1 Introduction
Many studies dealing with male gametogenesis have been carried out to investigate the phylogeny of the Platyhelminthes [1–16]. These studies revealed some features that are useful for phylogenetics, such as the number and structure of the flagella, the presence or absence of mitochondria and the arrangement of microtubules.
Spermatogenesis has been described for many parasitic platyhelminthes species and there are several reviews in this field [6,7,8,14,17]. Although there have been many studies on male gametogenesis in the Turbellaria [13,14,18–23], these were generally restricted to descriptions of the mature spermatozoon. Few studies dealing with the ultrastructure of spermatogenesis have been reported [24,25]. Therefore, additional research in this group is required.
The species Schmidtea. mediterranea examined here has become a model organism for experiments as it provides certain advantages. It has been the subject of several experiments on its capability of regeneration [26,27], its developmental biology [28,29] and for molecular analysis [27,30,31]. Recently, its complete genome has been sequenced [30] and its evolutionary history has been established [32]. Nonetheless, little is known about its reproduction [33,34], although it presents some quite interesting phylogenetic characteristics. The objectives of this work were to describe for the first time the process of spermatogenesis at the light and electron microscope level in S. mediterranea as well as sperm maturation and ultrastructure. We also compared the ultrastructural elements and their organization in the mature spermatozoon of S. mediterranea with those of other Platyhelminthes.
2 Materials and methods
2.1 Specimens
S. mediterranea specimens were collected in Lebna dam in the northeast of Tunisia, approximately 2 km from the Mediterranean Sea. The samples were taken between December and May, when the species is sexually mature [35].
2.2 Microscopy
For light microscopy, specimens were fixed in Steinmann's or Bouin's fluid and embedded in paraffin wax. Sections (6 μm) were stained with eosin and toluidine blue or with gallocyanin. Following staining, sections were dehydrated in increasing concentrations of ethanol and toluene, then coverslipped.
For transmission electron microscopy, worms were fixed overnight at 4 °C in 3% glutaraldehyde in 0.1 M phosphate buffer (pH 7.2). Specimens were then cut into small pieces, postfixed in 1% osmium tetroxide in the same buffer for 2 h at room temperature, and embedded in Epon-Araldite. Ultrathin sections, prepared with a Reichert-Jung Ultracut E ultratome equipped with a diamond knife, were stained with uranyl acetate and lead citrate for examination in a Jeol 100 SX electron microscope.
3 Results
The classification of the different cells in the testes of S. mediterranea proposed here is based mainly on the topographical arrangement of the cells and on previous studies.
3.1 Light microscopy
The numerous testes in S. mediterranea are situated dorsally, extending from anterior to the ovaries to almost the end of the tail (Fig. 1). They are rounded or oval shaped, stretch dorso-ventrally and measure 155 × 135 μm (Fig. 2a). Starting from the periphery to the center of the follicle, there are different stages of spermatogenesis and spermiogenesis (Fig. 2b). The spermatogonia are located peripherally and can be divided in two types. Type I spermatogonia have a large and pale nucleus. Type II spermatogonia are significantly smaller than the one of type I, and show a darker and central bulky nucleus. They may correspond to paired or grouped spermatogonia issued from mitosis underwent by spermatogonia type I. Toward the central region of the testis, there are distinct clusters of cells containing clumps of spermatocytes, of spermatids or of spermatozoa that can be distinguished on the basis of the aspect of the nucleus. At early pachytene stage, primary spermatocytes show clear nuclei with thick filaments. Identification of secondary spermatocytes is uneasy and cells with dark nuclei observed in the lumen probably correspond to this type of cells. Few spermatids are easily identified according to their dark nucleus due to the compaction of the chromatin. The last stage of spermiogenesis corresponds to the mature spermatozoa present in the lumen of the testis.
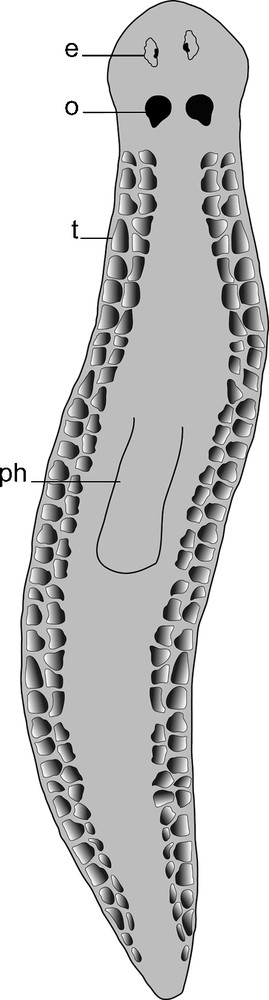
A schematic drawing showing the distribution of testes on the dorsal side of S. mediterranea. e: eye; o: ovary; ph: pharynx; t: testis.
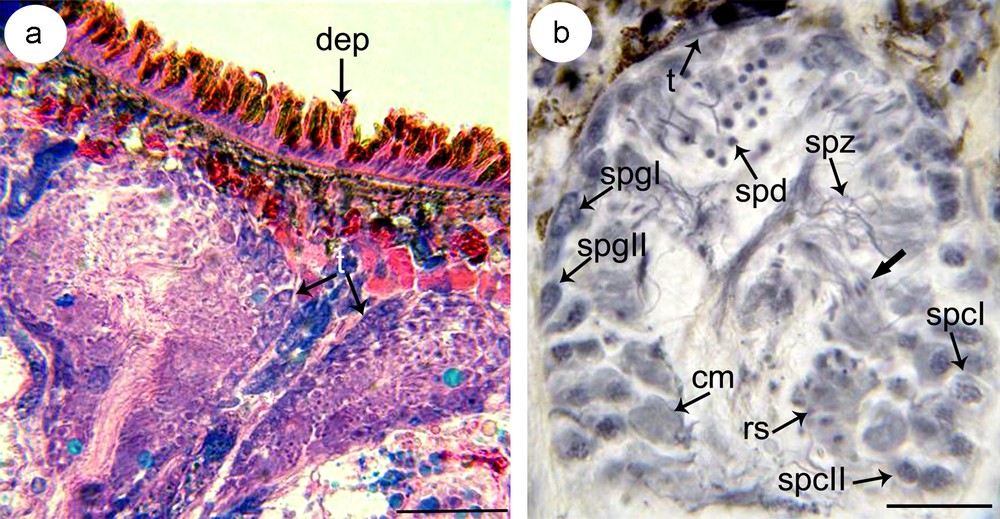
(a–b) Microphotograph showing the disposition of testes (t) on sagittal section (a) and the distribution of germ cells in different stages of spermatogenesis (b). Note from the periphery to the lumen of the testis basophilic type I spermatogonia (spgI) and type II spermatogonia (spgII), clusters of distinctly larger primary spermatocytes (spcI) and secondary spermatocytes (spcII), rosettes of spermatids (spd) and spermatozoa (spz). a: stained with eosin and toluidine, b: stained with gallocyanin. cm: cytoplasmic mass; dep: dorsal epidermis; rs: rosette of spermatocytes II; t: tunique.
3.2 Electron microscopy
The testis contains five types of cells: spermatogonia (types I and II), spermatocytes (types I and II), spermatids and spermatozoa.
Both spermatogonia type I and type II are rounded cells with a high cytoplasm: nucleus ratio. Each type I spermatogonia has a large nucleus containing a single nucleolus and granular chromatin with few clumps of heterochromatin scattered throughout a granular nucleoplasm (Fig. 3a). The reduced cytoplasm contains free ribosomes, few mitochondria and an electron-dense hemispherical mass of material: the chromatoid body. The cytoplasm of type II spermatogonia increases relatively compared to that of type I spermatogonia. It contains many free ribosomes and mitochondria (Fig. 3b). The latter are small, oval or round in profile, with lightly staining matrices. The granular and agranular endoplasmic reticulum are poorly represented whereas Golgi complexes were not observed. Beneath the nucleus, the chromatoid body is present.
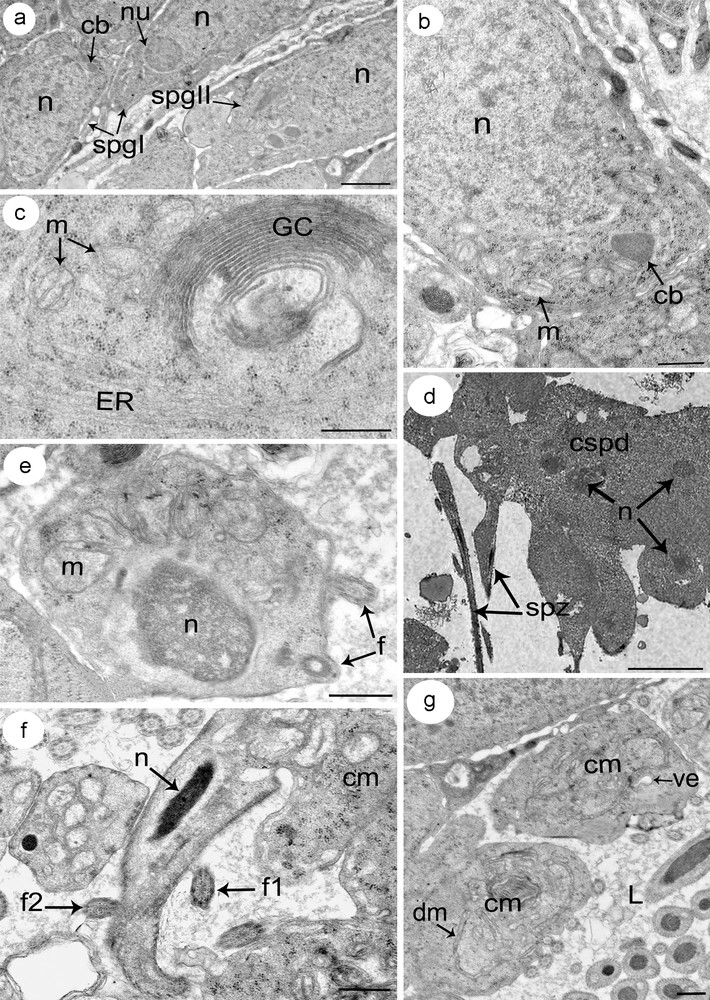
(a–g) Electron micrographs showing different stages of spermatogenesis. (a) A part of a testis showing spermatogonia type I (spgI) with large nucleus and reduced cytoplasm, and spermatogonia type II (spgII) with increased cytoplasm compared to type I spermatogonia (spgI). (b) Detail of a spermatogonia type II showing a large nucleus and relatively reduced cytoplasm containing numerous free ribosomes, rounded mitochondria (m) and chromatoid body (cb). (c) Detail of a cytoplasm from a spermatocyte distinguished by the prominent development of an endoplasmic reticulum (ER) and Golgi complexes (GC). (d) A rosette of spermatids. Note how cells still connect in cluster (cpsd). (e) A spermatid in the early stage of differentiation characterized by an ovoid nucleus (n) with condensed chromatin, and numerous mitochondria (m) which begin to cluster at this stage. Note the incorporation of the two flagella (f). (f) A section showing a differentiating spermatid with the conical-shaped projection of cytoplasm containing the elongated nucleus (n) and the arising of the two flagella (f1 and f2). (g) The remaining mass of cytoplasm (cm) after the releasing of the spermatozoa. It consists of many vacuolar elements (ve) together with some degenerating mitochondria (dm).
Primary spermatocytes are the largest of the cells formed during spermatogenesis with a marked increase in cytoplasmic volume. They are obtained after the mitotic division of type II spermatogonia and remain interconnected by cytoplasmic bridges. Their cytoplasm is characterized by an abundant amount of endoplasmic reticulum (ER) mostly associated with the Golgi complex (Fig. 3c). Ribosomes are scattered through the cytoplasm, sometimes assembled in clusters. Mitochondria are scattered with clearly visible matrice. Secondary spermatocytes, obtained by meiotic division of primary spermatocytes, are smaller than the latter and contain relatively the same organelles found in the cytoplasm of primary spermatocytes.
Spermatids arise from secondary spermatocytes, which divide to give a rosette of spermatids that still connected to a central cytoplasmic region (Fig. 3d). All spermatids of the same rosette are apparently identical in their rate of development, suggesting that their differentiation into spermatozoa is simultaneous. Each early spermatid has an ovoid shape with a large subspherical nucleus (Fig. 3e). In their cytoplasm, several mitochondria with lightly staining matrices are visible, together with granular ER and clusters of ribosomes. They begin to cluster at this stage, which indicates that they may fuse into a single elongated mitochondrion. The central cytoplasmic process and the two lateral flagella can be seen at this stage. Differentiation of a spermatid into a mature spermatozoon begins with the outgrowth of a conical-shaped projection of cytoplasm from the apical end of the spermatid (Fig. 3f). Then, the nucleus–characterized by a fibrillar structure–elongates and migrates into the projection pushing ahead the two flagella. The complete migration of mitochondria occurs at a final stage of spermiogenesis after nuclear migration has finished. The elongation continues until the newly formed spermatozoon is released from the general cell mass. The remaining mass of cytoplasm consists of many vacuolar elements together with some degenerating mitochondria and ER cisternae (Fig. 3g).
Based on transverse and longitudinal sections examined by electron microscopy, the mature spermatozoon of S. mediterranea can be differentiated into three regions (Figs. 4a–k; Fig. 5).
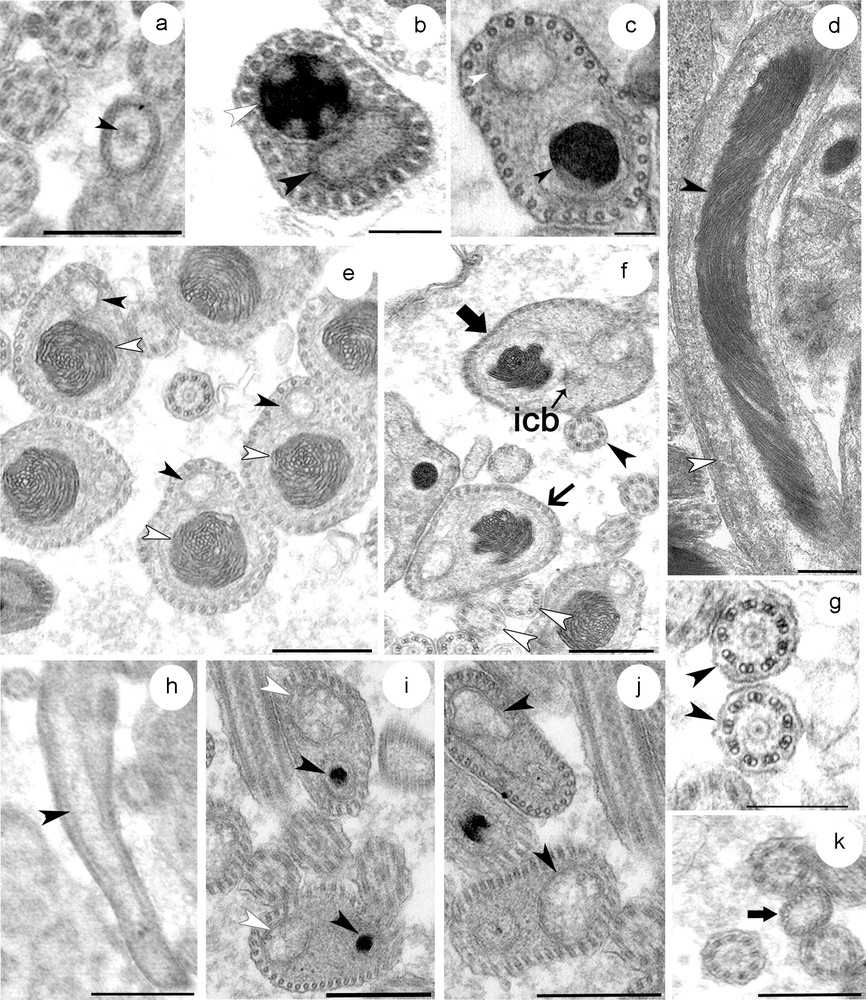
(a–k) Transverse and longitudinal sections at different level of the mature spermatozoon. (a) Transverse section through the proximal zone of the region I showing the anterior extremity of the nucleus. (b) The middle zone of the first region showing the sections of the condensed nucleus close to that of mitochondrion and a row of 30 cortical microtubules. Note the reduced cytoplasm at this level. (c) The distal zone of the region I is characterized by the increase of the amount of cytoplasm. (d) Longitudinal section of a spermatozoon at region I and II showing the elongated and helical structure of the nucleus, and the disposition of the latter together with mitochondrion. (e–f) Section through region II of the spermatozoa distinguished by a large nucleus with helical structure of the chromatin. The number of cortical microtubules is up to 40 at this proximal zone (e) and up to 50 at the distal zone (f). (g) The axonemal pattern in S. mediterranea (h–k) Portions of the region III containing only the mitochondrion (h) and the cortical microtubules. This zone starts when the distal extremity zone of the nucleus disappears (i). The number of microtubules between 30 and 40 at the proximal zone of this region (j), and decreases progressively at the tail zone until up to 20 microtubules (k).
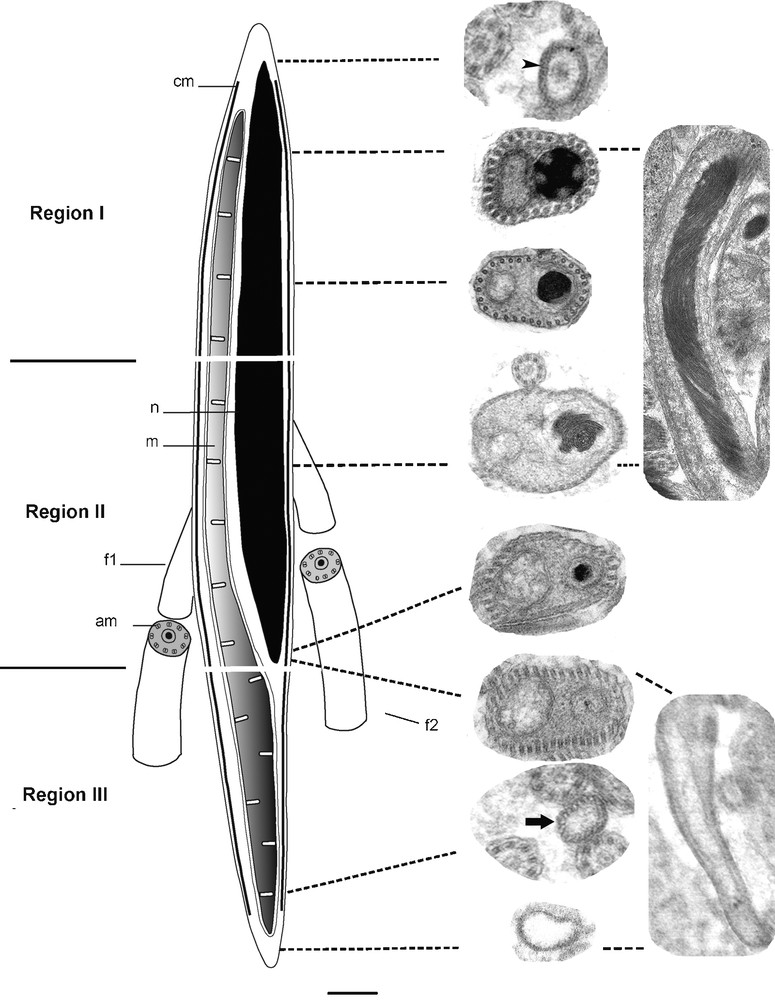
Reconstruction showing the ultrastructural organization of the mature spermatozoon in S. mediterranea. cm: cortical microtubules; n: nucleus; mitochondrion; f1: flagellum 1; f2: flagellum 2; am: axonemal microtubules.
The proximal part of the region I of the spermatozoon is characterized by the absence of the nucleus, the mitochondrion and the cortical microtubules. The plasma membrane encloses a medium-clear cytoplasmic content (Fig. 4a). At the middle zone of this region, there is a condensed nucleus and mitochondria which are found close together due to the reduced amount of cytoplasm at this level (Fig. 4b). The adjacent plasma membrane on this side is supported by a single layer of 30 microtubules, which appear to be orientated in straight lines and parallel with the longitudinal axis. The distal zone of this first region becomes wider which make nucleus and mitochondria less close to each other (Fig. 4c).
The region II represents the middle region of the spermatozoon. The proximal and middle zones of this region are characterized by a nucleus that is wider than the mitochondrion. In longitudinal section, the chromatin appears to form a helical pattern (Fig. 4d). The number of microtubules increases to between 30 and 40 at the proximal zone (Fig. 4e) and to between 40 and 50 at the middle zone (Fig. 4f). They lie immediately beneath the plasma membrane and circumscribe the body of the spermatozoon. The first flagellum can be seen emerging at this region. It comprises nine sets of doublet microtubules arranged in a cylinder around a central unit and therefore presents the typical 9+‘1′ trepaxonematan axonemal pattern (Fig. 4g). The second flagellum appears some distance from the first indicating that its emergence from the spermatozoon does not occur at the same level (Fig. 5). It possesses the same 9+’1′ pattern. In all sections of the spermatozoon examined by electron microscopy, we never found sections of axonemes inside the head region, suggesting that they become free at each side of the spermatozoon head very close to their attachment. At the distal extremity of this region, the nucleus, which has homogeneous chromatin, is narrower than the mitochondria until it disappears completely at the region III.
The region III represents the tail section of the spermatozoon. It starts when the distal extremity zone of the nucleus disappears and contains only the mitochondrion and a number of microtubules between 30 and 40 (Figs. 4h–j). The latter decreases progressively until up to 20 microtubules (Fig. 4k). The distal zone of the tail region forms the extreme tip of the spermatozoon characterized by lightly staining content devoid of the mitochondrion and microtubules.
4 Discussion
The structural organization of the testis of S. mediterranea and the development of its spermatozoa is similar to that described in other species of Turbellarian.
The main cellular features of spermatogonia, spermatocytes, spermatids and spermatozoon have been described in S. mediterranea at an electron microscopic level in this study. The amounts of ER and Golgi complexes increase greatly in both spermatocytes and spermatids. This has been demonstrated in previous studies but their function remains unclear [25,36]. In vertebrate spermatogenesis, it is known that Golgi complexes observed in spermatocytes are responsible for the production of the carbohydrate-rich granules that form the acrosomal cap. Recently, the presence of testosterone was detected in the hermaphroditic freshwater planarian B. brunnea towards the end of spermatogenesis [37]. Moreover, the study revealed that the levels of testosterone at this stage were three times higher than during the spermatocyte-building phase, suggesting that the increase of ER and Golgi complex is related to testosterone synthesis. It has been demonstrated that DeY1 genes–which play a role as gene regulators during spermatogenesis both as transcription factors and modulators of translation–are expressed in spermatogonia and at a higher level in spermatocytes and spermatids, while no expression was detected in spermatozoa [38]. On the other hand, Simoncelli et al. [39] demonstrated that an unusual tubulin isoform (SpTub-1) was expressed specifically in the testis of Schmidtea polychroa. The SpTub-1 mRNA was expressed in spermatogonia, spermatocytes and spermatids but never in spermatozoa, which indicates that this protein expression characterizes undifferentiated and differentiating germinal cells. Based on these previous findings, we suggest that the ER and Golgi complex elaborated during spermatogenesis might contribute to the synthesis of proteins and hormones in the male gonad of S. mediterranea such as testosterone, transcription factors and tubulin. This synthesis could be under the control of genes such as DeY1.
The numbers and disposition of cortical microtubules, flagella and mitochondria in spermatozoa of Platyhelminthes vary remarkably between groups, suggesting the value of these features in phylogenetics [5–8,40,41]. Thus, Justine et al. [42] proposed the classification of monogenean spermatozoa into four patterns using the number of axonemes and the presence or absence of cortical microtubules. The cortical microtubules would seem to give support and protection to the spermatozoon's structure. Their disposition and their number allow one in some cases to distinguish between very closely related species [43]. In S. mediterranea, we noted that the number of cortical microtubules increased from the anterior extremity of the spermatozoon (up to 30) to the middle region (up to 50) and then decreased to the tail up to 20. This organization has been observed in many other parasitic Platyhelminthes [15,16,44–47] and in freshwater planarians [25]. However, in other species the number decreases when going from anterior to posterior [48–50]. They can disappear entirely in some species of Nemertodermatides and Acoeles [3,51], and in some Digenes and Monogenea [11,12,52].
S. mediterranea possesses two axonemes with a 9+‘1′ pattern structure as in most forms of flatworms [20,53–58], with relatively few exceptions in the Acoel with a 9+2 arrangement [21,59] and in the Digenea with a 9+0 pattern [12].
The spermatozoon of S. mediterranea possesses an elongate mitochondrion that lies parallel to the spermatozoon nucleus from the middle zone of the first region until the subterminal extremity of the spermatozoon. Such organization characterizes many triclads [54,56–58]. Nevertheless, the numbers of mitochondria can increase among some species of Platyhelminthes, up to two or three mitochondria [22,48,60,61] but might disappear completely in the cestodes [55].
A dense cytoplasmic body has been noted in early stages of spermatogenesis of most Turbellarians [14,57,62] and even during the early oogenesis of many freshwater planarians such as S. mediterranea [33]. It has been reported in other animals as the ‘Balbiani body’ in female germ cells [63] or as the chromatoid body in mammalian spermatocytes and spermatids [64]. In S. mediterranea, we observed it only in spermatogonia, more rarely in primary spermatocytes but never in secondary spermatocytes, as is the case in other groups [65]. Thus, it constitutes a pertinent criterion of determination in early germ cells. Although it is considered as a plesiomorphic feature in Platyhelminthes [22], its function has not been established precisely. It has been described in other animals as an intracellular focal domain that organizes and controls RNA processing in male germ cells [64] or as RNA degradation site [66]. Further molecular characterization of its precise function in male germ cells is required.
Disclosure of interest
The authors declare that they have no conflicts of interest concerning this article.
Acknowledgments
The authors extend their appreciation to the Deanship of Scientific Research at King Saud University for funding the work through the research group project NoRGP-VPP-164. We also think the encouragement of the Tunisian Ministry of High Education and Scientific Research.