1 Introduction
During dehydration including the advanced state of thirst, numerous central and peripheral mechanisms are activated in human and other species in order to deal with, and adapt to this water imbalance, and which can take little or a lot of time depending on the species. Unlike the rat, Meriones shawi which is a semi-desert rodent, has particular ability to support a prolonged dehydration period that could reach over several months [1,2]; this rodent presents many characteristics and adaptations which allow it to survive in dry and hostile seasons. For example, nephrons of M. shawi are very developed, allowing it effectively to retain water by excretion of highly concentrated urine [3]. The study of desert animals allows us to understand many things about water balance and resistance to dehydration and therefore understanding some associated behaviours, mainly those related to the search for water and food by adjusting their locomotor activity. The water seeking behaviour is driven by the motivation of the animal to survive and therefore maintain the sustainability of the species; in this case, the dopamine (DA) systems with others work as a motivational agent [4]. The main sources of in cortical and subcortical areas of the brain are the DA-releasing neurons of the ventral midbrain, located especially in the substantia nigra pars compacta (SNpc) and ventral tegmental area (VTA) [5]. Axons departing from these two nuclei are assembled in the medial forebrain bundle (MFB). This system is connected to various other areas of the brain including the frontal cortex and particularly the accumbens nucleus and striatum [6,7]. The axons of MFB innervate the striatum in a precise somatotopy, the group of neurons located in the middle of the SNpc project directly to both the dorsal and the medial parts of the striatum, while neurons located laterally in the SNpc project too into the lateral and the ventral parts of striatum [8]. When the MFB axons are stimulated, they release DA from their axon tips into the synapse cleft of different target structures. The DA release in SNpc and VTA is made by two different modes, tonic and phasic [9,10]. In their tonic mode, DA neurons maintain stable the baseline level of DA in many neural structures that is vital for enabling the normal functions of neural circuits [11]. In their phasic mode, DA neurons suddenly and highly increase or decrease their firing rates for 100–500 ms, causing large changes in DA concentrations in brain structures and which persists for several seconds [11,12].
It is well established that DA is involved in the control of salt and water metabolism, since it stimulates the secretion of aldosterone and vasopressin which are involved in tubular sodium and water reabsorption [13,14]. Moreover, the implication of the nigro-striatal dopaminergic pathway in motor control is widely accepted, since the phasic release of DA originating from SNPC regulates the motor activity [15]. However, little is known about the effect of dehydration on the dopaminergic system originating from SNpc and VTA with their subsequent nigro-striatal outputs. Moreover, the effect of prolonged dehydration on locomotor activity remains obscure. Except for some old papers on the rat, no work has been done concerning the effect of this osmotic stress on desert species.
The objective of this study was to assess using an experimental open-field test and TH immunohistochemical localization, whether the dopaminergic system originating from SNpc and VTA with their outputs is implicated in locomotor activity behavior of M. shawi under the effects of prolonged water deprivation.
2 Material and methods
2.1 Animals
In the present study, we chose a particular rodent as an animal model of species that can resist long period of thirst. M. shawi seems to be a good example of these species since it presents the particularity to tolerate water deprivation which can reach several months [1,16]. Meriones are semi-desert rodents present in the desert regions of Marrakech and were kept in captivity in our breeding farm for several generations. For the experiment, our animals were aged 1 month of both sexes and were used for both behavioural and immunohistochemical studies with five animals in each group: group 1: controls (free access to water) and group 2: water dehydrated animals for 1 month. The rodents were housed at a constant room temperature (25 °C), with a 12-h dark–light cycle and free access to food for both study groups. Water bottles have been given freely to controls but not to water-deprived animals. All animals were treated in compliance according to guidelines of the Cadi Ayyad University, Marrakech (Morocco), and all efforts were made to minimize animal suffering and to reduce the number of animals used.
2.2 Open-field test
To assess the locomotor activity in our animals, we used the “open-field” test. As described by Erazi et al. [17], briefly it consists of a device of 25 identical squares made out of wood (20 cm per side). For the experiment, each animal was placed in the middle of the field and the ambulation (the number of squares traversed by animal) was recorded during 20 min.
2.3 Immunohistochemistry
Animals of each group were anesthetised with intraperitoneal (i.p.) injection of sodium pentobarbital (40 mg/kg), and perfused transcardially with chilled physiological saline and paraformaldehyde (4%) in phosphate buffer (PBS, 0.1 M, pH 7.4). Then, a frontal section of 20 μm was realized in the midbrain through substantia nigra (SN) and VTA (Bregma -5,3 mm), the MFB (Bregma 0,20 mm) and the caudate nucleus (Bregma 0,20 mm) with a microtome and collected in PBS. The slides were selected and preincubated during 2 h in PBS with 0.3% triton and 0.1% bovine serum albumin (BSA). Under agitation, the slides are then incubated overnight at 4 °C in a solution of anti-tyrosine hydroxylase (anti-TH: the key enzyme of catecholamine biosynthesis in the brain and the adrenals [18]) antibody (1/1000) containing PBS (0.1 M, pH 7.4), Triton (0.3%), and BSA (1%). The slides are then washed three times with PBS (0.1 M, pH 7.4) containing BSA (1%) for 5 min. The slices are then incubated with the secondary antibody (rabbit anti-immunoglobulins, 1/2000) for 2 h at room temperature. After three washes, the slides were incubated for 1 h 30 min in PBS buffer containing Triton (0.3%) and the Avidin-biotine peroxidase complex (Kit ABC, Vector; 1/500). TH was revealed following the enzymatic reaction of the peroxidase in presence of the 3,3′diaminobenzidine (DAB; Sigma, Oakville, Canada; 0.03%) and hydrogen peroxide (0.006%) in Tris buffer (0.05 M pH 7.5). The sections were then collected, dehydrated and mounted in Eukit for optical microscopy observation. The specificity of the immunoreactive materials was tested following subjection of the slides to the same immunohistochemical procedure as described above and using the pre-immune serum or omitting of the primary antibodies. These tests showed that the primary antibodies for TH display specific labelling.
2.4 Immunolabelling quantification and statistical analysis
TH immunoreactivity (TH-IR) quantification was performed according the protocol published by Vilaplana and Lavialle [19]. The digitization and storage of images were performed using a Zeiss-Axioskop 40 microscope equipped by a digital Canon camera. Images were digitized into 512/512 pixels with eight bits of grey resolution and were stored in TIFF format. Image processing and quantification were performed using Adobe Photoshop v.6.0.
After transformation of each image to the binary mode, the percentages of black pixels were obtained using the image histogram option of Adobe Photoshop. This percentage corresponds to the TH-immunopositive areas throughout SNpc. For controls and water-deprived animals, five sections were randomly chosen for the quantification of each group. Data are reported as mean ± SEM, results are expressed as mean ± SEM. The Student's t-test was used to analyze differences between groups. Values of P lower than 0.05 was considered significant. Statistical analyses were performed using the computer software SPSS 10.0 for Windows® (IBM, Chicago, IL, USA).
3 Results
3.1 Effect of dehydration on locomotor performance in M. shawi
Using the open-field apparatus to assess the effect of water deprivation of 1 month in M. shawi on the locomotor performance, our data demonstrated that dehydrated meriones exhibited a hyperactivity following 1 month of total water deprivation as compared to controls (Fig. 1).
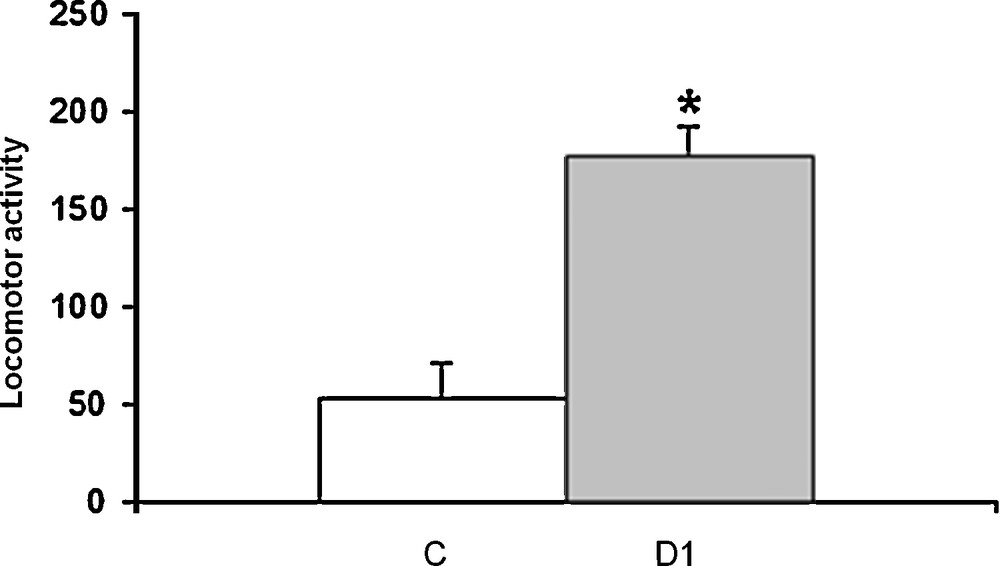
Histograms displaying the measurement of locomotor activity for 20 minutes in Meriones shawi using the open-field test. C: control; DH: Meriones dehydrated for 1 month. *P < 0.05 vs. controls.
3.2 Effect of dehydration on TH immunoreactivity in substantia nigra pars compacta (SNpc) and ventral tegmental area (VTA)
Our data showed in control meriones (Figs. 2A and 3A), the presence of many TH-immunoreactive neurons within both SNpc and VTA and their neuronal processes which extend within and out from these nuclei.
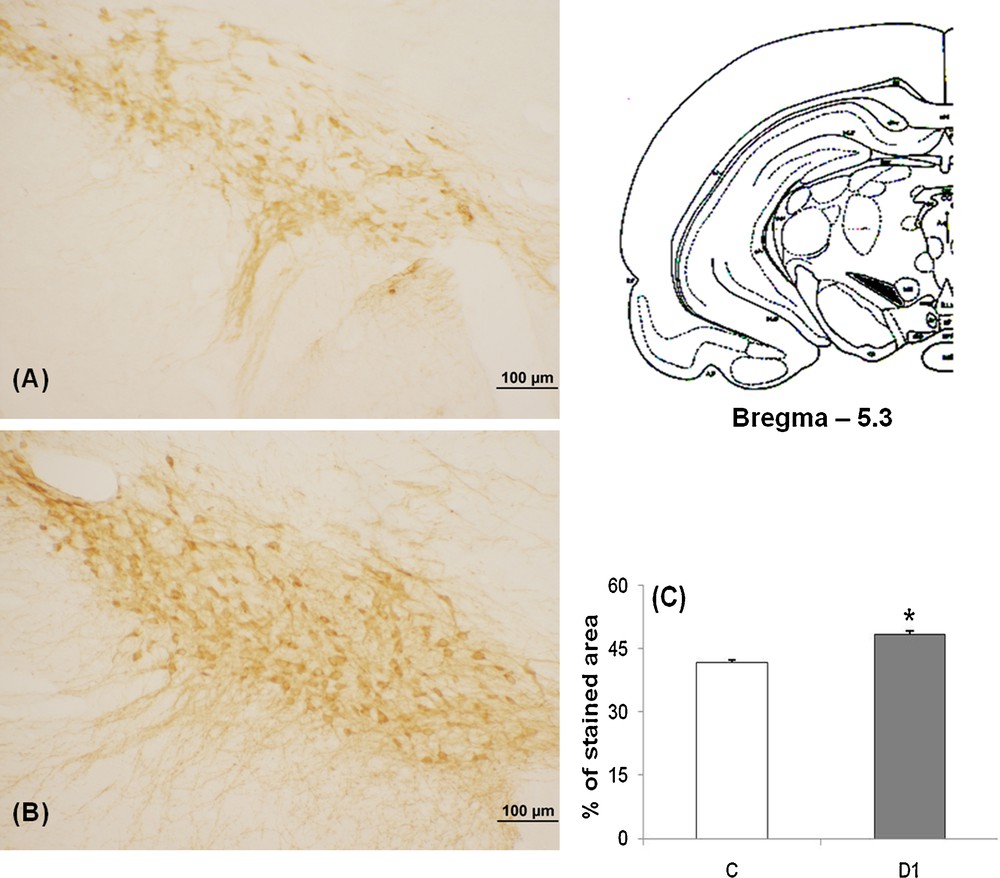
Light micrographs of frontal sections through SNpc of adult Meriones shawi, immunolabelled with antiserum against TH (A, B); in controls (A), after 1 month of water deprivation (B). C. Histogram represents the percentage areas of immunoreactivity of TH in SNpc in control and dehydrated for 1 month (D1). *P < 0.05 vs. controls. SNpc: Substancia nigra pars compacta.
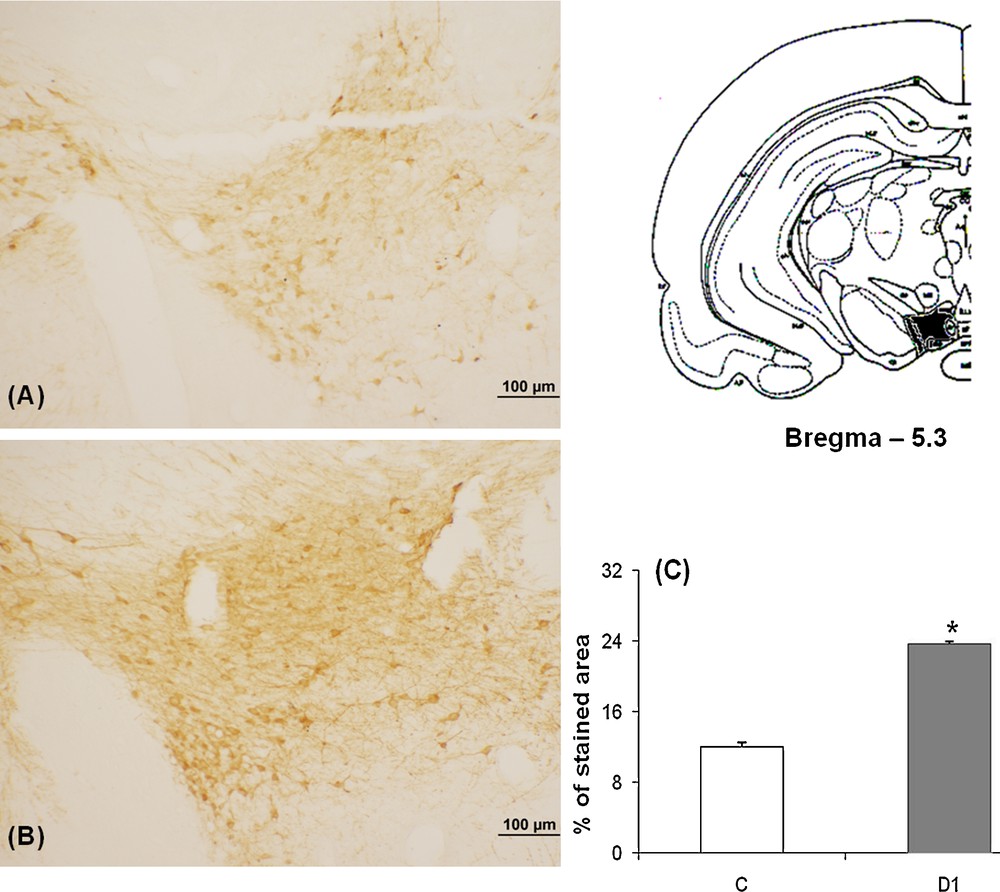
Light micrographs of frontal sections through VTA of adult Meriones shawi, immunolabelled with antiserum against TH (A, B); in controls (A), after 1 month of water deprivation (B). C. Histogram represents the percentage areas of immunoreactivity of TH in VTA in control and dehydrated for 1 month (D1). *P < 0.05 vs. controls. VTA: ventral tegmental area.
After 1 month dehydration (Figs. 2B and 3B), an obvious enhancement of the immunoreactivity was observed in the two nuclei; SNpc and VTA. TH neurons appeared strongly immunoreactive and very dense; the neuronal processes showed a rise of their immunoreactivity corresponding to a possible increase of the TH expression in the neuronal processes.
Quantification of the immunoreactive TH neurons in SNpc and VTA showed a statistically significant increase between controls and 1 month water-deprived group (Figs. 2C and 3C) [*: versus controls; P < 0.05].
3.3 Effect of dehydration on the nigro-striatal pathways: the medial forebrain bundle
To assess whether increased TH immunoreactivity within the SNpc nucleus occurring in dehydrated meriones is generalized to the subsequent projections, we were interested to the nigro-striatal pathways especially the MFB, indeed, our finding showed an increased TH expression in the MFB of dehydrated meriones for 1 month, TH-immunoreactive fibers are dense and constitute a mass of fibers with high size (Fig. 4B) as compared to controls in which the immunostaining is weak (Fig. 4A).
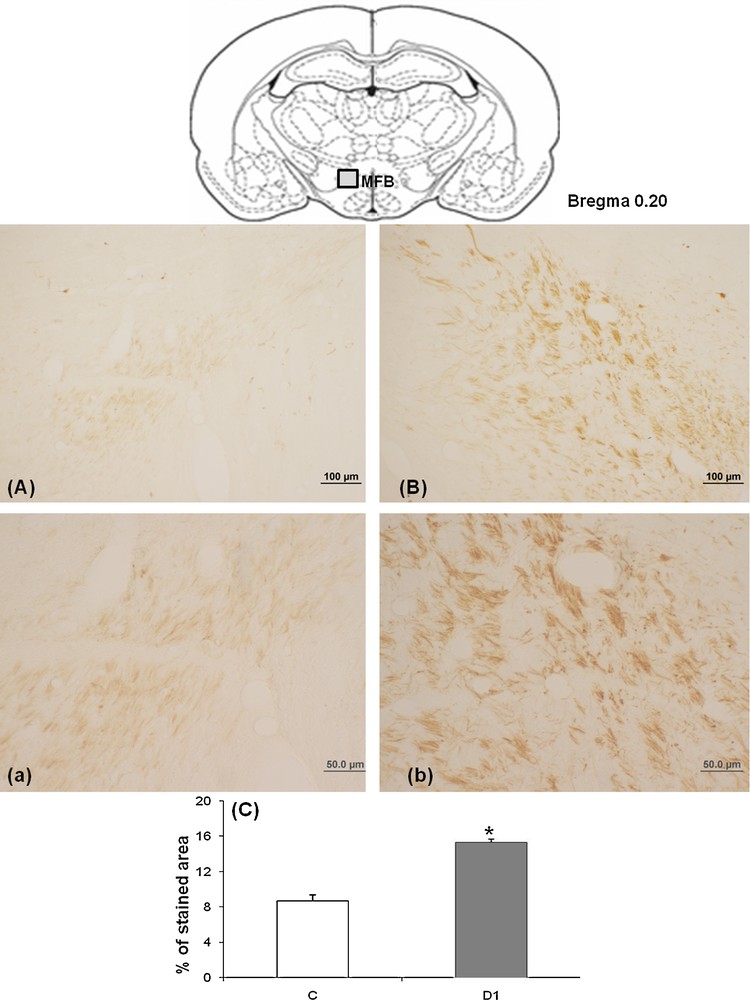
Light micrographs of frontal sections through MFB of adult Meriones shawi, immunolabelled with antiserum against TH (A, B); in controls (A), after 1 month of water deprivation (B). a–b: high magnification of MFB respectively in Meriones control and after 1 month of water deprivation. C. Histogram represents the percentage areas of immunoreactivity of TH in MFB in both control and dehydrated animals for 1 month (D1). *P < 0.05 vs. controls. MFB: medial forebrain bundle.
High magnification showed details of TH-immunoreactive fibers which appeared strongly immunoreactive in the dehydrated (Fig. 4Bb) versus control (Fig. 4Aa) meriones.
Quantification of the immunoreactive TH neurons in MFB showed a statistically significant increase between controls and 1 month water-deprived group (Fig. 4C) [*: versus controls; P < 0.05].
3.4 Effect of dehydration on the nigro-striatal projections within the caudate nucleus
To ascertain whether changes of the TH immunoreactivity in SNpc and VTA occurring during water deprivation are observed in other brain structures, we undertook an immunohistochemical study of the TH in the caudate putamen to describe the changes which occurred in the nigro-striatal projections after dehydration. Effectively, our findings showed, after 1 month of dehydration (Fig. 5B), a significant rise of the density of TH-immunoreactive fibers within the caudate putamen in the dehydrated meriones compared to their controls (Fig. 5A).
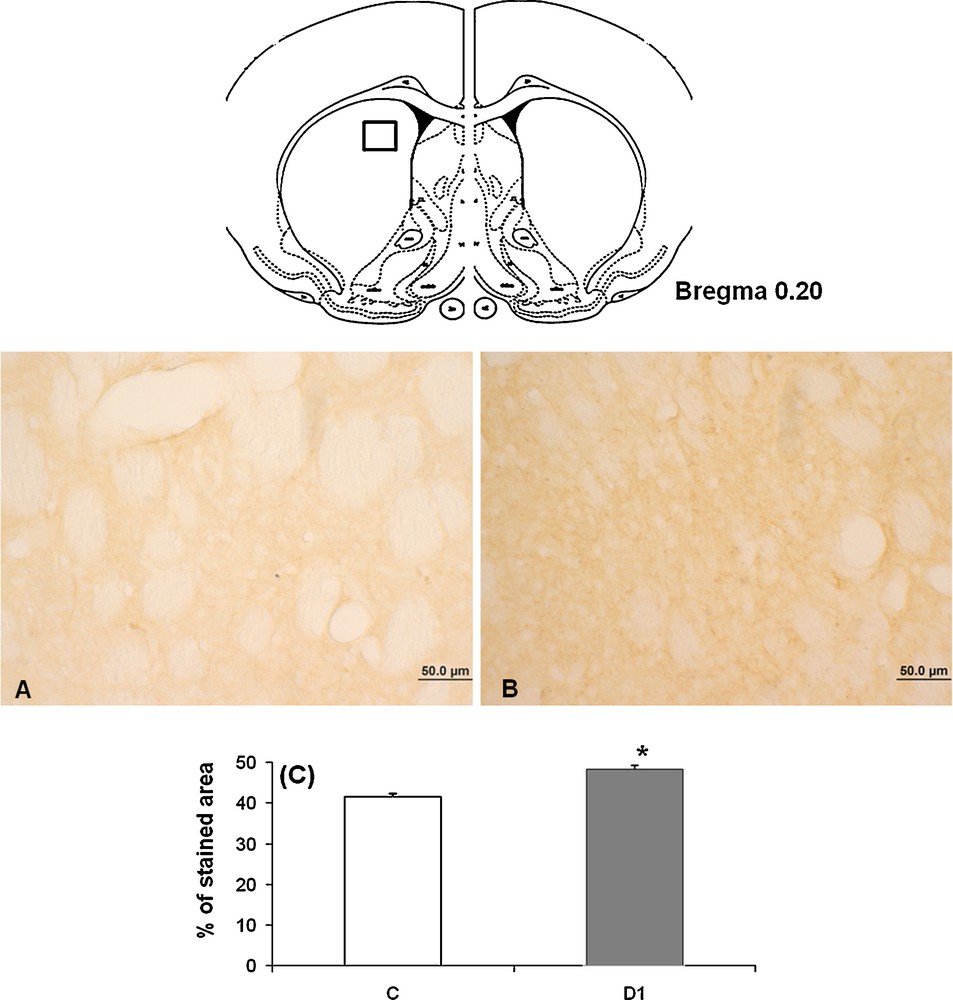
Light micrographs of frontal sections through striatum of adult Meriones shawi, immunolabelled with antiserum against TH (A, B); in controls (A), after 1 month of water deprivation (B). C. Histogram represents the percentage areas of immunoreactivity of TH in striatum in both controls and dehydrated animals for 1 month (D1). *P < 0.05 vs. controls. Squares: areas of magnification.
Quantification of the immunoreactive TH neurons in caudate nucleus showed a statistically significant increase between controls and 1 month water-deprived group (Fig. 5C) [*: versus controls; P < 0.05].
4 Discussion
Little is known about the effect of osmotic stress on the dopaminergic system involved in locomotor behavior; except for some old papers on the rat [20–24], no work has been done concerning the effect of prolonged water deprivation in the desert species. In our knowledge, the present work is the first in this field which aimed to assess the effect of a long period of water deprivation as an osmotic stress in a particular desert rodent studied for a long time in our laboratory. M. shawi is known to be a good animal model for studding the consequences of dehydration on the central nervous system, since it can tolerate thirst for long period reaching over several months [1,2,16]. It seems that this species has developed particular mechanisms for adaptation to its desert environment involving neuropeptids [16] as well as neurotransmitters [1]. Beside the implication of serotoninergic system in the response to dehydration [1], the dopaminergic system seems to be implicated too. Indeed, our finding demonstrated in the desert rodent M. shawi submitted to an osmotic stress by complete water restriction for 1 month, using the open-field test, an enhancement of locomotor performance as compared to control meriones, this was concomitant with a central rise of tyrosine hydroxylase (TH) in substantia nigra (SN) and VTA with their subsequent striatal outputs. Indeed, in a rat submitted to 23 hours of water restriction, using running wheels, the locomotor activity was enhanced [20,21] and this was concordant with other studies using circular stablimeter cages [22] while the use of rectangular stablimeters demonstrated an opposite result [23,24]. These conflicting findings may result in large part from differences in the type of apparatus used [25]. Otherwise, other studies using a Parkinson model in rat with striatal lesion via 6-OHDA, demonstrated a much greater increase of locomotor activity in dehydrated than in vehicle-treated rats. Moreover, the magnitude of increase in locomotor activity was inversely correlated with the magnitude of DA depletion in the caudate putamen, nucleus accumbens, septum and hypothalamus [26]. Previous studies have reported in rats submitted to 2 days of water deprivation, an increased DA level in the neural lobe of posterior pituitary regions containing terminals of tuberohypophysial [27].
Even in vitro, DA release was measured amperometrically from rat pheochromocytoma cells (PC12 cells: a neuroendocrine tumor of the medulla of the adrenal glands which secretes excessive amounts of catecholamines) in high osmolarity conditions and it has been reported an increase in the number of release events displaying a prespike feature or “foot” and the foot area and duration when the cells are stimulated in high osmolarity saline [28].
It is well established that DA is involved in the control of water intake behavior; thus, the mesolimbic DA system is involved in the ingestion of NaCl solutions. Indeed, in Na-depleted rats the intake of hypertonic NaCl solutions is accompanied by an increase in DA levels in the nucleus accumbens [14] and the preference for NaCl is reduced after lesioning of the VTA [29]. In situ hybridization and ligand-binding studies suggested an involvement of this system in the increased salt appetite in rats after Na depletion [30]. Moreover, in thirsty rats, the DA D1-like receptor antagonist SCH 23390 reduced the intake of water and hypotonic and isotonic NaCl solutions [31], while increasing the intake of hypertonic solutions, which, conversely, was reduced by administration of a DA D1-like receptor agonist [32]. In the same experimental conditions, the DA D2-like receptor antagonist sulpiride increased the intake of water and hypotonic and isotonic NaCl solutions [31].
Furthermore, much evidence has supported an obvious control of the arginine vasopressin (AVP) upon the dopaminergic system and vice versa [33–36]. Indeed, our previous study in M. shawi has demonstrated an increased arginine vasopressin immunoreactivity in both of the supraoptic and paraventricular nuclei which becomes significant from 1 month of total water deprivation [16], this neuropeptide has been recognized to interact with the nigro-striatal DA system at the level of the DA terminals in the nucleus caudatus rather than at the level of the substantia nigra by increasing the DA release by the nucleus caudatus rather than DA synthesis in DA neurons of substantia nigra [37].
5 Conclusion
The present investigation has shown in the desert rodent M. shawi, an obvious involvement of the DAergic system between substantia nigra and the nigro-striatal pathways in the response to osmotic challenge by prolonged water restriction, with an enhancement of DA immunoreactivity in both origin nuclei substantia nigra and VTA and their subsequent nigro-striatal outputs that may reflect on the locomotor behavior of the dehydrated meriones. The latter have shown hyperactivity after 1 month of water deprivation. This DA responsiveness observed may be one of the underlying mechanisms developed by this desert species beside others such as serotonin [1] and vasopressin [16] which interact to enable it to tolerate thirst over several months.
Disclosure of interest
The authors declare that they have no conflicts of interest concerning this article.
Acknowledgments
This work has been supported by OTAN (PDD (CP) - (LST.CLG979823) and CNRST (SC.Vie 05/10).