as much as 7.5-fold – in the ibAGP1::TP::GUS-lines, indicating that the TP sequence of ibAGP1 was able to enhance the accumulation of foreign protein even though its transcription level was relatively low (Fig. 1). In contrast, in tuber tissues, the ibAGP1 promoter alone was able to direct a significant increase in GUS activity, with the GUS activity of lines 17 and 33 increasing by as much as approximately 15.6-fold relative to that of the CaMV35S::GUS-line. In addition, the TP in the ibAGP1::TP::GUS-lines directed an enhanced expression of GUS activity that was up to twofold higher than that of the ibAGP1::GUS-lines (Fig. 2a). Ultimately, the ibAGP1::TP::GUS-lines showed a 26-fold increase in GUS activity in the tuber compared with that in the CaMV35S::GUS-line. The results of the histochemical analyses with X-gluc (fluorescent assay) also verified that the ibAGP1 promoter and its TP increased the accumulation of GUS (Fig. 2b): in the ibAGP1::GUS- or ibAGP1::TP::GUS-lines, there was GUS staining of the entire tissue sample, while in the CaMV35S::GUS-lines, the staining pattern was restricted to a certain area of the tissue sample.
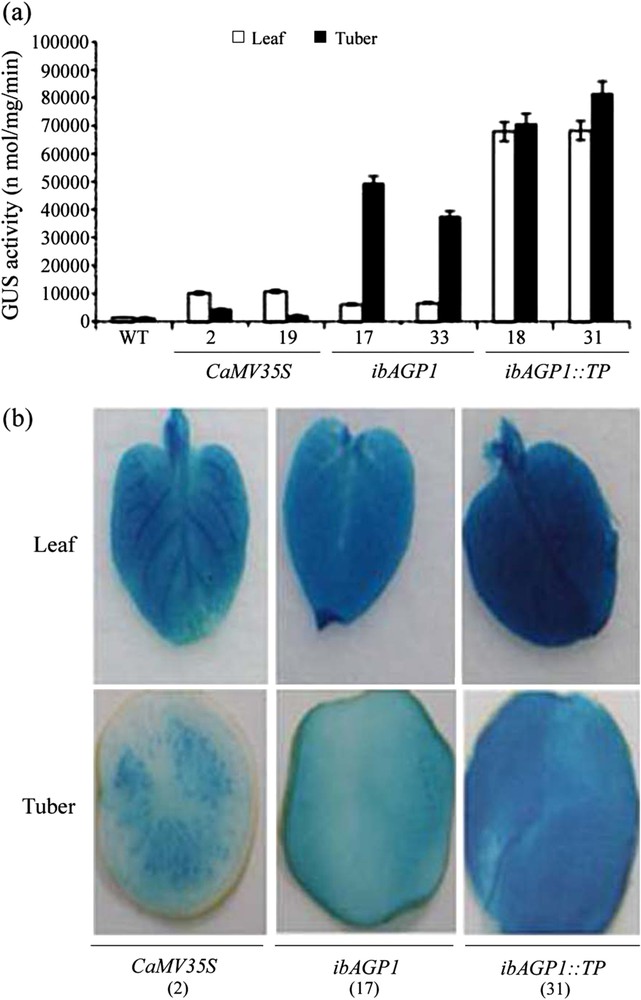
Activity of the ibAGP1 promoter and its transit peptide in the leaf and tuber of transgenic potato plant. The leaf tissue was harvested from potato plants grown for 2 weeks in pots, and tubers (diameter 3.0 cm) were harvested from potato plants grown for 12 weeks in pots. The total soluble protein was extracted from each sample. (a) GUS activity was measured with the fluorescence spectrophotometer after an aliquot of 50.0 μg of total protein was reacted with MUG as a substrate. The results are expressed as means ± standard deviation (SD) of independent experiments (n = 3) from three individual transgenic plants. WT represents non-transgenic Solanum tuberosum cv. Jowon and numbers indicate transgenic plants for each labeled constructs. (b) Both leaf and tuber tissues from transgenic plants bearing CaMV35S::GUS (CaMV35S), ibAGP1::GUS (ibAGP1), ibAGP1::TP::GUS (ibAGP1::TP::GUS) constructs were stained with X-gluc, as described in Section 2.
3.3 Effect of exogenous sucrose on the activity of the ibAGP1 promoter
As the activity of ibAGP1 promoter has been shown to be sucrose inducible in Arabidopsis, sweetpotato, and carrot, we also examined the induction activity of an exogenously supplied high concentration of sucrose on the ibAGP1 promoter and its TP in in vitro-propagated transgenic potato tissues. As depicted in Fig. 3, GUS activity was clearly elevated by exogenously supplied sucrose – up to more than 1.5-fold in both the ibAGP1::GUS- and ibAGP1::TP::GUS-transgenic lines. GUS activity was relatively very high in in vitro cultured tissues of ibAGP1::TP::GUS-transgenic lines without sucrose, and exogenously supplied sucrose further enhanced GUS activity by up to nine-fold in comparison to that of the ibAGP1::GUS-transgenic lines.

The effect of exogenous sucrose on the ibAGP1 promoter and TP in transgenic potato plants. Transgenic plants were sub-cultured on MS media either without or with sucrose (3.0%) for 2 weeks. The entire upper part of the plant (leaf and stem) was then ground in a mortar and pestle with extraction buffer. The aliquot (50.0 μg) of total protein was used for the GUS activity assay. GUS activity was measured on a fluorescence spectrophotometer after reacting with MUG as a substrate. The results are expressed as means ± SD of independent experiments (n = 3) from three individual transgenic plants. WT represents non-transgenic Solanum tuberosum cv. Jowon and numbers indicate transgenic plants transformed with CaMV35S::GUS (CaMV35S), ibAGP1::GUS (ibAGP1), and ibAGP1::TP::GUS (ibAGP1::GUS).
3.4 Activity of the ibAGP1 promoter at various developmental stages
During the development of tubers, the endogenous sucrose concentration is elevated to enable active starch accumulation. To study GUS activity in more detail, we therefore examined GUS activity at different developmental stages (based on tuber diameter) of the transgenic tubers. The results of this analysis are shown in Fig. 4. GUS activity was higher (4.1-fold) in 4-mm-diameter tubers of ibAGP1::GUS-transgenic plants (very young developmental stage) than in 2-mm-diameter tubers carrying the same construct. After a diameter of 4 mm had been reached, GUS activity gradually increased until it reached a maximum activity (up to 6.9-fold) at a tuber diameter of 35.0 mm, following which it decreased until the fully developed tuber size was reached (60.0 mm).
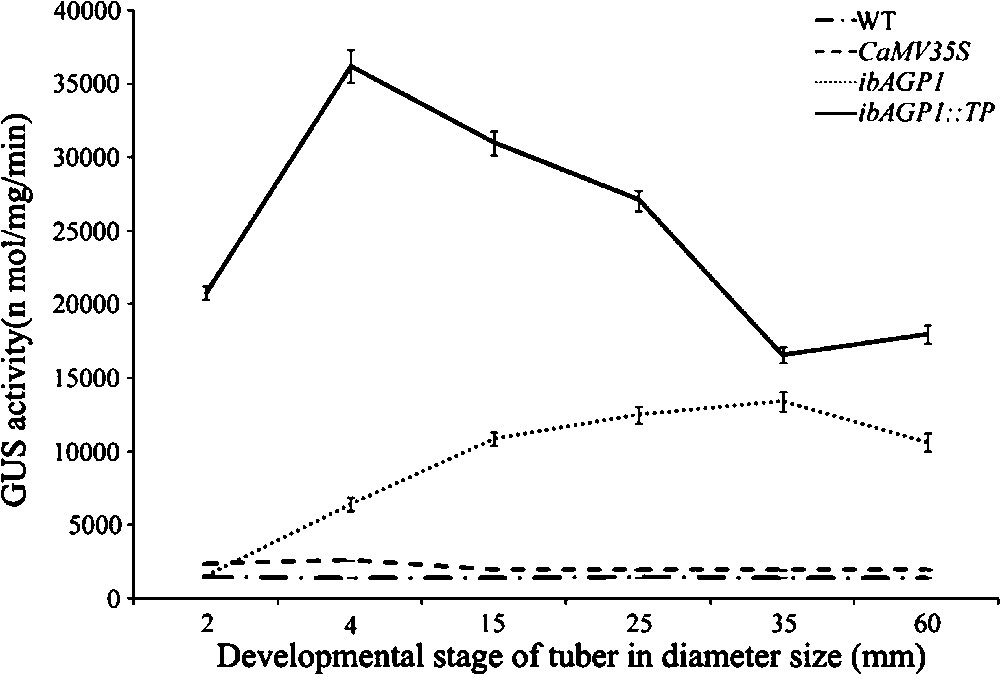
Activities of the ibAGP1 promoter and its TP during different developmental stages of tubers in transgenic potato plants. Tubers were harvested from plants grown for 12 weeks in pots and classified into six groups depending on their developmental stage. GUS activity was measured as described in Section 2. Means from three tubers of three different individual transgenic plants for each recombinant constructs [CaMV35S::GUS (CaMV35S), ibAGP1::GUS (ibAGP1) and ibAGP1::TP::GUS (ibAGP1::TP)] are given. Values shown are the mean of three replicates (SE < 10% of the mean). WT represents non-transgenic Solanum tuberosum cv. Jowon.
In the case of ibAGP1::TP::GUS-lines, however, GUS activity was very high even in the earliest stage of tuber development (2 mm in diameter), with these youngest tubers having an 8.7-fold higher GUS activity than the 2-mm-diameter tubers of the ibAGP1::GUS-lines. This activity increased further – up to 13.9-fold in the 4-mm-diameter tuber compared to that of similarly sized tubers of the ibAGP1::GUS-line – and then decreased until the tubers were 60 mm thick, although the latter still retained a higher level of GUS activity than that found in their similarly sized counterparts of the ibAGP1::GUS-lines.
4 Discussion
The availability of a strong constitutive promoter is critical in any “molecular farming” biotransformation system that aims at maximizing the expression levels of foreign genes in transgenic plants. Several promoters have been used for the large-scale production of foreign proteins in potato tubers. Chong and Langridge [18] produced human lactoferrin (hLF) with an enhanced version of the CaMV35S and auxin-inducible manopine synthase (mas) P2 promoters in transgenic potato tubers. In their system, the lactoferrin level produced by the auxin-induced mas P2 promoter was approximately tenfold higher than that expressed by the enhanced CaMV35S promoter. Park and Cheong [7] attempted to express recombinant human interleukin-2 (rhIL-2) in transgenic potato tubers and found that the biological activity of the RhIL-2 generated under the control of the patatin promoter was approximately twofold higher than that generated under the control of the CaMV35S promoter. These studies clearly demonstrate that more diverse promoters are needed to obtain strong and/or optimum gene expression in potato tubers.
To evaluate the potential of the ibAGP1 promoter as a strong promoter for the transgenesis of potato plants, we analyzed the activity of ibAGP1 promoter in both leaf and tuber tissue of transgenic potato plants. In growing tubers, the ibAGP1 promoter directed an enhanced and strong GUS expression in the ibAGP1::GUS-transgenic lines (15.6-fold) in comparison to that in the tubers of the CaMV35S::GUS-transgenic lines (Figs. 1 and 2). This result indicates that the activity of ibAGP1 promoter was much stronger than that of CaMV35S promoter in the potato tuber possibly due to a higher translational effect (level of protein per unit of mRNA) since the relative levels of GUS transcripts were not significantly different between the transgenic lines carrying either the CaMV35S::GUS or the ibAGP1::GUS construct. In fact, the transgenic lines carrying the CaMV35S::GUS actually accumulated a higher amount of GUS transcript than those carrying the ibAGP1::GUS construct.
This higher activity of the ibAGP1 promoter in the potato tuber may be attributable to the sucrose-inducible nature of the ibAGP1 promoter. Kwak et al. [32] reported that the activity of the ibAGP1 promoter increased significantly with increasing endogenous sucrose content in the storage roots of sweetpotato and taproots of carrot and that it was elevated in Arabidopsis leaves following exogenous treatment with sucrose. We also observed that ibAGP1 promoter activity in in vitro-cultured transgenic potatoes increased (up to more than 1.5-fold) following treatment with exogenously supplied sucrose (Fig. 3), suggesting that the sucrose inducibility of the ibAGP1 promoter is well conserved in potato tubers. This result is also supported by the observation that GUS activity in ibAGP1::GUS-transgenic lines increased gradually with increasing diameter of the tubers (Fig. 4). Although we did not measure the sucrose content in the potato tubers of different developmental stages, Viola et al. [41] reported that sucrose content begins to increase at a very early developmental stage – when the swelling stolen is less than 2–5 mm in diameter – and further increases by as much as four- to eightfold in 10-mm swollen tubers. Roessner-Tunali et al. [42] reported that the sucrose concentration in a growing tuber is maintained at a level about fivefold higher than that found in leaves. In contrast, the sucrose concentration was found to gradually decrease in fully grown tubers, when sucrose was not being transferred from the source tissue, i.e. the leaves of the plant [43,44]. This trend was even more evident when the tubers were detached from the plants; there was up to a 70% drop in the sucrose level within 3 days [45]. These results are consistent with our observations of decreasing ibAGP1 promoter activity at a later stage of tuber development (diameter: 60 mm) in a transgenic potato line. Previous results also suggest that a high sucrose concentration plays a pivotal role during tuberization in general and at the onset of tuberization in particular, when it functions as a trigger for the transition from stolon to tuber [46–48]. High sucrose concentrations are important contributory factors to the active and rapid starch accumulation process that occurs in young developing tubers as well as to the induction of genes involved in tuber metabolism [49,50]. Although several attempts have been made to up-regulate or down-regulate the genes involved in sucrose and starch metabolism, with the aim of exploring the exact role of sucrose in tuberization, the process remains largely unknown [51–55].
We also observed that GUS activity was further enhanced in ibAGP1::TP::GUS-lines when GUS protein was targeted to plastids (amyloplasts in potato tuber) using the TP sequence of ibAGP1. The enhancing effect of the ibAGP1 TP may be derived from the targeting properties of the TP itself or from a specific interaction between the ibAGP1 promoter and the TP. Further studies need to be carried out to determine the mechanism underlying the enhancing effect of the TP in our system. TPs have also been found to facilitate increases in the accumulation of foreign protein in transgenic tobacco [25] and tobacco hairy root cultures [26] expressing lysine decarboxylase (ldc). In both cases, LDC activity was higher when the ldc-coding region was fused to the rbcS TP in the transgenic tobacco plants and hairy root cultures [25,26]. Bae et al. [56] recently reported that the simultaneous targeting of a fungal xylanase to both chloroplasts and peroxisomes resulted in the amount of accumulated xylanase increasing to 160% of that when only chloroplasts were targeted and 240% of that when only peroxisomes were targeted [56]. The results obtained in our study, however, indicate that GUS activity in the ibAGP1::TP::GUS-transgenic lines was elevated only in tubers up to 4 mm in diameter, with GUS activity declining gradually in larger tubers as they thickened. Given our observation that the activity of the ibAGP1 promoter increased with increasing potato tuber size, the decrease in GUS activity in tubers larger than 4 mm may be attributable to the limited effect of the TP in enhancing GUS protein accumulation during the later stages of tuber development – and not be related to ibAGP1 promoter activity at all. However, very high levels of GUS activity still remained during the later stages of tuber development since the TP sequence of ibAGP1 was able to direct the GUS foreign protein into the chloroplast. We did not examine the quantity of additional foreign protein that can be accumulated in the plastids of transgenic potato tubers or how this quantity would be affected by the developmental stage of the growing tubers. Nevertheless, our preliminary results suggest that the TP sequence of the ibAGP1 gene promoted an increase in GUS activity of between 1.7- and 5.7-fold in comparison to that found in the ibAGP1::GUS-transgenic lines.
The sucrose-inducibility of the ibAGP1 promoter may be an additional benefit since transgenic potato can be easily propagated in an in vitro system [57,58], and mini-tubers can be easily induced in a controlled system [59–61]. Indeed, results from earlier studies suggest that in vitro tuberization does not require any other growth regulators but, rather, relies on the presence of a high sucrose concentration in the culture media [62]. Moreover, cell suspension culture systems for potato are well established [63–65]. Since the ibAGP1 gene is known to be expressed in both photosynthetic (source) and non-photosynthetic (storage) tissue and is remarkably up-regulated by sucrose content in the medium [32,66], we support the view that targeted foreign proteins can be massively accumulated in in vitro suspension cell cultures, tissues, and mini-tubers simply by adding sucrose to the culture medium.
In conclusion, these preliminary results suggest that the ibAGP1 promoter and its TP have the potential to be a strong foreign gene expression system with promising applications for molecular farming in transgenic potato.
Acknowledgements
This work was supported by a grant (code 20070301034017) from the BioGreen 21 Program, Rural Development Administration, Republic of Korea. Shin-Woo Lee was also supported for a sabbatical year by JinJu National University. We are grateful to Ms. Ann Jenks for her critical proof reading on the manuscript.