1 Introduction
The history of mountain vegetation cover is often, by default, reduced to that of high-mountain flora, “alpine flowers”. The main objective of the present Note is to expand resolutely this question on the following three axes:
- – Orophile plants refer to plants which have adapted to mountain conditions in the broadest sense, including all biological levels and not just that of high mountains above the upper limit of forests. The determination of the lower limit of the orobiome, i.e., mountain limit in relation to hills or plains, has formerly been discussed [1], and it has been proposed that an altitude of 500 metres be maintained as a rough estimate for Mid-Europe (the Alps, the Carpathians, etc.). The term alpine is known to have two different meanings: (a) that which characterises the high mountains referring to different ranges, we therefore talk of the alpine belt or alpine flora; and (b) that which refers to the Alps range itself, the terms alpien or alpic have therefore been proposed. However, in this work the term alpine is used in both senses and, in general, the context helps clear any ambiguity. This term is used here to refer to the entire alpine arc including all of the Western Alps [2,3].
- – Plant population should be understood both as flora and ecosystems; however, the two histories must be separated since the time scale is completely different. Hundreds of thousands of years are generally required to form a species and millions of years for the individualisation of a genus. However, a forest, with its structure and soil, may be formed in several millenniums. Of course, the reforestation of the Alps is recent (postglacial); nevertheless recent or current ecosystems were only able to establish themselves since there already existed a diversified flora, part of which is Pliocene.
- – The term Genesis is used here to cover at once the geographic origins of orophile species, the mechanisms of their differentiation and that of the setting up of plant communities (biocoenoses and ecosystems), etc. The plant population of a range requires the adaptation, via uplift, of pre-existing plain species, but also migration from other mountains of pre-adapted species. Physiological, cytological or genetic adaptations, already well studied, have now been renewed with the introduction of molecular biology. However, questions relating to the geographical origin of species, their migration and the setting up of plant communities are sometimes pushed to the background. The present article favours a geobotanical and ecological approach, which is often neglected nowadays.
2 Is the history of the vegetation of the Alps to be remade?
2.1 Traditional points of view
The first major geobotanists to study the Alps [4,5] maintained a simple schema comprising three periods: (a) first of all, the differentiation of an initial flora from the warm-climate flora occupying Central Europe during the Miocene epoch, which Diels [6] named arcto-tertiary flora; to this would be added a significant contingent from the Asian ranges, including in particular Saxifraga, Primula, Gentiana, and Pedicularis, the plant cover of the Alps being therefore formed in part by “impoverished stolons” (kümmerliche Ausläufer) of flora from central or western Asia; (b) the second period is that of glaciations, which destroyed this thermophilous flora, forcing them to seek shelter and introducing exogenous cold-climate flora; (c) the final stage is postglacial warming, including reforestation from shelters and arctic-alpine disjunctions.
This schema has been outdated for a long time now. The commonly, now accepted, approach, which has been summarised in Fig. 1, takes into account major events in the range's geological history and climatic evolution, and includes, between the first thermophilous and the last glacial flora, the vital role of a flora developed essentially during the Pliocene epoch.
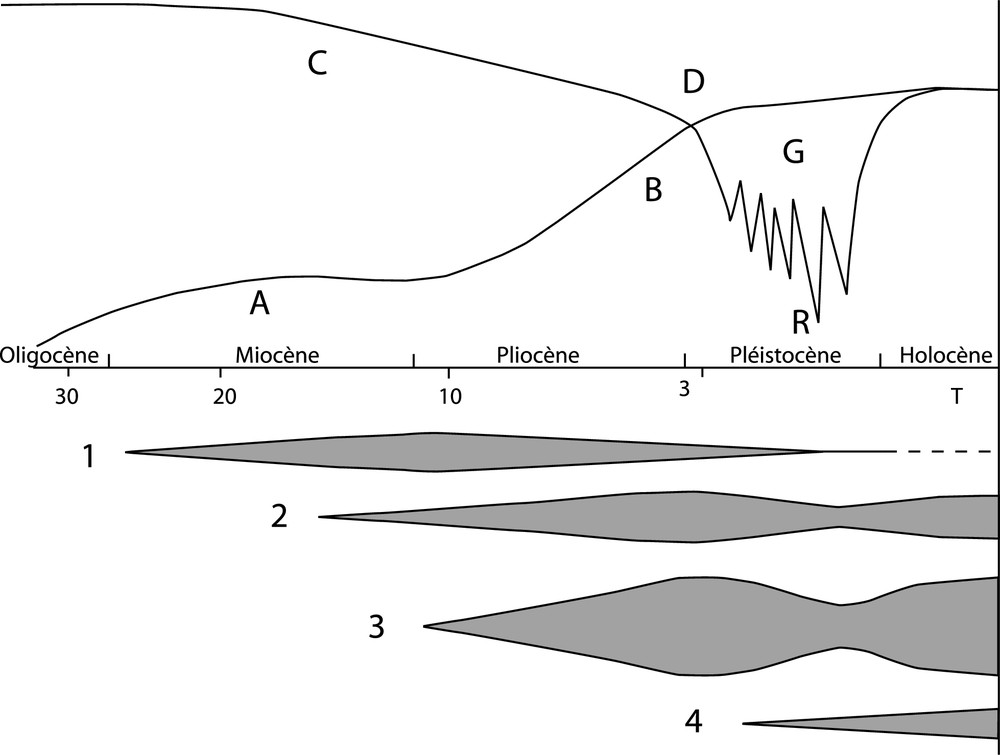
Attempt at a representation of the formation of the plant population in the Alps. Timescale (or geologic periods, and dating in million years BP) were dilated from left to right. AB: Surrection of the range; CD: Progressive cooling of approximatively 20 to 10 °C for Central Europe; G: Glaciations, which the last but one, called Riss, would have been the coldest. 1 to 4: The successive plant populations (the migrations from remote origins are not represented here). 1: Arctic-tertiary vegetation of subtropical origin, decreasing then by the competition of the later vegetation and almost completely destroyed by the glaciations. 2: Mesogean plant population. 3: Plant population of medio-European origin, the most important. 4: Arctic alpine contribution, appeared during interglacial period.
(a) With respect to the formation of the alpine range, uplift is difficult to date since, due to the structural complexity of the Alps, the different massifs were formed at very different times and interpretations regarding each of the massifs can differ from one geologist to another. Following a long tectonic preparation phase during the Cretaceous–Oligocene epoch, Mittelgebirge type reliefs (Raxlandschaft) [7] were first of all formed (Middle Miocene epoch?) on a peneplain surviving in the place of old eroded hercynian chains, where the first orophytes would have been mid-mountain plants [8]. The uplift of the high range is more recent, this continued during the Pliocene epoch and only reached its current state during the Pleistocene epoch.
(b) It is widely accepted that the climate of Central Europe, primarily subtropical, has got gradually colder since the Middle Miocene and, in particular, during the Pliocene epoch; the annual average temperature has fallen from 20 to 10 °C (which is approximately the current stand, following significant and sudden oscillations during the Glacial Age). This gradual cooling is due, at least in part, to astronomical causes; however, it is also nowadays related to a drift towards the north of the plate on which the alpine range is located, what has been confirmed by paleomagnetic studies.
(c) The majority of orophytes in the alpine range most probably date from the Pliocene epoch, as is also the case for other large Mid-European ranges (the Pyrenees, the Carpathians, the Balkans). This contribution may be divided into biogeographical contingents, which we will come back to (cf. Section 3.1). The formation of these contingents, which are largely dominated in the range's current flora, is issued from a temperate flora which is similar to that which currently covers southern and Central Europe. Surprisingly, even recent works [9,10] still attribute this Pliocene flora to descendants, in part of Tertiary flora and mainly of remote contributions (Mediterranean and Asian mountains, arctic regions), leaving little place for the simple theory of a direct origin (cf. see Section 3.1 below).
2.2 In search of new perspectives
These traditional views on the origin of the vegetation of the Alps call for more in-depth analysis. However to “review” does not necessarily mean the mere introduction of new information. This could also mean, in addition to new facts, the representation of traditional ones, of which previous works simply provided a partial overview.
(a) Taking into account all of the mid-latitude mountains in Europe (from the Pyrenees to the Carpathians and northern Balkans), it would appear that the Alpine arc is merely at the centre of a system (Fig. 2), which I have already proposed to call the generalised alpine System [2] or the Mid-European Orosystem [11]. The unity and originality of this system is apparent in its flora (its area clearly coincides with that of Abies alba, Quercus from the pubescens group [12] and Rhododendron ferrugineum, among others), or in its significant endemism. The most recent and best illustration of this unity was provided, with the same outline, in Flora Alpina [13]. Biocoenotics also show the unity of the system, in terms of the ecology of beech forests [14] as well as in the phytosociological composition of the Alpine belt [15].
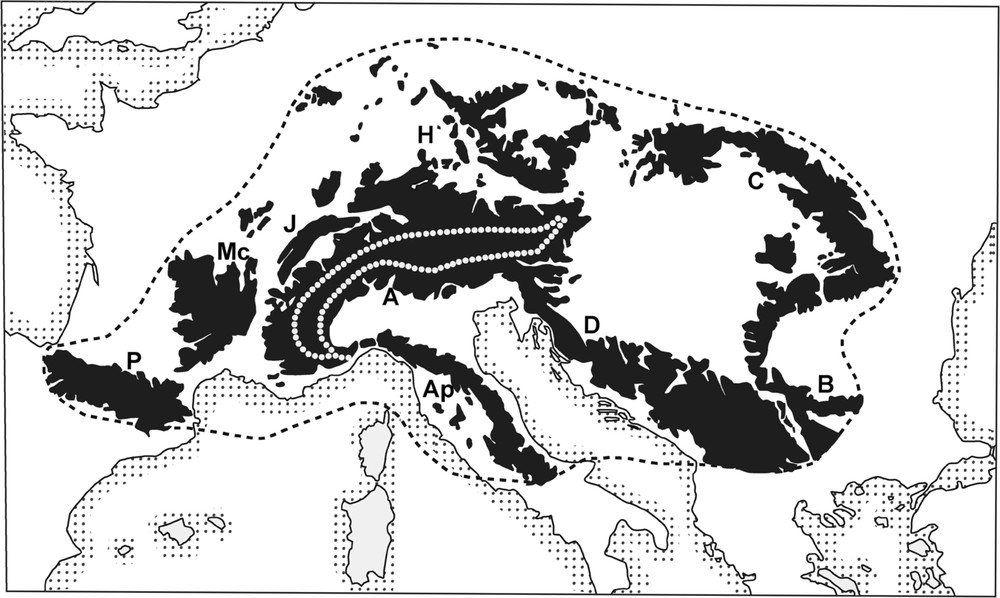
The alpine system (sensu P. Ozenda). In black, territory situated over 500 meters. A: The Alps (the intra-alpine axis is surrounded with a dotted line); M: French Massif Central, J: Jura, Ap: Northern Apennines, D: Dinarides, C: Carpathian Mountains, B: Bulgaria, H: Hercynian Massifs.
It therefore seems logical, and it would be fertile, to replace the history of the population of the Alps with that of the entire system and even that of the continent as a whole. Moreover, even within the alpine range itself, the latest contributions from the study of the Western Alps must be integrated into an approach that for a long time has been based on that of the Central and Eastern Alps and, in particular, that of the Swiss Alps.
(b) There is still much work to be done to link the history of plants with recent developments in the geology of the Alps and, more generally, that of southern Europe. In particular, the impact of glaciations on plants is much more complex than the traditional viewpoint would suggest. It includes not only a series of phases involving to-and-fro succession, but also the underestimated role of interglacial periods that no longer appear as mere remissions, but as active periods in the formation of the plant cover. The study of the Late-Glacial and Holocene epochs, which have been the focus for the majority of research works, is certainly instrumental since it represents the most significant part of the setting up of the current flora and ecosystems, and, since it is the most recent period, it is also the easiest to study. However, this was not one single point within the history of the range; rather it is a repetition of what occurred at the start of each interglacial period.
(c) The population of a mountain results from two major elements: an autochthon core, formed by the plants already in place during the uplift, and migrations from other mountains. Their relevant parts obviously depend on the degree of isolation of the range in question, but on many other factors also. Tolmatchev [16] considered that the primary nucleus (autochthonous part) was predominant and that the part resulting from migration was generally overestimated. This is indeed the case in a large number of chains in central Asia, in particular the most isolated (“Model I” from Agakhanyanz and Breckle) [17]. This is also the case for many Mediterranean mountains such as the Atlas Mountains. Immigrant species are however more numerous in the connected mountains (“Model II” from Agakhanyanz and Breckle).
What about the Alps? The physical significance of this range and the fact that it is the most widely studied immediately led to comparisons between this and other very large ranges. Brockmann-Jerosch [5] studied the proportion of species common to the Alps and those of other large systems: the Caucasus, the Ural Mountains, the Altai Mountains and the Himalayas. With regard to the possible or presumed origins from the Altai Mountains or the Himalayas, contributions to ranges surrounding the Alps even at the heart of Europe have been ignored. The perspective is completely different if the focus is turned, not just to the Alps themselves, as prestigious as they may be, but to the entire alpine system in general. This system then appears as a vast and isolated entity which certainly generates autochthon plants on its own scale.
The separation of the two populations, autochthon and immigrant, is not abrupt, since the latter have had to undergo exchanges and reorganisation as part of their integration, which may have lead to the development of endemics.
(d) The history of plants must be separated from that of flora, since the time scale is completely different. In contrast with the wealth of knowledge acquired on the history of flora, we know relatively little about the formation of communities (biocoenoses, ecosystems). Plant population does not occur passively via significant physical events (uplift, glacial phases, etc.), rather it is structured by interactive development, reacting to the environment, climate and paedogenesis, via a process spanning many centuries.
(e) Biodiversity still presents modifications that are made more obvious by the fragility of mountain environments and which have two causes: increased human impact and climate change. These issues, which are currently very topical, are the object of a significant amount of works and publications in the Alps, like everywhere, which would be impossible to summarise here. They will only be mentioned for reference, with the exception of the effect of global warming on the alpine belt.
3 Formation of the autochthon core
3.1 A slow process
(a) The so-called arcto-tertiary period (see 1, Fig. 1). The first orophile flora of the Alps was formed from a lowland flora which must have included a mosaic of tropical or subtropical plants mixed with temperate plants, i.e., a landscape that is nowadays seen in south east USA, in part of China or on the south west backslope of the Caucasus Mountains in Colchis (Walter's zonobiome of laurifoliate forests). During this period, the alpine range probably comprised modestly high reliefs accommodating mid-mountain orophytes [18] just like in that time the Pyrenees and part of the Balkans.
Several genera (or more generally supraspecific taxa) survived from this initial flora, endemic to the alpine system, some of which are clearly related to tropical families or tribes: Borderea and Ramonda (Pyrenees), Haberlea (the Dinarides) and Berardia (Western Alps). Xatartia and Endressia from the Pyrenees and some from the Balkans must also be added [19]. These relics can mainly be found in the southern part of the alpine system: the Pyrenees (in particular, the Spanish part) and the Balkan Mountains; since these underwent less glaciations than the Alps; it is not surprising that genera belonging to tropical families such as the Gesneriaceae and the Dioscoreaceae survived here rather than in the Alps.
Other species, although not endemic, do, however, present characteristics of this flora: Wulfenia carinthiaca and Rhodothamnus chamaecistus, whose related species are more commonly found in Anatolia and the Middle East, and perhaps also the large Gentians from the Gentiana lutea tribe, which have no equivalent in the current lowland flora.
The formation of the arcto-tertiary core slowed down after the Miocene epoch, since the European subtropical flora decreased during this period [20]. Glaciations subsequently played a significant role in the extinction of this cortège, which was most vulnerable to the cold; it seems that competition would have afterwards led to their elimination anyway.
(b) The Pliocene epoch: contingents of temperate origin (2 and 3, Fig. 1). The majority of orophytes in the Alps most probably date from this epoch, as is the case for other large Mid-European ranges (the Pyrenees, the Carpathians and the Balkans). Differentiation of this flora occurred gradually from the end of the Miocene epoch, when global cooling occurred, and led to the distinction, in broad and for greater clarity, of at least two Mesogean and Mid-European contingents.
The formation of these contingents, which are largely dominant in these mountain's current flora, derives from a similar temperate flora which today covers southern and Central Europe (but also in part by the spread of Nordic species to the south). The relationship with the temperate stock of the European plains is evident: of the more than 600 genera that currently comprise the mid and high mountain flora in the Alps [13,21], around 30 are no longer represented in their lowland. Among 59 genera listed in the nival flora of the French Alps [22], only five are unknown in the lowland. A common origin, based on the common European stock, and certainly inter-chain exchanges, explain the relationship between flora in the Alps, the Pyrenees, the Carpathians and the northern Balkan Mountains (over 50%, if you take into account species and sub-species that are closely related or vicariants), as well as the biocoenotic similarity between the ranges [23,24].
The qualitative and quantitative differences between altitude flora and the mid-mountain or plain flora from which they originate has often led to oppose them. In fact, these are continuous, altitude plants are not a world apart but a borderline case. Generas which have succeeded in adapting to the alpine environment have found spaces here which are free from competition and where the parcelling of land and ecological variety has favoured diversification. This latter continued during the Pleistocene epoch, activated by climate changes and agitation of flora, which is perhaps advantageous at mid or low altitude [25,26].
Certain genera have given rise to a profusion of mountain species and the number of endemics is remarkable: close to 100 species in the Alps alone for all of the Cerastium, Viola, Primula, Campanula, Galium, Gentiana, Phyteuma and Achillea genera [27]. Several different levels can be distinguished within this significant endemism [28]. The first group includes species which are endemic to one single range, either the Alps only (around 500), or another chain, the Pyrenees, the Carpathians, the Dinarides or the northern Apennine Mountains: one thousand species in total. The second group includes species that exist in several ranges but are absent out of the alpine system, such as Rhododendron ferrugineum; in the absence of known statistics, these can be estimated at 200 to 300 species. The two groups as a whole represent at least one tenth of the flora present in Europe. What is most remarkable is the presence, in the second group, of supraspecific taxa: the genus Soldanella, Primula sect. Auriculastrum, Androsace sect. Aretia, Gentiana sect. Coelanthe and Megalanthe, Saxifraga sect. Aizoonia. The case of the Dentaria section of the Cardamine genus is particularly demonstrative: it includes around 10 species, all except one (C. bulbifera) endemic to the alpine orosystem, and linked to the beech forests of the mid-mountain.
The differentiation of the contingent of Mesogean origin (2, Fig. 1) had probably already started at the end of the Miocene epoch. The alpine species of Silene, Dianthus, Iberis, Globularia, Sempervivum and Linaria in particular, can be attributed to this period. An example of this dispersion via the entire alpine orosystem and the western Mediterranean region is the Senecio genus of the incanus group [29] and the Saxifraga genus of the Aizoonia section [28]. The high percentage of endemic species belonging to the Campanula and Saxifraga genera in the south-western Alps and the eastern Italian Alps is also remarkable [30]. One of the most interesting elements is the differentiation of the Juniperus thurifera species into two sub-species [31]. One is scattered throughout the mountains of Spain and Morocco and the other appears sporadically in the Pyrenees and in Corsica, but also in numerous stations and sometimes in significant populations in the south-western Alps [32]. Pinus nigra and perhaps Abies alba must also be attributed to this contingent, due to the current distribution of their related species throughout the Mediterranean.
This Mesogean contingent is, however, difficult to separate:
- – from the end phase of the arcto-tertiary group, to which Petrocallis, Minuartia, or even the above-mentioned Juniperus are linked;
- – from penetrations of pontic or south west Asian origins, from the extension of steppes linked to the drying of the Mediterranean Basin at the end of the Miocene epoch; and,
- – above all, from post-glacial ascent of species taking shelter during glaciations in the northern Mediterranean regions but which, unlike the Mesogean contingent, have not had time to form endemics.
The Mid-European contingent (3, Fig. 1) is certainly the largest and most distinctive group (echte Alpenelemente from Walter and Straka) [20]: eight of the 10 species existing at the highest altitude in the Alps belong to it [33]. The Dwarf Juniper, Juniperus communis ssp. alpina (= J. nana, J. sibirica) may be proposed as an example of this contingent. It is a sub-species formed from the common Juniper from which it is now well differentiated, in morphological and ecological terms [34]. In the Mediterranean mountains, prostate forms of the Juniperus communis s.str. are only acclimatised species or sub-species in the process of isolation (Juniperus hemisphaerica). A biochemical study of this complex [35] has showed the great complexity of its distribution and suggests that its evolution must still continue at present.
3.2 Inter-range exchanges
The relationship between flora from different ranges of the alpine system and the distribution of the above-mentioned contingents throughout the whole system, imply that exchanges must have always played a significant role. A large part may certainly be attributed to the broad dispersion mechanisms of diaspores (seeds, parts of inflorescences); however migrations within the system were certainly facilitated by the existence of intermediary reliefs between the large ranges. Therefore, the above-mentioned relationship between the Western Alps and the Pyrenees was certainly formed via an area that was then punctuated by reliefs which were smaller than the current ones (south of the central Massif and the Cevennes in particular) or have almost disappeared, such as the Provence secondary ranges which only remains as still imposing but isolated peaks.
3.3 A bicentrism of the Alps?
Certain massifs appear to have been particularly fertile, proven by the high number of endemics in the south-western Alps as well as in the Lombard and Julian Alps. In more general terms, as statistics increase, so too does the difference between the Western and Eastern Alps. Pawlowski [36] had already listed 165 and 203 endemics for each of the two parts of the range, as opposed to only 84 endemics represented in both parts at the same time. Moreover, a count of the total number in the alpine belt, according to Flora alpina, drew similar conclusions [37]. It would therefore seem that there exists a floral bicentrism in the alpine arc, like that in the Scandinavian range.
Looking beyond the framework of the alpine range to the continental level, the hypothesis may be put forward that this split in two is also a major division of the whole alpine system, based on two large centres of differentiation. For instance the alpine belt of the Western Alps has 52% of species in common with that of the Pyrenees in spite of the distance, but only 56% in common with the Eastern Alps despite the continuity. Noticeable is the case of the two pines of the subalpine belt, for a long time belonged to one species: Pinus uncinata, extends from the Pyrenees to the Swiss Alps, and its twin Pinus mugo from Piedmont to Bulgaria. Within the Soldanella genus, the area of the principal species coincides almost exactly with that of the orosystem whose this genus is endemic, however alone S. villosa is located in the west (the Pyrenean–Cantabrian region) while 12 other species are found in the eastern part (Austria, the Carpathians and the northern Balkans) [38].
4 Remote origins?
When referring to the boundaries of the alpine orosystem, as represented in Fig. 2, we exclude from migration concept relatively close contributions from other regions in Europe: the boreal fraction of the Mid-European contingent, a part of the Mesogean contingent, returns due to post-glacial reforestation, arrivals from the Atlantic such as Buxus, and of course xenophytes.
4.1 Migrations of Asian origin
The fundamental fact seems to be the floristic relationship with central Asian mountains, where the most widely cited are the Altai and the Himalayas. Genera such Primula, Pedicularis, Gentiana and Saussurea have maximum differentiation in these ranges. The Altai system is probably the most interesting, since it constitutes, due to its latitude, extension and altitude, the central Asian counterpart of the alpine orosystem [39]; however, it is the Himalayan origin that is most frequently cited. The Rhododendron genus, which includes hundreds of species in the mountains of China, a dozen in the Himalayas, only includes 5 in the Caucasus and 2 in the Alps. Along the Himalayan system, the number of species for each of the Primula and Pedicularis genera falls gradually from more than 40 in Bhutan to less than 20 in Kashmir.
However, there are certain difficulties. If, as is often suggested, after Gams, that the origin of a group must first of all be researched at the point of its maximum diversity, this theory must be tested with molecular genetics. On the one hand, these mountains are not all of the same latitude as the Alps, and large plains separate them from the European ranges; it is perhaps not the species themselves that were displaced, but ancestors that had a similar biology to that of the current hill plants, which were able to propagate following lowest reliefs. Moreover, it is to be noted that it is not necessary to invoke massive migrations from central Asia. Genera such as Rhododendron, Saussurea and Leontopodium are assumed to be of central Asian origin given their weak presence in the European mountains, but Primula, Pedicularis and Gentiana are originally part of European flora and more generally of the large temperate stock of Eurasia. Their gradual deterioration from the east to the west, from China to Western Europe, is not necessarily proof of migration, but perhaps simply a reflection of the relative lack of holarctic flora stock in Europe compared to that of central or Eastern Asia. Moreover, alpine taxa such as the Soldanella or the Aizoonia section of Saxifraga are endemic to Europe.
4.2 The Larch and Arolla Pine cortege
One important case is to be noted here: that of the flora, also with Asian relations, present in the intra-alpine axis. Taken as a whole, this axis appears uniform: it stretches, significantly unchanged, from the Austrian Alps to the Mercantour in the Alps Maritimes [37]. However, a more thorough analysis of its vegetation identifies two storeys: the high mountain, which is very similar to that of the Prealps, except for the predominance of siliceous substrates, and the mid-mountain, in which the most inner valleys are home to original plants [40] both in their ecology (thermal and hydric continentality) and biodiversity. The most distinctive elements are Asian-related: Larix europaea, Pinus cembra and a cortège of shrubby or herbaceous species (Astragalus alopecurus, Kochia prostrata) mixed with sub-Mediterranean penetrations.
Their set-up is often situated within the glacial age or the post-glacial repopulation. However, thousands of kilometres separate them from their presumed origin and their arrival in the Alps certainly took a long time. Coming from Siberia during the Pliocene cooling already, the Larch and Arolla Pine were then able to grow back during glaciations in the perialpine shelters, taking possession of the intra-alpine part during the interglacial periods then very early in the Late-Glacial epoch before about 9500 B.P. [41].
Other internal basins were established in the Tatras and the Pyrenees [42], but are the result of continental conditions only, and unlike the internal alpine region they did not accommodate any significant migrations.
5 Return of the called arctic-alpine effect
This is a vast and complex question, which is often reduced to the reductionist theory of an area disjunction. It would be impossible to summarise the extensive literature here and we will therefore only deal with new or controversial aspects.
Many species exist at once in the arctic and subarctic regions and on the other hand in the Alps and other Mid-European chains. The most frequently cited include: Dryas octopetala, Oxyria digyna, Linnaea borealis, Betula nana and numerous Salix. This is the case for 10% of European Pteridophytes and many Lichens such as Nephroma arcticum. Sometimes the two parts of the area are linked by intermediary stations, such as Dryas; they are generally completely separated, or even occupied by different forms or related species. It is also to be noted that entire communities are also found on both sides: Loiseleurietum, Kobresietum simpliciusculae, Caricetum bicoloris-atrofuscae, with the same characteristic species and the same structure.
Arctic-alpine disjunction is a well-established fact; the classic explanation is well known. The general cooling of the European continent led to, at the time of the glacial paroxysm, the advance to the south of subarctic plants. During each interglacial warming period, which may have been warmer than the current climate, and probably, to a certain extent, during each interglacial stage, this cold-climate flora was pushed back by warming and by the ascent of more southern thermophilous flora; they therefore moved towards colder territories, either in the North towards the northern glacial front, or at altitude in the Alps or other ranges. It is difficult to assess whether this ascent towards the colder climate was due to physiological non-resistance to warming, or competition between temperate species coming back in force.
This explanation, which is often overly simplified, does not really take into account the following:
- (a) It is normally accepted by default, based on Darwin and Hooker, that the geographical origin of arctic-alpine plants was arctic. In fact, some species, such as Linnaea borealis, are almost exclusively present in the northern part and are very rare on the alpine side. However, this traditional explanation requires significant revision. The relative lack of and the uniformity of current arctic and subarctic flora implies a marginal or recent flora, rather than a fundamental stock. It is more plausible that in genera such as Primula and Pedicularis, which include hundreds species in the Central-Asian mountains and several tens in European mountains, the less frequent species of Northern Europe are originally orophiles which followed the retreat of glaciers towards the north and are therefore not arctic-alpines as in the Carex or Salix genera, but rather alpine-arctics [2,3]. A detailed study would probably show that the case of alpine-arctics is so important as that of the true arctic-alpines.
- (b) It also appears that it is often not the case of one single species but of a pair of two isolated vicariant species from one common stem: therefore in the Salix genera, the glauca-glaucocinerea, retusa-polaris, lapponum-helvetic pairs or even Lomatogonium rotatum — L. carinthiacum, Cardamine bellidifolia — C. alpina. Based on an old hypothesis, part of these disjointed areas would be formed on the edge of a cold lowland complex, belonging to the general euro-Siberian stock, which would have differentiated, on the northern side and the alpine ranges side, taxa that is almost identical but of polygenic origin or very close vicariants. The two stocks formed in this way can therefore undergo exchanges during the vicissitudes of the glacial periods.
The arctic–altaic disjunction between northern Siberia and the Altai mountains must be affected by the same problems. Around fifty species even present arctic–altaic-alpine disjunction [20].
Moreover, successive to-and-fro glaciation movements gives some idea of the amount of time required for specification, taking for example the case of arctic-alpine willows [43]. The Salix genus is clearly of Nordic origin, since the number of species decreases steadily from north to south. The disjunction seems to concern of the first of the three mechanisms above. Of around 60 representatives in Europe, three quarters are cold-climate species inhabitant the boreal and arctic regions on one side and the alpine and subalpine belts of the high mountains on the other. If one part of the species comprises endemics localised on one side or the other, many are present on both sides.
Several levels of specification can be distinguished: (1) Salix myrsinifolia presents two areas, north and south, which are very extensive and linked by intermediary stations; (2) with respect to S. reticulata the two parts are reduced and well separated; (3) S. polaris is replaced to the south by S. retusa; (4) S. glauca, from the Scandes, is replaced in Iceland by ssp. callicarpaea, in the Alps by S. glaucosericea and in the Pyrenees by S. pyrenaica.
This analysis has the advantage that it concerns a phenomenon whose duration is relatively well known now that the chronology of Quaternary glaciations is also well established. Taking the simple theory upon which the disjunction and speciation process is in proportion to time, or the related theory according to which each of the above-mentioned different stages correspond to a different glaciation and taking into account that the estimated duration of approximately millions of years for all quaternary glaciations, therefore the differentiation of a species spreads over a period of around 200,000 to 400,000 years, this figure constituting at least an upper limit. This is, however, subject to that molecular genetics studies do not modify the internal systematics of the genus and call into question the existence of the groups of species mentioned here, such as those maintained in Flora europaea.
It may also be noted that the pairs of Salix mentioned above are still pairs of species and that there does not seem to be very close pairs of genera that may have been determined by the arctic-alpine disjunction. In more general terms, alpine orogenesis seems to have only determined differentiations at the species or section level and rarely led to real generic formations, except in some cases, such as that of Berardia, Xatartia or Wulfenia which seem to derive from a very old stock (so-called arctic-tertiary flora) or Soldanella for example. So that it may also be supposed that a period of one million years would be the lower limit for the duration of generic differentiation.
6 The set up of the biocoenoses
Observable cases of mountain ecosystems under formation are rare: at the very most, the reforestation of the moraines liberated by the retreat of glaciers, or the progression of woody populations on abandoned pastures may be cited. It is plausible that these older communities were very different to the current ones [44] and that, for example, comparisons between the vegetation of the late-glacial Dryas and the current cold steppes are quite forced.
6.1 Biocoenotic uniformity of the alpine system
As in the case of the autochthon flora, the extended framework of the orosystem is once again taken into account. This option is justified by its remarkable uniformity. Unity and originality therefore appear just as well in the forest formations of the mid-mountains as in the vegetation of the alpine belt.
In terms of forest formations, the most significant palaeobiological data comprises pollens from trees and macrofossil plant remains, and for the Quaternary only. These elements, that only concern the species themselves, did, however, allow the reconstitution of the succession of forest stages during the Holocene epoch [45]. This approach assumes that the tree species observed are also representative of the forest biocoenoses. It is justifiably remarkable that the European beech forests form three large ecological groups that are very similar from one range to the other in the Alpine System, besides the nuances of their related flora [14], so that traces of the presence of beech in a certain point can be a valuable indicator of the past existence of a beech forest complex in this region. This is less clear for coniferous forests; a relationship can however be seen between formations of subalpine and north-dinaric spruces in Slovenia or between those of the Tatras and the Sudetes [46], while the spruce forests of central Europe are significantly different to those of the Scandinavian mountains.
The biocoenotic unity of the supra-forest vegetation in the alpine system has been tested using traditional phytosociological alliances as a basis. The level of alliance has been maintained in this statistics since it refers to comparable concepts from one region to the other, while the associations can often be subdivided [47,48] in works focusing on the specificity of regions rather than the general characteristics of the alpine system. Taking as a reference the alliances represented in the central or Eastern Alps, those found in the other parts of the system were researched. The percentage of similarity evaluated in this way is high throughout the entire system: 100 for the Western Alps and the Tatras, 73 for the southern Carpathians, 67 for the Dinarides, 59 for the Pyrenees and 50 for the Bulgarian ranges [49].
6.2 Are the grasslands of our alpine belt the only ones in the world?
The originality of the alpine belt in the Alps, and in more general terms of the system, can be clearly seen when compared with the boreo-alpine belt of Northern Europe [50], with other Mediterranean mountains [51], with the Ural Mountains [52], the Himalayas [53] or the Rocky Mountains [54].
It is noted, however, that ecological, geomorphological or extreme climate formations (rocks, cliffs, marsh), the so-called azonal formations, are relatively similar in the Alps and northern Europe: these include dwarf-shrubs (Loiseleuria and Empetrum), snowbeds (dwarf Salix) and windy grasslands (Elyna myosuroides). However, the grasslands, the so-called zonal formations, are completely different. They were stable and natural during the entire Holocene (Urwiesen of Ellenberg) [55]. In a review of the alpine belt on a worldwide scale, Grabherr [56] concluded that the zonal grasslands of the Mid-European ranges are characteristic of these mountains only and are therefore endemic. One may question the reasons explaining this uniqueness. Is it the isolation of the alpine system in a corner of Eurasia, far away from the Asian mountain block? Or the significance of penetrations of Mesogean origin within the thermonemoral zone? A clear reason is that of the essential role played by graminoids, mainly of the Carex (C. curvula and C. sempervirens), Festuca and Sesleria genera, as species forming these biocoenoses (Grabherr's key species). In this way, the Festuca genus includes 37 species habitating or penetrating the alpine belt within the Alps range, of which 19 are endemic, compared to some species found only in the Scandes. It is perhaps in the Caucasus, where the Festuca alpine grasslands have a significant place, that the relationship with the Alps can be seen [57].
If the floristic originality of our altitude grasslands seems well established, it remains to be seen how their functioning as an ecosystem, studied by Grabherr in the Carex curvula grasslands [58], differs from that of the boreo-alpine grassland, for example [59].
6.3 Is the alpine belt under threat from warming?
The general increase in temperature (Global change) observed during the 20th century, which is evaluated at 0.8° to 1°, is also seen in the Alps. There could be a temptation to only see one continuation, or even acceleration, of post-glacial warming: the start of an interglacial period. However, as Huntly comments [44], the speed of warming is 10 to 100 times faster than post-wurmian deglaciation.
The most well-known effect of warming in mountains is the regression of glaciers: it often accompanied by reforestation of frontal moraines with vegetation, which is like a repetition, to a lesser extent, of the Late-Glacial epoch. This has led to detailed works in the Swiss and Austrian Alps from 1950, of which a history was recently provided by Burga, as well as a very detailed study of a case of the succession of herbaceous, fructuous and then woodland stages in the Austrian Alps [60,61].
It has been also confirmed that the enrichment of nival level flora, seen several times since 1950, has accelerated: as in the Swiss massif in the Bernina Mountains [62]. A comparison of records taken at the start and end of the 20th century show an increase in the number of flora in more than 10 of the summits. In three of them, at around 3000 metres, it almost tripled, with acceleration during recent decades. In the central Austrian Alps, the maximum altitudes observed are high, depending on the species, from 1 to 4 metres per decade for 70–90 years, the average temperature was also high at around 0.7°, from 8 to 10 metres per decade [63].
6.4 Could extrapolation occur in future years? A catastrophic scenario?
Warming from 2° to 3.5°, which is possible if the greenhouse effect accelerates during the next half century, could subsequently bring about, assuming that a balance has been obtained, an increase in ecological limits in 4 the mountains in the form of an entire vegetation stage [64]. The forest limit will rise (once the balance has been reached) to 2700 m in Dauphiné, 2500 m in Bavaria, and in the Pyrenees and Mercantour it will rise to 2900 m, perhaps leading to a significant reduction and most likely fragmentation of the territory within the alpine belt. If the extension of this belt is split between the rise of the timberline and the subalpine heathland on one side, and crests and summits on the other, the current surface of the alpine arc, which is evaluated at 1000 square kilometres, will be significantly reduced. The geographical extension of the belt, which is present all along the range, will be reduced into two separate parts located in the Western and Eastern Alps, following its disappearance in the lower massifs. Moreover, this residual area will be divided up into small islands. A significant reduction in the number of flora is therefore to be feared, the rarification of a number of species and even the extinction of a significant part of them. It is also certain that biocoenoses will not be deplaced as such but will be disassociated. However, it is still only a very schematic model:
- (a) Migration would be very different from one species to another, depending on the reproduction method of each species. High altitude plants, adapted to extreme conditions, will also undergo variations in these conditions and certain ones are capable of surviving for centuries in spite of variations in the environment [65]. The weak density of the occupation, the diversity of the microclimates and substrates determining the multiplicity of biotopes, would allow many species to limit their migration to one single station and survive there for a long time provided that reproduction remains possible. The fragmentation of the area will only have limited effects since the islands will be higher than 20 km2 or so.
- (b) Different barriers can frustrate migration: the nature of the soil, relief irregularity, competition, intervention of climatic factors other than temperature and variations in snow cover.
- (c) These migrations clearly require a very long time for balancing, much more so than the rise of isotherms [66]. The appearance of new adaptations is not excluded but would require a much longer time than the migrations themselves [67].
Acknowledgements
The author thanks Gabrielle and Jean-Luc Borel for their valuable help in the improvement of the English text and of the figures.