1 Introduction
It is widely accepted that plant growth is tightly regulated by both plant growth substances, such as indole-3-acetic acid (auxin, IAA), and environmental factors, such as temperature [1–5]. It was established that temperature-dependent plant growth correlated with the level of free auxin [1] and depended on auxin transport as well as IAA metabolism [2]. It was also shown that binding of auxin to its receptor was temperature-dependent [6].
The effect of auxin on plant cell elongation and proton extrusion is a well-studied aspect of auxin action. The correlation between IAA-induced proton efflux and acceleration of elongation growth proves the acid growth theory, which explains the mechanism of auxin-induced growth (for a review, see [7]). According to this theory, auxin-induced medium acidification is mediated by the increase in either the activity or the amount of plasma membrane (PM) H+-ATPase [8,9]. The PM H+-ATPase converts the chemical energy from the hydrolysis of ATP to ADP into an electrochemical gradient of H+ ions, which results in apoplast acidification and hyperpolarization of the membrane potential. The cell wall acidification provides favourable conditions for cell wall loosening, which is required for cell elongation, while plasma membrane hyperpolarization is needed to uptake of osmotically active ions, especially K+ [8,10–12].
The temperature dependence of ATP hydrolysis and proton transport for maize roots by vanadate-sensitive PM H+-ATPase was previously found by Brauer et al. [13]. These authors suggested that proton transport and ATP hydrolysis by the vanadate-sensitive PM H+-ATPase were indirectly coupled and the temperature optimum for proton transport by the vanadate-sensitive proton pump from maize roots was found to be between 20 and 30 °C.
Cadmium and lead are major environmental pollutants, which cause multiple direct and indirect effects on plant growth and metabolism [14–19]. These metals produce alterations in the functionality of membranes, by changing their permeability, fluidity, protein and lipid composition [20–26] and by affecting the activity of enzymes associated with a membrane such as the PM H+-ATPase [23,27–29]. Many authors previously demonstrated that Cd induced rapid depolarization of the membrane potential [18,24,27,30,31] resulting from the decreased PM H+-ATPase activity [23,28,29,32]. In contrast to Cd, Pb-induced changes to the membrane potential depend on the metal concentration and plant species. For example, in Nitellopsis obtusa cells, Pb at lower concentrations (0.01 and 0.1 mM) caused hyperpolarization of the plasma membrane potential, whereas at a higher concentration (1.0 mM), depolarization was observed [33]. In turn, what was found in Zea mays was a marginal depolarization of the membrane potential in the cells of roots [27] and coleoptile [34] incubated at 0.01–0.50 mM.
In spite of considerable research devoted to the effect of Pb and Cd on the growth of higher plants [18,31,35–38], only a few papers deal with the relationship between the temperature and the toxic effect of these metals. For example, Öncel et al. [14] showed on wheat plants that Cd toxicity increases in parallel with the temperature whereas Pb did not have any toxic effect irrespective of the temperature. It was also found that increased temperature enhanced Cd uptake in Solanum nigrum and Lolium perenne ([39] and literature therein).
The main goal of the current study was to determine the interrelations between the temperature (20, 25 and 30 °C), heavy metals (Cd, Pb) and the elongation growth of maize coleoptile segments by:
- • studying the effects of the temperature and Cd or Pb on growth in the absence (endogenous growth) or presence of IAA (IAA-induced growth) in the incubation medium, where the growth and pH changes of the incubation medium were measured simultaneously;
- • determining Cd and Pb accumulation, for each temperature respectively, in maize coleoptile segments.
2 Material and methods
2.1 Plant material and growth conditions
Seeds of maize (Zea mays L.) cv. Koka were soaked in running tap water for 2 h and then sown on wet wood wool in plastic boxes and placed for 4 days in a growth chamber (type MIR-553, Sanyo Electric Co., Japan) at 27 °C. The experiments were performed with 10-mm long coleoptile segments cut from 4-day-old etiolated seedlings. The coleoptile segments, with the first leaves removed, were cut 3 mm below the tip of the seedlings.
2.2 Chemicals
An aqueous stock solution (1 mM) of indole-3-acetic acid (Serva, Heidelberg, Germany) was prepared with the potassium salt of IAA. IAA at a final concentration of 10 μM was used.
An aqueous stock solution of CdCl2 (Fluka, Switzerland) or PbCl2 (Fluka, Switzerland) at the final concentration 0.1 mM was used.
2.3 Growth and pH measurements
The experiments were performed in which growth and proton extrusion were measured simultaneously in the same tissue [11]. The record of longitudinal extension of a stack of 21 segments was held by the shadowgraph method (magnification ×21). The coleoptile segments (each 10 mm in length) were placed in the incubation medium of the following composition (control medium, Artificial Pond Water [APW]): 1 mM KCl, 0.1 mM NaCl, 0.1 mM CaCl2 and initial pH 5.8–6.0. The volume of the incubation medium in the growth and pH-measuring apparatus was 6.3 ml (0.3 ml segment−1). In this set-up, the segments were incubated (in an intensively aerated medium) for 7 h at the desired temperatures (20, 25 and 30 °C). The flow of the medium was driven by a peristaltic pump (type Peri-Star PRO, World Precision Instruments, USA). The measurements of pH were performed with the pH-meter CP-315 (Elmetron, Poland) and the pH-electrode OSH 10 – 10 (Metron, Poland). The temperature control for all solutions was achieved by immersing the elongation and pH measuring system in a thermostatically controlled water bath. Prior to the addition of auxin (10 μM) and Cd (0.1 mM) or Pb (0.1 mM) to the incubation medium, the coleoptile segments were equilibrated for 2 hours at the desired temperature. Both metals concentration (0.1 mM) was chosen taking into account our previous papers [18,31] and also experiments performed by other authors [17,37], who showed that Cd and Pb at 0.1 mM caused moderate toxic effect on plant growth. Simultaneous measurements of elongation growth and pH were recorded every 15 min under the same conditions. All manipulations and growth measurements were carried out under dim green light.
2.4 Metal accumulation
The effect of temperature (20, 25 and 30 °C) on Cd and Pb concentrations in maize coleoptiles segments was measured by emission spectrometry with the excitation by argon inductively coupled plasma technique (ICP-AES) by means of a Spektroflame-M spectrophotometer (Spectro Analytical Instruments, Germany). Before chemical analysis, 110 intact coleoptile segments were split along the long axis and preincubated for 2 h in an intensively aerated APW medium (control) at the desired temperature (20, 25 and 30 °C). The composition and volume (0.3 cm3 segment −1) of the incubation medium were the same as that used in growth experiments. After preincubation of the coleoptile segments, cadmium (0.1 mM) or lead (0.1 mM) were introduced into the incubation medium for 5 h. Moreover, another treatment was studied, in which metal (Cd or Pb) was added together with IAA (10 μM). After each treatment, the halves of the segments were removed from the solution and washed three times with deionized water, whereupon they were dried at 80 °C to determine their dry weight. For cadmium and lead analyses, dry plant tissue was mineralized. Each sample (of ca. 0.2 g dry matter) was treated with 5 ml ultra-pure concentrated nitric acid (Merck) and left for 24 hours. Next, the samples were digested until complete mineralization was achieved. After mineralization, the samples were diluted with redistilled water to the volume of 10 ml. The concentration of cadmium and lead were measured by ICP-AES (frequency 27.12 MHz, power 1.0 kW, plasma gas 14.0 l/min, auxiliary gas 0.5 l/min, carrier gas 1l/min, analytical line: Cd2+ 228.802 nm, Pb2+ 220.353 nm). As standards for control of the elemental analysis, Virginia tobacco leaves (CTA-VTL-2) were used; the results fit the range of certified recommended values.
All experiments concerning the accumulation of Cd2+ and Pb2+ were replicated at least four times. The results are expressed as mean values ± SE.
2.5 Statistical analysis
The data were analyzed by using the computer software by StatSoft, Inc. (2008) Statistica (data analysis software system), version 8.0. www.statsoft.com. The differences between individual treatments and the control were analyzed using one-way Anova and LSD-test. Pearson's correlation coefficients between the temperature of medium and metal accumulation or the elongation growth of maize coleoptile segments were also calculated. Statistical significance was P < 0.05.
3 Results
3.1 Effect of Cd and Pb on endogenous and IAA-induced elongation growth of maize coleoptile segments incubated at various temperatures
Figs. 1 and 2 show the effect of temperature (20, 25 and 30 °C) on endogenous (without exogenous auxin) and IAA-induced growth, respectively, of maize coleoptile segments treated with Cd and Pb.

Effect of Cd and Pb on endogenous elongation growth (without exogenous IAA) of maize coleoptile segments incubated at various temperatures (20, 25 and 30 °C). A. The growth of a stack of 21 segments (10 mm in length), expressed as their elongation (μm cm−1). After preincubation (2 h at the desired temperature) of the coleoptile segments in a control medium, a metal (Cd or Pb) was added (arrow). Values are means (n = 8). B. Cumulative growth, after 7 h of the experiment, of maize coleoptile segments. After 2 h of preincubation, a metal (Cd or Pb) was added. Values are means (n = 8). Bars indicate ± SE. Means followed by the same letter are not significantly different from each other using the LSD-test (P < 0.05).

Effect of Cd and Pb on IAA-induced elongation growth of maize coleoptile segments incubated at various temperatures (20, 25 and 30 °C). A. The growth of a stack of 21 segments (10 mm in length), expressed as their elongation (μm cm−1). After preincubation (2 h at the desired temperature) of the coleoptile segments in a control medium, a metal (Cd or Pb) and IAA were added (arrow). Values are means (n = 8). B. Cumulative growth, after 7 h of the experiment, of maize coleoptile segments. After 2 h of preincubation, a metal (Cd or Pb) and IAA were added. Values are means (n = 8). Bars indicate ± SE. Means followed by the same letter are not significantly different from each other using the LSD-test (P < 0.05).
As can be seen in Fig. 1, the endogenous growth (growth in the control medium – APW) of maize coleoptile segments increased when the medium temperature was raised from 20 to 30 °C. The maximal endogenous growth of maize coleoptile segments was observed at 30 °C (1595.2 ± 76.1 μm cm−1) and it was 70 and 128% greater compared with segments grown at 25 and 20 °C, respectively (Fig. 1B). Regardless of the temperature of the incubation medium, Pb diminished the endogenous growth of maize coleoptile segments by about 20% within 5 h of treatment with this metal. Moreover, it was shown (Fig. 1) that the growth of maize coleoptile segments incubated at 20 °C in the presence of Cd was not significantly affected, whereas Cd at 25 and 30 °C inhibited the elongation growth of segments by respectively 35 and 9% compared with the controls (APW at 25 or 30 °C). The time at which the toxic effect of Pb or Cd was observed, depended on the desired temperature (20, 25 or 30 °C) (Fig. 1A).
Similarly to the endogenous growth, the maximal IAA-induced growth of maize coleoptile segments was observed at 30 °C (Fig. 2). At this temperature, the elongation of the segments in the presence of IAA was enhanced by 38% compared to the maximal value of endogenous growth (Figs. 1B and 2B). Interestingly, IAA, irrespectively of temperature, did not change the growth response of maize coleoptile segments incubated in the presence of Cd or Pb (Figs. 1 and 2).
3.2 Effect of Cd and Pb on pH changes of the incubation medium of maize coleoptile segments incubated at various temperatures
Simultaneously with the endogenous and IAA-induced growth of maize coleoptile segments, pH changes of their incubation medium were measured. The temperature dependence of medium pH of maize coleoptile segments was expressed as Δ [H+], where Δ [H+] means the difference between H+ concentration at 7 and 2 h (Δ [H+]). The data in Fig. 3 indicate that the proton extrusion by coleoptile segments in the control medium (APW) increased when the medium temperature was raised. Maximal medium acidification (Δ [H+]) was observed at 30 °C and it was 1.5 nmol [H+]/segment. IAA added (after 2 h of preincubation) to the medium containing coleoptile segments caused an additional proton extrusion, which similarly to the control, increased when the medium temperature was raised and reached the maximum (3.40 nmol [H+]/segment) at 30 °C (Fig. 4).

Effect of Cd and Pb on medium pH of maize coleoptile segments incubated at various temperatures (20, 25 and 30 °C) measured simultaneously with endogenous growth. pH changes of the incubation medium of maize coleoptile segments are expressed as Δ[H+], where Δ[H+] means the difference between H+ concentration ([H+]) at 420 min and 120 min. Values are means (n = 8). The differences between the values of H+ concentrations followed by the same letter are not significantly different from each other using the LSD-test (P < 0.05).
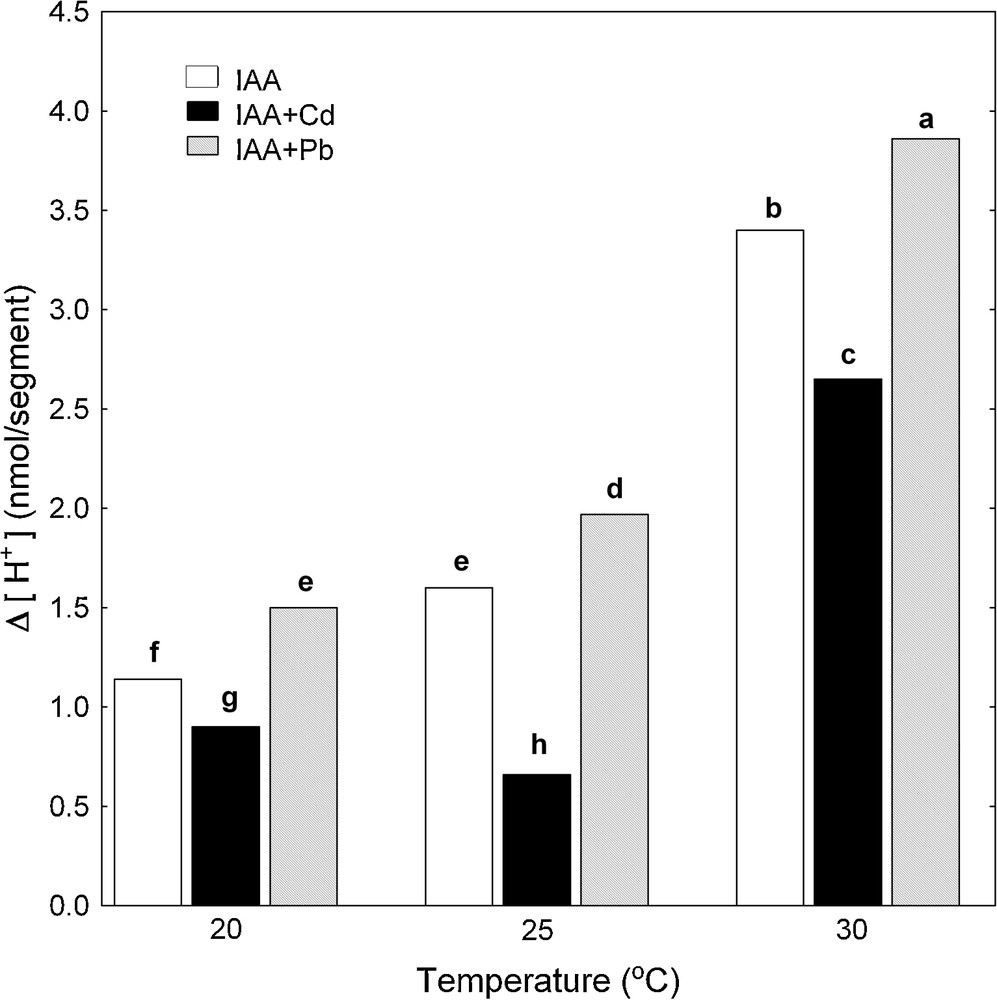
Effect of Cd and Pb on medium pH of maize coleoptile segments incubated at various temperatures (20, 25 and 30 °C) measured simultaneously with IAA-induced growth. pH changes of the incubation medium of maize coleoptile segments are expressed as Δ[H+], where Δ[H+] means the difference between H+ concentration ([H+]) at 420 min and 120 min. Values are means (n = 8). The differences between the values of H+ concentrations followed by the same letter are not significantly different from each other using the LSD-test (P < 0.05).
Pb added into both control medium (Fig. 3) and medium containing IAA (Fig. 4) stimulated much more effectively acidification of the medium compared to Cd, with the exception of proton extrusion at 20 °C, where there was not statistical significant difference between control medium and control medium with Pb or Cd (Fig. 3). By contrast to Pb, Cd significantly suppressed proton extrusion (Figs. 3 and 4), except for cases when this metal was added into control medium (APW) at 20 and 30 °C (Fig. 3).
3.3 Effect of temperature and IAA on accumulation of Cd and Pb in maize coleoptile segments
Under control conditions (without heavy metals), irrespective of temperature of the incubation medium, there were no statistical differences in the content of Cd and Pb in maize coleoptile segments (Table 1). The accumulation of Cd in maize coleoptile segments treated with Cd (0.1 mM) increased when the medium temperature was raised from 20 to 30 °C. IAA added together with Cd at 25 and 30 °C did not change the content of Cd in coleoptile segments. The addition of IAA together with Cd to the medium at 20 °C diminished the content of Cd in coleoptile segments compared to the concentration of this metal in the segments incubated without IAA.
Effect of temperature and IAA on accumulation of Cd and Pb (mg kg−1 d.w.) in maize coleoptile segments.
Metal (mg · kg−1 d.w.) |
Treatment | Temperature (°C) | ||
20 | 25 | 30 | ||
Cd | APW (control) | 0.64 ± 0.07f | 0.65 ± 0.06f | 0.75 ± 0.12f |
APW + IAA | 0.69 ± 0.12f | 0.57 ± 0.00f | 0.72 ± 0.08f | |
APW + Cd | 1159.5 ± 93.6d | 1400.5 ± 57.8c | 1616.6 ± 12.2a | |
APW + IAA + Cd | 970.8 ± 150.2e | 1446.6 ± 26.6bc | 1554.7 ± 37.7ab | |
Pb | APW (control) | 1.06 ± 7.99d | 1.16 ± 0.83d | 1.19 ± 1.18d |
APW + IAA | 16.33 ± 10.64d | 7.50 ± 4.96d | 8.44 ± 6.66d | |
APW + Pb | 1431.6 ± 94.4c | 2289.1 ± 102.7a | 1710.8 ± 95.4b | |
APW + IAA + Pb | 1682.9 ± 83.7bc | 1704.9 ± 200.4b | 1731.2 ± 81.2b |
In contrast to Cd, Pb content in maize coleoptile segments did not correlate with the increasing temperature of the incubation medium. The highest concentration of Pb in the segments was observed at 25 °C. At this temperature, the accumulation of Pb in coleoptile segments incubated in the presence of Pb was 60 and 34% higher in comparison to 20 and 30 °C, respectively (Table 1). Interestingly, the temperature had no effect on Pb content in maize coleoptile segments when Pb was added with IAA.
3.4 Relationships between the temperature of medium and metal accumulation or the elongation growth
The interrelations were assessed between the temperature of the medium (APW or APW + IAA) and the metal accumulation or the elongation growth of maize coleoptile segments (Table 2). High and positive correlations between the temperature of the medium (APW or APW + IAA) and Cd accumulation were observed. Positive correlations were also found between the temperature and Pb accumulation but they were low and statistically insignificant. The elongation growth of maize coleoptile segments was positively correlated with the temperature of medium irrespective of the presence or absence of IAA. In the presence of Pb or Cd, the correlation coefficient between the temperature and growth was high and positive although the maximal toxic effect of Cd on endogenous (35%) and IAA-induced growth (37%) was observed at 25 °C and the toxic effect of Pb on growth did not change with temperature (Table 2, Figs. 1B and 2B).
Pearson's correlation coefficients between the temperature of a medium (APW or APW + IAA) and a metal accumulation or the elongation growth of maize coleoptile segments.
Correlation coefficient | |||||
Cd accumulation | Pb accumulation | Growth without metals | Growth in the presence of Cd | Growth in the presence of Pb | |
Temperature of APW | 0.90* | 0.24 | 0.95* | 0.83* | 0.97* |
Temperature of APW + IAA | 0.84* | 0.10 | 0.79* | 0.82* | 0.81* |
4 Discussion
Monocot coleoptiles represent the classical model for studying auxin-dependent cell elongation because the growth of the coleoptiles only involves cell elongation. The data presented in this article clearly show that the elongation growth of maize coleoptile segments was positively correlated with temperature of the medium (Table 2) and both endogenous and IAA-induced growth of maize coleoptile segments was maximal at 30 °C (Figs. 1 and 2). These results are in line with our previous findings that the optimal temperature for the elongation of maize coleoptile segments falls in the range 30–35 °C [3,40]. It was also previously shown that the optimal temperatures for the germination and early growth of maize seedlings fall in similar (32–35 °C) temperature range [41].
As can be seen in Figs. 3 and 4, the increase in the temperature of the incubation medium of maize coleoptile segments from 20 to 30 °C enhanced proton extrusion. Similar to the elongation growth, the temperature optimum for proton extrusion was found at 30 °C. IAA added to the medium (after 2 h of preincubation) caused an enhancement of elongation growth and additional decrease of medium pH in comparison to the medium without growth substance. The growth and proton extrusion by maize coleoptile segments observed at 20, 25 and 30 °C in the presence of IAA (Figs. 2 and 4) were in good qualitative agreement with the results obtained with this model system by Karcz and Burdach [3,12]. These authors suggested that the temperature optima observed for endogenous growth and for growth in the presence of IAA, represent a balance between the temperature-dependent effects on metabolic pathways and respiratory rates.
The inhibition of plant growth is often found in the environmental tests for toxic heavy metals, although the mechanisms involved in these processes are still not completely understood [15,31,36,42]. Figs. 1 and 2 show the effect of temperature (20, 25 and 30 °C) on endogenous and IAA-induced growth of maize coleoptile segments incubated in the presence of Cd or Pb. It was shown that the growth of maize coleoptile segments at 20 °C was not significantly affected by Cd, whereas this metal showed its toxic effects when added to the incubation medium at 25 and 30 °C (Figs. 1 and 2). The maximal inhibition of endogenous and IAA-induced growth of segments (by 35 or 37%, respectively) was observed at 25 °C. In this temperature, Cd present in the incubation medium of coleoptile segments significantly suppressed proton extrusion compared to the medium without Cd (Figs. 3 and 4). Both the inhibition of elongation growth and proton extrusion in the presence of Cd are probably connected with the fact that cadmium, at least in part, inhibited the elongation growth of maize coleoptile segments by reduced the PM H+-ATPase activity as it was proposed by Karcz and Kurtyka [31] and Kurtyka et al. [18]. This suggestion is also supported by other authors who showed that Cd inhibited the PM H+-ATPase activity in sugar beet [43], cucumber [32], wheat, sunflower [23], oat [29] and maize [28,44]. It was also recently showed by Kabała et al. [45] that Cd ions diminished both ATP hydrolysis and proton transport in plasma membranes in cucumber root cells. These authors suggested that Cd-dependent inhibition of the PM H+-ATPase may be a result of direct metal action on the enzyme protein and/or a modification of membrane fatty acids [45].
The effects of temperature on metal toxicity, uptake and accumulation have been the subject of several studies. For example, Öncel et al. [14] and Qian et al. [46] observed that the increase of temperature tends to enhance the toxic effects of cadmium on wheat seedlings or Chlorella vulgaris growth, respectively. In contrast, in the current study, higher toxic effect of Cd on elongation growth was detected at 25 °C than at 30 °C (Figs. 1 and 2). This effect may be associated with both temperature-dependent metabolism and free auxin level in plant, since the elongation growth depends on both agents. The inhibition of Cd uptake and translocation of the metal from root to shoot was observed, when Arabidopsis thaliana plants were treated with low temperature or a metabolic inhibitor [47]. Similarly, in Solanum nigrum and Lolium perenne, the temperature-dependent Cd uptake were also detected by other authors ([39] and literature therein). In our studies, it was established that the content of Cd in maize coleoptile segments increased when the medium temperature was raised (Table 1) and that there is a high and positive correlation between the temperature of the medium and Cd accumulation, irrespective of the presence of IAA (Table 2).
On the other hand, Cd affects IAA content in plant [48]. Xu et al. [48] showed that 50 μM Cd treatment reduced the IAA content in roots of Medicago truncatula seedlings by about 50%. These authors found that Cd diminishes the level of IAA in roots by increasing the activity of IAA oxidase. Such increase in the activity of IAA oxidase was also determined in the leaves of Pisum sativum plants stressed with 100 μM Cd [37]. It was also previously shown that IAA oxidase activity strongly depends on temperature [49]. This enzyme activity was gradually increased with the increase of temperature with the maximum between 20 and 30 °C and it was obviously decreased at 30 °C. Thus, it is supposed that the maximum toxic effect of Cd on the elongation growth of a maize coleoptile segment observed here at 25 °C is caused by Cd-induced high activity of IAA oxidase. Although the accumulation of cadmium increased when the medium temperature was raised from 20 to 30 °C (Table 1), the maximum toxic effect of cadmium was observed at 25 °C. It is suggested that this phenomenon is much more dependent on Cd-induced increase of IAA oxidase activity than the temperature-dependent Cd accumulation.
In contrast to Cd, Pb (regardless of the medium temperature) diminished both endogenous and IAA-induced growth of maize coleoptile segments by ca. 20%) (Figs. 1 and 2). These results suggest that the toxic effect of Pb on elongation growth is not dependent on the temperature and the growth inhibition observed in the presence of Pb was accompanied by higher acidification of the medium compared to the control (medium without metals) (Figs. 3 and 4). This means that the toxic effect of Pb on elongation growth, in contrast to Cd, is not connected with the inhibition of the PM H+-ATPase activity. A negligible effect of Pb ions on the PM H+-ATPase activity was also observed by other authors [27,28,32].
According to Mukherji and Maitra [50], the toxic effect of Pb on growth of Oryza sativa seedlings is the result of an increase in IAA oxidase activity. However, these authors detected a higher activity of this enzyme after the addition of very high concentrations of Pb (equal to or higher than 2 × 10−2M). In the present study, the coleoptile segments were treated with Pb at a lower concentration (10−4 M), thus, it is proposed that the toxic effect of the metal on the elongation growth, in contrast to Cd, is not related with activity of IAA oxidase. It is supposed that the inhibition of elongation growth caused by Pb observed here was connected with other processes such as the lowering of the protein content in tissues or alterations in lipid composition of membranes as it was previously shown by Przymusiński et al. [51] and Stefanov et al. [21,22]. Interestingly, the accumulation of Pb in maize coleoptile segments was not correlated with temperature, whereas strong and positive correlation between the accumulation of Cd and the temperature was observed (Table 2). These results suggested that the accumulation of Pb does not depend on plant metabolism as it was found for Cd (Tables 1 and 2).
Taken together, these results suggest that endogenous and IAA-induced elongation growth as well as proton extrusion by maize coleoptile segments are positively correlated with the temperature and both depend on the PM H+-ATPase activity, which in turn is also temperature-dependent. Cd and Pb affected endogenous and IAA-induced elongation growth in maize coleoptile segments in a different manner. The toxic effect of Cd on elongation growth of coleoptile segments is connected with decrease of the PM H+-ATPase activity and probably with Cd-induced high activity of IAA oxidase, whereas the effect of Pb did not depend on the activity of any of the enzymes.
Disclosure of interest
The authors declare that they have no conflicts of interest concerning this article.