1 Introduction
Anopheles crawfordi Reid, 1953 belongs to the Lesteri Subgroup of the Hyrcanus Group within the Myzorhynchus Series of the subgenus Anopheles [1]. So far, the distribution of this anopheline species has been recorded from India (Assam), Thailand, Cambodia, Vietnam, peninsular Malaysia and Indonesia (Sumatra) [2,3]. Even though A. crawfordi could be found abundantly as a proven outdoor-biter of humans in certain localities of eastern and southern Thailand, its status as a vector of pathogens of human diseases remains obscure and needs to be investigated more intensively [2]. However, our recent experiments indicate that this anopheline species could be an important vector of the filarial nematode, nocturnally subperiodic Brugia malayi, as determined by 80–85% susceptibility rates and an average of six L3 larvae per infected mosquito [4]. These results were in agreement with previous investigation indicating that A. crawfordi could provide satisfactory susceptibility to B. malayi in Malaysia [5,6]. Additionally, A. crawfordi is considered an economic pest due to its vicious biting-behavior of cattle [1,2,5].
Cytogenetic investigations of A. crawfordi from two different localities in Thailand (eastern region: Chanthaburi Province; southern region: Phang Nga Province) were performed by Baimai et al. [7]. The results of their studies demonstrated that A. crawfordi exhibits genetic diversity at the chromosomal level, via a gradual increase in extra heterochromatin on the X and Y chromosomes. Two karyotypic variants (cytological forms), namely forms A (X1, Y1) and B (X2, Y2), were identified. The marked genetic variation of the X and Y chromosomes, as in other species of Anopheles, may indicate the existence of a species complex. The identical morphology or minimal morphological distinction among sibling species (isomorphic species) and subspecies (cytological races) within species complexes leads to difficulty in reliably identifying individual sibling species, which may differ in biological characteristics (e.g., microhabitats, resting and biting behaviors, sensitivity or resistance to insecticides, susceptibility or refractory character to pathogens, etc.) that may influence their vectorial capacity. Thus, inaccurate identification of individual members within a species complex may result in the failure to distinguish vector and non-vector species, and complicate vector control [8]. Although marked genetic variation at the chromosomal level of A. crawfordi has been observed, little is known about the genetics of chromosomal forms. This paper reports on the existence of two additional karyotypic forms of A. crawfordi and the results of cross-mating between the four karyotypic forms and comparisons of sequences for the second internal transcribed spacer (ITS2) of rDNA, and cytochrome c oxidase subunits I (COI) and II (COII) of mtDNA.
2 Materials and methods
2.1 Field collections and establishment of isoline colonies
Wild-caught, fully engorged females of A. crawfordi were collected from cow-baited traps at six locations in Thailand (Chiang Mai and Nan Provinces, northern region; Chumphon, Phang Nga, Trang and Songkhla Provinces, southern region), and two locations in Cambodia (Ratanakiri and Mondulkiri) (Fig. 1, Table 1). A total of 29 isolines were established and maintained using the techniques described by Choochote and Saeung [9]. The isolines were identified as A. crawfordi based on the morphology of the egg, larval, pupal, and adult stages of F1 progenies, using available keys [2,3,10]. The isolines were used for studies of the metaphase karyotype, cross-mating experiments and molecular analyses.
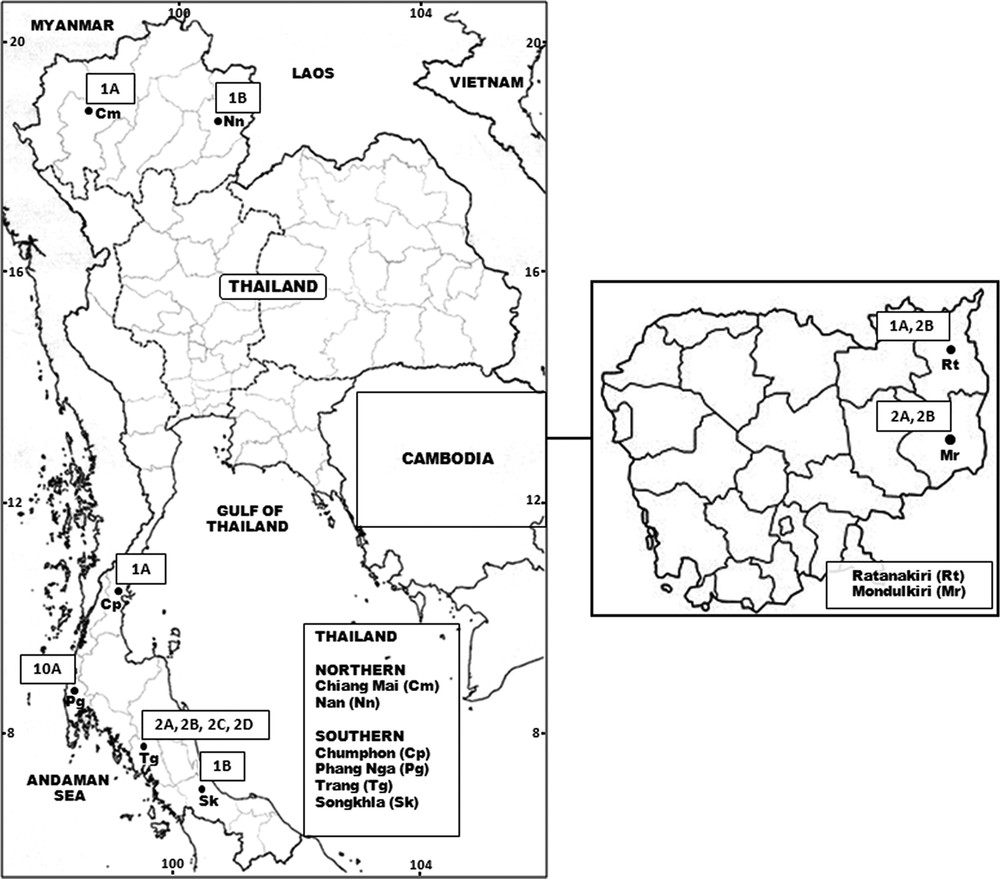
Maps of Thailand and Cambodia showing eight provinces where specimens of A. crawfordi were collected and the number of isolines of the four karyotypic forms (A–D) detected in each location.
Isolines of four karyotypic forms (A–D) of A. crawfordi and their GenBank accession numbers.
Location (geographical coordinate) |
Code of isolinea | Karyotypic form | GenBank accession number | Reference | ||
ITS2 | COI | COII | ||||
Thailand | ||||||
Chiang Mai (18° 47′ N, 98° 59′ E) |
Cm1Aa | A (X1, Y1) | AB779131 | AB779160 | AB779189 | This study |
Nan (19° 21′ N, 100° 39′ E) |
Nn1Ba | B (X1, Y2) | AB779132 | AB779161 | AB779190 | This study |
Chumphon (10° 29′ N, 99° 11′ E) |
Cp1A | A (X3, Y1) | AB779133 | AB779162 | AB779191 | This study |
Trang (07° 33′ N, 99° 38′ E) |
Tg1Ba | B (X3, Y2) | AB779134 | AB779163 | AB779192 | This study |
Tg2Ca | C (X2, Y3) | AB779135 | AB779164 | AB779193 | This study | |
Tg3Aa | A (X3, Y1) | AB779136 | AB779165 | AB779194 | This study | |
Tg4Da | D (X2, Y4) | AB779137 | AB779166 | AB779195 | This study | |
Tg6B | B (X2, Y2) | AB779138 | AB779167 | AB779196 | This study | |
Tg8D | D (X2, Y4) | AB779139 | AB779168 | AB779197 | This study | |
Tg11A | A (X2, Y1) | AB779140 | AB779169 | AB779198 | This study | |
Tg12C | C (X2, Y3) | AB779141 | AB779170 | AB779199 | This study | |
Phang Nga (08° 27′ N, 98° 31′ E) |
Pg4A | A (X1, Y1) | AB779142 | AB779171 | AB779200 | This study |
Pg5Aa | A (X2, Y1) | AB779143 | AB779172 | AB779201 | This study | |
Pg6A | A (X1, Y1) | AB779144 | AB779173 | AB779202 | This study | |
Pg7A | A (X2, Y1) | AB779145 | AB779174 | AB779203 | This study | |
Pg8A | A (X2, Y1) | AB779146 | AB779175 | AB779204 | This study | |
Pg9A | A (X2, Y1) | AB779147 | AB779176 | AB779205 | This study | |
Pg11A | A (X2, Y1) | AB779148 | AB779177 | AB779206 | This study | |
Pg12A | A (X1, Y1) | AB779149 | AB779178 | AB779207 | This study | |
Pg14A | A (X1, Y1) | AB779150 | AB779179 | AB779208 | This study | |
Pg16A | A (X2, Y1) | AB779151 | AB779180 | AB779209 | This study | |
Songkhla (07° 13′ N, 100° 37′ E) |
Sk1Ba | B (X3, Y2) | AB779152 | AB779181 | AB779210 | This study |
Cambodia | ||||||
Ratanakiri (13° 44′ N, 107° 0′ E) |
Rt1Aa | A (X1, Y1) | AB779153 | AB779182 | AB779211 | This study |
Rt2B | B (X2, Y2) | AB779154 | AB779183 | AB779212 | This study | |
Rt3B | B (X2, Y2) | AB779155 | AB779184 | AB779213 | This study | |
Mondulkiri (12° 27′ N, 107° 14′ E) |
Mr1Ba | B (X2, Y2) | AB779156 | AB779185 | AB779214 | This study |
Mr2A | A (X2, Y1) | AB779157 | AB779186 | AB779215 | This study | |
Mr3A | A (X1, Y1) | AB779158 | AB779187 | AB779216 | This study | |
Mr4B | A (X2, Y2) | AB779159 | AB779188 | AB779217 | This study | |
Vietnam | ||||||
3.6 | – | – | KF431868 | KF431892 | Ngo et al. [49] | |
269 | – | – | KF431873 | KF431896 | Ngo et al. [49] | |
286 | – | – | KF431865 | KF431889 | Ngo et al. [49] | |
302 | – | – | KF431881 | KF431902 | Ngo et al. [49] | |
A. belenrae | – | – | EU789794 | – | – | Park et al. [38] |
A. kleini | – | – | EU789793 | – | – | Park et al. [38] |
A. lesteri | – | – | EU789791 | – | – | Park et al. [38] |
ilG1 | – | – | AB733028 | AB733036 | Taai et al. [50] | |
A. paraliae | Sk1B | B (X1, Y2) | AB733487 | AB733503 | AB733519 | Taai et al. [40] |
A. peditaeniatus | RbB | B (X3, Y2) | AB539061 | AB539069 | AB539077 | Choochote [31] |
A. pullus | – | – | EU789792 | – | – | Park et al. [38] |
– | – | – | AY444348 | AY444347 | Park et al. [35] | |
A. sinensis | i2ACM | A (X, Y1) | AY130473 | – | – | Min et al. [37] |
– | – | – | AY444351 | – | Park et al. [35] | |
i1BKR | B (X, Y2) | – | – | AY130464 | Min et al. [37] |
a Used in crossing experiments.
2.2 Metaphase karyotype preparation
Metaphase chromosomes were prepared from 10 early fourth-instar larval brains of F1 progenies of each isoline, using techniques previously described by Saeung et al. [11]. Identification of karyotypic forms followed the standard cytotaxonomic systems of Baimai et al. [7].
2.3 Cross-mating experiments
The ten laboratory-raised isolines of A. crawfordi were selected arbitrarily from the 29 isoline colonies, which were representative of four karyotypic forms, i.e., form A [Cm1A (X1, Y1), Tg3A (X3, Y1), Pg5A (X2, Y1), Rt1A (X1, Y1)], form B [Nn1B (X1, Y2), Tg1B (X3, Y2), Sk1B (X3, Y2), Mr1B (X2, Y2)], form C [Tg2C (X2, Y3)], and form D [Tg4D (X2, Y4)] (Table 1). These isolines were used for cross-mating experiments to determine post-mating barriers by employing the techniques previously reported by Saeung et al. [11].
2.4 DNA extraction and PCR amplification
Total genomic DNA was isolated from individual F1 progeny adult female of each isoline (Table 1) using the DNeasy® Blood and Tissue Kit (QIAGEN). Primers for amplification of the ITS2, COI, and COII regions followed previous studies by Saeung et al. [11]. The ITS2 region of rDNA was amplified using primers ITS2A (5′-TGT GAA CTG CAG GAC ACA T-3′) and ITS2B (5′-TAT GCT TAA ATT CAGGGGGT-3′) [12]. The 709-bp fragment of the mitochondrial COI barcoding region was amplified using the LCO1490 (5′-GGT CAA CAA ATC ATA AAG ATA TTG G-3′) and HCO2198 (5′-TAA ACT TCA GGG TGA CCA AAA AAT CA-3′) primers of Folmer et al. [13]. The mitochondrial COII region was amplified using primers LEU (5′-TCT AAT ATG GCA GAT TAG TGC A-3′) and LYS (5′-ACT TGC TTT CAG TCA TCT AAT G-3′) [14]. Each PCR reaction was carried out in 20 μL containing 0.5 U Ex Taq (Takara), 1X Ex Taq buffer, 2 mM of MgCl2, 0.2 mM of each dNTP, 0.25 μM of each primer, and 1 μL of the extracted DNA. For ITS2, PCR consisted of initial denaturation at 94 °C for 1 min, 30 cycles at 94 °C for 30 s, 55 °C for 30 s, and 72 °C for 1 min, and a final extension at 72 °C for 5 min. The amplification profile of COI and COII comprised initial denaturation at 94 °C for 1 min, 30 cycles at 94 °C for 30 s, 50 °C for 30 s, and 72 °C for 1 min, and a final extension at 72 °C for 5 min. The amplified products were electrophoresed in 1.5% agarose gels and stained with ethidium bromide. Finally, the amplicons were purified using the QIAquick® PCR Purification Kit (QIAGEN). The PCR products were sequenced in both directions using the BigDye® V3.1 Terminator Cycle Sequencing Kit and 3130 genetic analyzer (Applied Biosystems).
2.5 Sequencing alignment and phylogenetic analysis
Sequences were aligned using the CLUSTAL W multiple alignment program [15] and edited manually in BioEdit version 7.0.5.3 [16]. All positions containing gaps and missing data were excluded from the analysis. The Kimura two-parameter (K2P) model was employed to calculate genetic distances [17]. Using the distances, construction of neighbor-joining trees [18] and the bootstrap test with 1000 replications were performed with Molecular Evolutionary Genetics Analysis (MEGA) version 6.0 [19]. Bayesian analysis was conducted with MrBayes 3.2 [20] by using two replicates of 1 million generations with the nucleotide evolutionary model. The best-fit model was chosen for each gene separately using the Akaike Information Criterion (AIC) in Mr Model test version 2.3 [21]. The general time-reversible (GTR) with gamma distribution shape parameter (G) was selected for ITS2, whereas the GTR + I + G was the best-fit model for COI and COII. Bayesian posterior probabilities were calculated from the consensus tree after excluding the first 25% trees as burn-in. Available sequences of the Hyrcanus Group were retrieved from GenBank using BLAST (http://blast.ncbi.nlm.nih.gov/Blast.cgi) for performing the phylogenetic analysis with our sequences (Table 1).
3 Results
3.1 Metaphase karyotypes
Cytological observations of F1 progenies of 29 isolines of A. crawfordi demonstrated distinct types of sex chromosomes due to the addition of extra heterochromatin. There were three types of X (metacentric X1, submetacentric X2, and large submetacentric X3) and four types of Y chromosomes (small telocentric Y1, large subtelocentric Y2, small subtelocentric Y3, and submetacentric Y4) (Fig. 2). These types of X and Y chromosomes comprised four forms of metaphase karyotypes on the basis of Y chromosome configurations, designated as forms A (X1, X2, X3, Y1), B (X1, X2, X3, Y2), C (X2,Y3), and D (X2, Y4). The number of isolines of these karyotypic forms occurring in different localities in six and two provinces of Thailand and Cambodia, respectively, are illustrated in Fig. 1 and Table 1. Forms C and D are new metaphase karyotypes discovered in the present study. Forms A and B appeared to be common in both Thailand and Cambodia, whereas forms C and D were confined to Trang Province, southern Thailand.
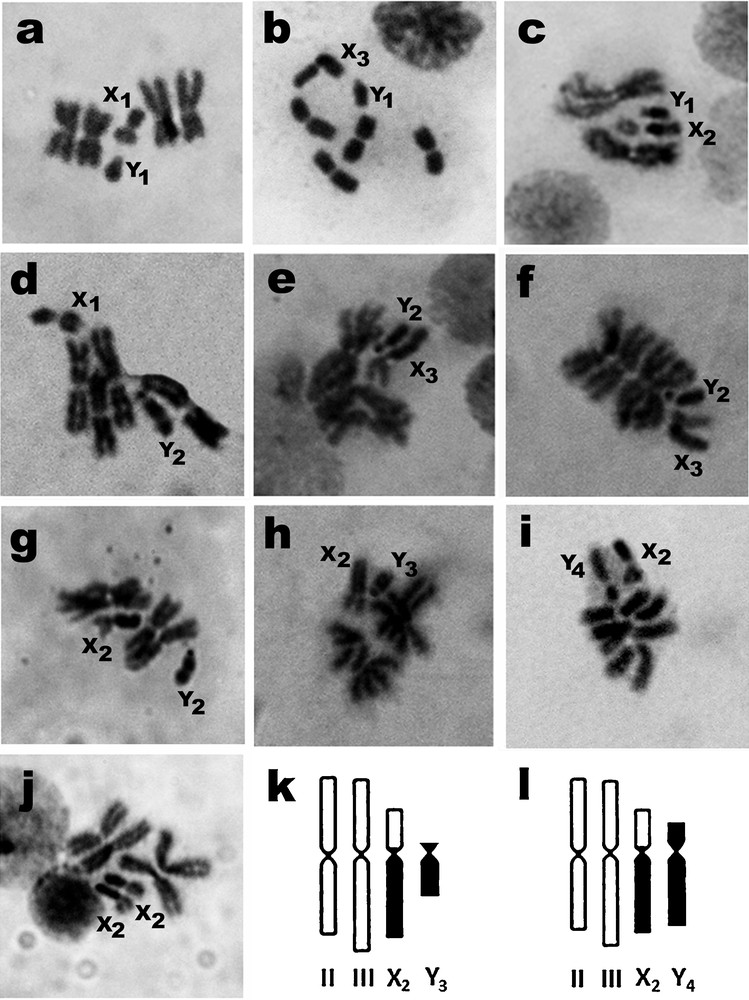
Metaphase karyotypes of A. crawfordi: a: form A (X1, Y1: Chiang Mai); b: form A (X3, Y1: Chumphon); c: form A (X2, Y1: Trang); d: form B (X1, Y2: Nan); e: form B (X3, Y2: Trang); f: form B (X3, Y2: Songkhla); g: form B (X2, Y2: Ratanakiri); h: form C (X2, Y3: Trang); i: form D (X2, Y4: Trang); j: form B (homozygous X2, X2: Mondulkiri); diagrams of representative metaphase karyotypes of form C (k) and form D (l).
3.2 Cross-mating experiments
Details of hatchability, pupation, emergence and adult sex ratio of parental, reciprocal and F1-hybrid crosses among the 10 isolines of A. crawfordi representing forms A–D are shown in Table 2. All crosses yielded viable progeny through the F2 generations. No evidence of genetic incompatibility and/or post-mating reproductive isolation was observed among these crosses. The salivary gland polytene chromosomes of the fourth-instar larvae of F1-hybrids from all crosses showed synapsis without inversion loops along the whole length of all autosomes and the X chromosome (Fig. 3).
Cross-mating experiments of 10 isolines of A. crawfordi.
Crosses (female × male) |
Total eggs (number)a |
Embryonation rateb |
Hatched n (%) |
Pupation n (%) |
Emergence n (%) |
Total emergence n (%) | |
Female | Male | ||||||
Parental cross | |||||||
Cm1A × Cm1A | 309 (179, 130) | 90 | 272 (88.03) | 258 (94.85) | 248 (96.12) | 111 (44.76) | 137 (55.24) |
Nn1B × Nn1B | 251 (141, 110) | 87 | 208 (82.87) | 200 (96.15) | 200 (100.00) | 88 (44.00) | 112 (56.00) |
Tg3A × Tg3A | 395 (166, 229) | 92 | 348 (88.10) | 317 (91.09) | 311 (98.11) | 162 (52.09) | 149 (47.91) |
Tg1B × Tg1B | 413 (234, 179) | 79 | 326 (78.93) | 293 (89.88) | 287 (97.95) | 149 (51.92) | 138 (48.08) |
Tg2C × Tg2C | 314 (200, 114) | 85 | 264 (84.08) | 259 (98.11) | 256 (98.84) | 111 (43.36) | 145 (56.64) |
Tg4D × Tg4D | 228 (123, 105) | 83 | 185 (81.14) | 183 (98.92) | 183 (100.00) | 93 (50.82) | 90 (49.18) |
Pg5A × Pg5A | 326 (146, 180) | 88 | 284 (87.12) | 281 (98.94) | 278 (98.93) | 138 (49.64) | 140 (50.36) |
Sk1B × Sk1B | 269 (103, 166) | 97 | 261 (97.03) | 256 (98.08) | 251 (98.05) | 118 (47.01) | 133 (52.99) |
Rt1A × Rt1A | 254 (156, 98) | 93 | 236 (92.91) | 231 (97.88) | 229 (99.13) | 127 (55.46) | 102 (44.54) |
Mr1B × Mr1B | 269 (175, 94) | 88 | 237 (88.10) | 232 (97.89) | 230 (99.14) | 112 (48.70) | 118 (51.30) |
Reciprocal cross | |||||||
Cm1A × Nn1B | 360 (217, 143) | 80 | 284 (78.89) | 281 (98.94) | 281 (100.00) | 132 (46.98) | 149 (53.02) |
Nn1B × Cm1A | 283 (105, 178) | 93 | 252 (89.05) | 252 (100.00) | 252 (100.00) | 111 (44.05) | 141 (55.95) |
Cm1A × Tg3A | 232 (146, 86) | 94 | 204 (87.93) | 200 (98.04) | 196 (98.00) | 114 (58.16) | 82 (41.84) |
Tg3A × Cm1A | 258 (129, 129) | 92 | 235 (91.09) | 230 (97.87) | 228 (99.13) | 108 (47.37) | 120 (52.63) |
Cm1A × Tg1B | 269 (151, 118) | 90 | 221 (82.16) | 217 (98.19) | 213 (98.16) | 96 (45.07) | 117 (54.93) |
Tg1B × Cm1A | 278 (113, 165) | 93 | 256 (92.09) | 246 (96.09) | 239 (97.15) | 126 (52.72) | 113 (47.28) |
Cm1A × Tg2C | 320 (134, 186) | 95 | 282 (88.13) | 282 (100.00) | 282 (100.00) | 149 (52.84) | 133 (47.16) |
Tg2C × Cm1A | 337 (179, 158) | 96 | 313 (92.88) | 285 (91.05) | 242 (84.91) | 117 (48.35) | 125 (51.65) |
Cm1A × Tg4D | 280 (120, 160) | 90 | 252 (90.00) | 232 (92.06) | 230 (99.14) | 112 (48.70) | 118 (51.30) |
Tg4D × Cm1A | 282 (113, 169) | 88 | 240 (85.11) | 200 (83.33) | 196 (98.00) | 102 (52.04) | 94 (47.96) |
Cm1A × Pg5A | 255 (138, 117) | 95 | 242 (94.90) | 242 (100.00) | 230 (95.04) | 97 (42.17) | 133 (57.83) |
Pg5A × Cm1A | 260 (160, 100) | 96 | 247 (95.00) | 232 (93.93) | 216 (93.10) | 111 (51.39) | 105 (48.61) |
Cm1A × Sk1B | 296 (170,126) | 95 | 281 (94.93) | 281 (100.00) | 275 (97.86) | 138 (50.18) | 137 (49.82) |
Sk1B × Cm1A | 333 (160, 173) | 90 | 290 (87.09) | 258 (88.97) | 201 (77.91) | 104 (51.74) | 97 (48.26) |
Cm1A × Rt1A | 263 (145, 118) | 94 | 247 (93.92) | 230 (93.12) | 230 (100.00) | 121 (52.61) | 109 (47.39) |
Rt1A × Cm1A | 277 (163, 114) | 92 | 255 (92.06) | 247 (96.86) | 230 (93.12) | 118 (51.30) | 112 (48.70) |
Cm1A × Mr1B | 287 (109, 178) | 87 | 227 (79.09) | 209 (92.07) | 209 (100.00) | 102 (48.80) | 107 (51.20) |
Mr1B × Cm1A | 308 (194, 114) | 78 | 234 (75.97) | 234 (100.00) | 234 (100.00) | 113 (48.29) | 121 (51.71) |
F 1 -hybrid cross | |||||||
(Cm1A × Nn1B)F1 × (Cm1A × Nn1B)F1 | 320 (136, 184) | 86 | 243 (75.94) | 221 (90.95) | 221 (100.00) | 104 (47.06) | 117 (52.94) |
(Nn1B × Cm1A)F1x (Nn1B × Cm1A)F1 | 357 (168, 189) | 91 | 300 (84.03) | 267 (89.00) | 267 (100.00) | 134 (50.19) | 133 (49.81) |
(Cm1A × Tg3A)F1 × (Cm1A × Tg3A)F1 | 296 (169, 127) | 80 | 216 (72.97) | 216 (100.00) | 207 (95.83) | 101 (48.79) | 106 (51.21) |
(Tg3A xCm1A)F1 × (Tg3A × Cm1A)F1 | 325 (126, 199) | 87 | 260 (80.00) | 257 (98.85) | 257 (100.00) | 131 (50.97) | 126 (49.03) |
(Cm1A × Tg1B)F1 × (Cm1A × Tg1B)F1 | 235 (108, 127) | 91 | 207 (88.09) | 207 (100.00) | 205 (99.03) | 86 (41.95) | 119 (58.05) |
(Tg1B × Cm1A)F1 × (Tg1B × Cm1A)F1 | 252 (145, 107) | 84 | 171 (67.86) | 169 (98.83) | 166 (98.22) | 86 (51.81) | 80 (48.19) |
(Cm1A × Tg2C)F1 × (Cm1A × Tg2C)F1 | 318 (131, 187) | 83 | 261 (82.08) | 261 (100.00) | 253 (96.93) | 121 (47.83) | 132 (52.17) |
(Tg2C × Cm1A)F1 × (Tg2C × Cm1A)F1 | 354 (164, 190) | 85 | 290 (81.92) | 287 (98.97) | 276 (96.17) | 132 (47.83) | 144 (52.17) |
(Cm1A × Tg4D)F1 × (Cm1A × Tg4D)F1 | 263 (188, 75) | 80 | 200 (76.05) | 182 (91.00) | 180 (98.90) | 86 (47.78) | 94 (52.22) |
(Tg4D × Cm1A)F1 × (Tg4D × Cm1A)F1 | 250 (150, 100) | 97 | 212 (84.80) | 212 (100.00) | 210 (99.06) | 116 (55.24) | 94 (44.76) |
(Cm1A × Pg5A)F1 × (Cm1A × Pg5A)F1 | 265 (126, 139) | 91 | 230 (86.79) | 230 (100.00) | 225 (97.83) | 106 (47.11) | 119 (52.89) |
(Pg5A × Cm1A)F1 × (Pg5A × Cm1A)F1 | 250 (102, 148) | 88 | 195 (78.00) | 183 (93.85) | 172 (93.99) | 86 (50.00) | 86 (50.00) |
(Cm1A × Sk1B)F1 × (Cm1A × Sk1B)F1 | 336 (136, 200) | 85 | 269 (80.06) | 269 (100.00) | 269 (100.00) | 110 (40.89) | 159 (59.11) |
(Sk1B × Cm1A)F1 × (Sk1B × Cm1A)F1 | 320 (162, 158) | 92 | 269 (84.06) | 269 (100.00) | 269 (100.00) | 134 (49.81) | 135 (50.19) |
(Cm1A × Rt1A)F1 × (Cm1A × Rt1A)F1 | 227 (148, 79) | 84 | 154 (67.84) | 140 (90.91) | 137 (97.86) | 66 (48.18) | 71 (51.82) |
(Rt1A × Cm1A)F1 × (Rt1A × Cm1A)F1 | 235 (108, 127) | 97 | 218 (92.77) | 218 (100.00) | 218 (100.00) | 116 (53.21) | 102 (46.79) |
(Cm1A × Mr1B)F1 × (Cm1A × Mr1B)F1 | 268 (159, 109) | 79 | 204 (76.12) | 204 (100.00) | 204 (100.00) | 102 (50.00) | 102 (50.00) |
(Mr1B × Cm1A)F1 × (Mr1B × Cm1A)F1 | 245 (100, 145) | 65 | 152 (62.04) | 150 (98.68) | 147 (98.00) | 69 (46.94) | 78 (53.06) |
a Two selective egg-batches of inseminated females from each cross.
b Dissection from 100 eggs.
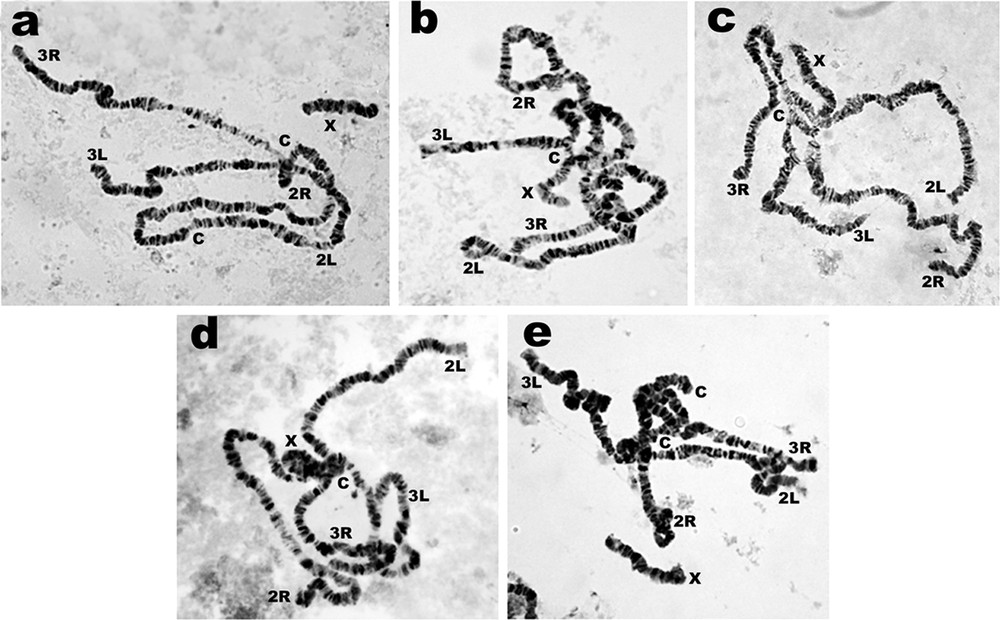
Complete synapsis in all arms of salivary gland polytene chromosome of F1-hybrid larvae of A. crawfordi: a: Cm1A female × Sk1B male; b: Cm1A female × Tg2 C male; c: Cm1A female × Tg4D male; d: Cm1A female × Rt1A male; e: Cm1A female × Mr1B male.
3.3 DNA sequences and phylogenetic analysis
All sequences generated from the 29 isolines are available in the DDBJ/EMBL/GenBank nucleotide sequence database under accession numbers AB779131-AB779217 (Table 1). The length of the ITS2 region ranged from 446 to 449 bp in seven and 22 isolines from Cambodia and Thailand, respectively. A. crawfordi from both provinces of Cambodia differed from A. crawfordi in Thailand by a deletion of T, C, and T at positions 21, 280, and 292, respectively. However, they all showed the same length for COI (658 bp, excluding primers) and COII (685 bp) sequences. The evolutionary relationships among the four karyotypic forms were determined using neighbor-joining (NJ) and Bayesian analysis. Both phylogenetic methods showed similar tree topologies, thus, only the Bayesian tree is shown in Figs. 4–6. All 29 isolines were placed within the same cluster and well separated from other species of the Anopheles hyrcanus group (Anopheles belenrae, Anopheles kleini, Anopheles lesteri, Anopheles paraliae, Anopheles peditaeniatus, Anopheles pullus and Anopheles sinensis). The mean intra-specific sequence divergences within (0.000–0.018) and between (0.000–0.016) the four karyotypic forms are not significantly different for the DNA regions (Table 3). In addition, COI and COII sequences of A. crawfordi from Vietnam (Table 1) formed the clade with our sequences with high support (NJ = 82–100%, BPP = 100%, Figs. 5–6). The low mean genetic distance among specimens examined for COI (0.017) and COII (0.011) genes were good supportive evidence of a single species.
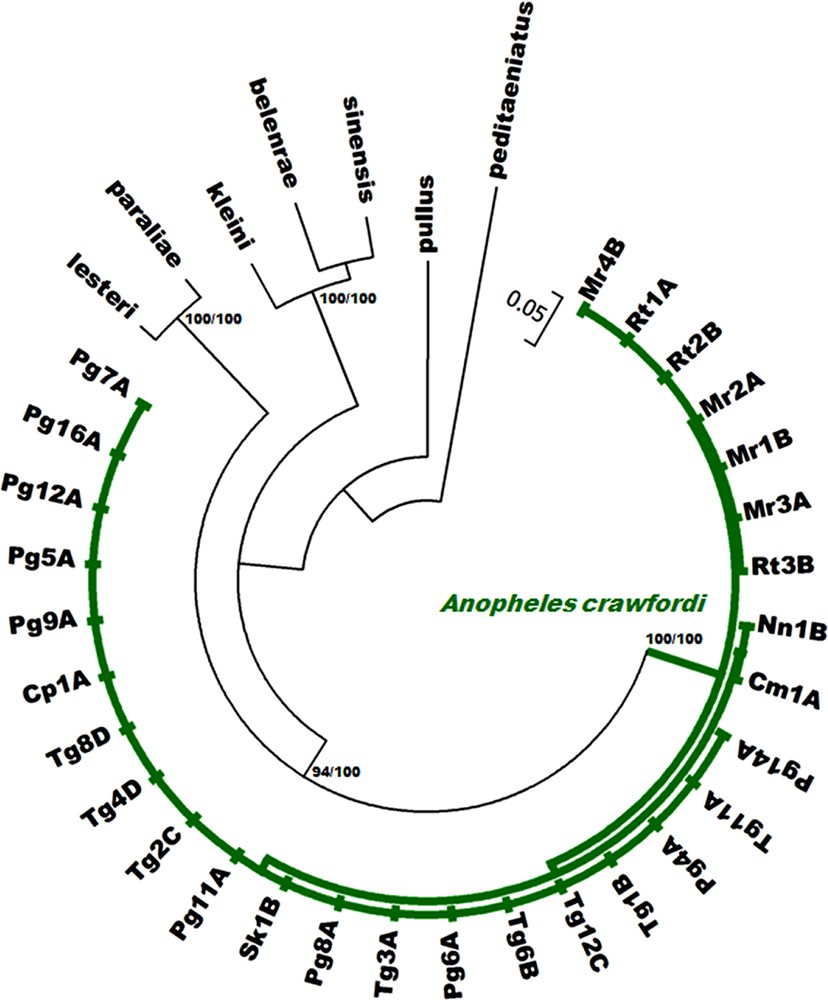
Phylogenetic relationships of A. crawfordi from Thailand and Cambodia using Bayesian analysis based on ITS2 sequences compared with seven species of the Hyrcanus Group. Codes for the specimens are shown in Table 1. Numbers on branches are bootstrap values (%) of NJ analysis and Bayesian posterior probabilities (%). Only the values higher than 50% are shown. Bars represent 0.05 substitutions per site.
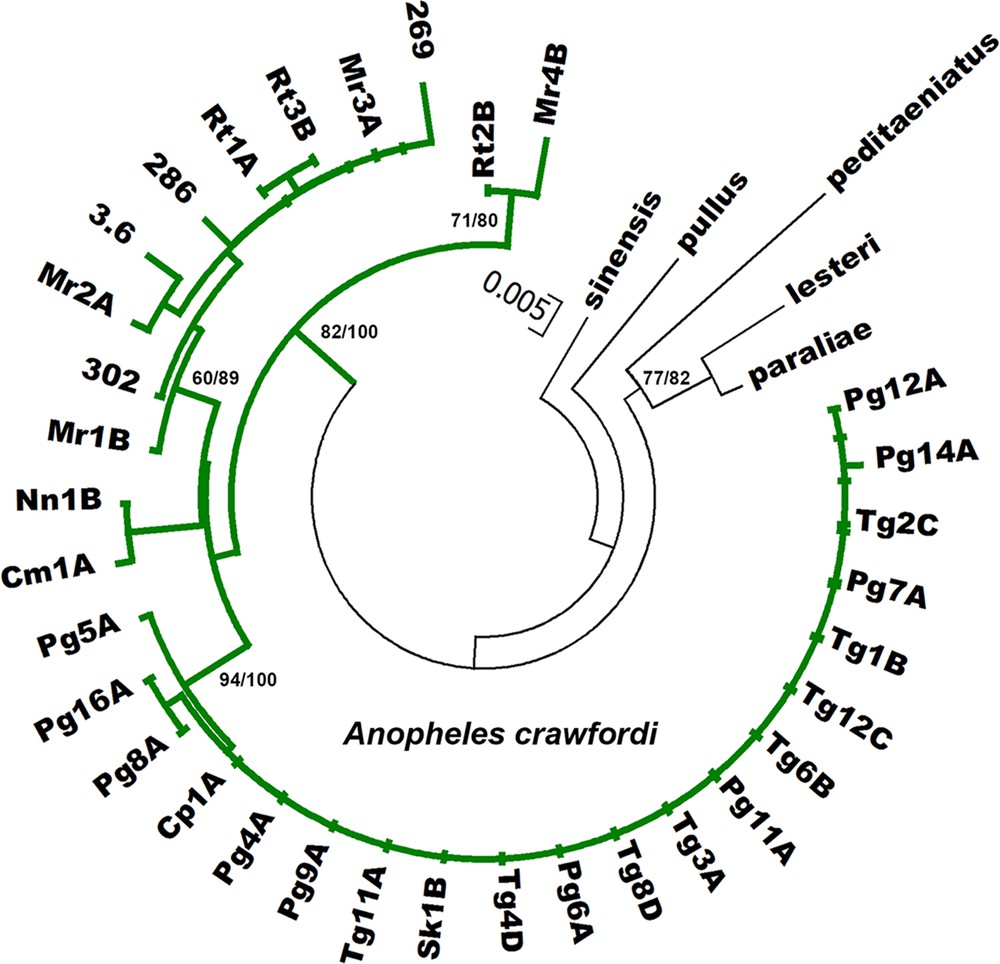
Phylogenetic relationships of A. crawfordi from Thailand, Cambodia, and Vietnam using Bayesian analysis based on COI sequences compared with five species of the Hyrcanus Group. Codes for the specimens are shown in Table 1. Numbers on branches are bootstrap values (%) of NJ analysis and Bayesian posterior probabilities (%). Only the values higher than 50% are shown. Bars represent 0.005 substitutions per site.
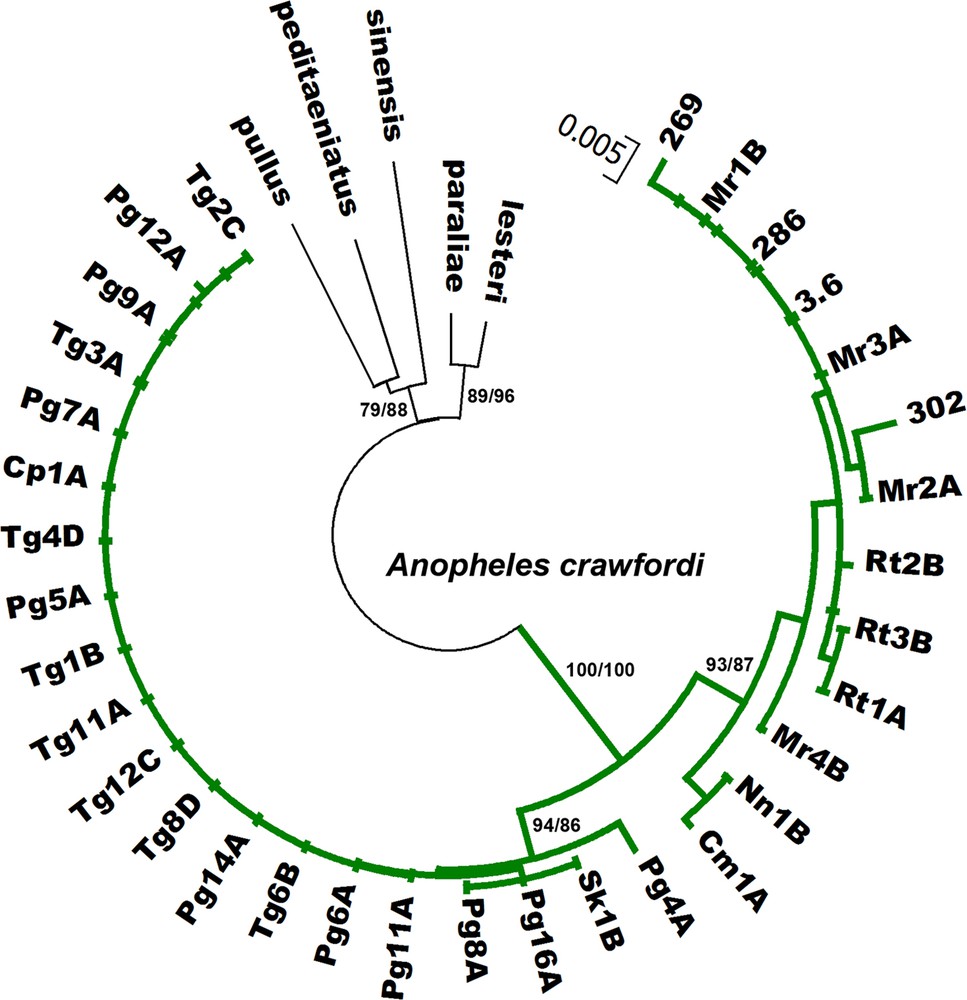
Phylogenetic relationships of A. crawfordi from Thailand, Cambodia, and Vietnam using Bayesian analysis based on COII sequences compared with five species of the Hyrcanus Group. Codes for the specimens are shown in Table 1. Numbers on branches are bootstrap values (%) of NJ analysis and Bayesian posterior probabilities (%). Only the values higher than 50% are shown. Bars represent 0.005 substitutions per site.
Mean intra-specific divergence of ITS2, COI and COII sequences of A. crawfordi Forms A, B, C and D from Thailand and Cambodia obtained using the Kimura two-parameter (K2P) model.
ITS2 | COI | COII | |
Within form | |||
A | 0.009 | 0.010 | 0.008 |
B | 0.014 | 0.018 | 0.012 |
C | 0.000 | 0.000 | 0.000 |
D | 0.000 | 0.000 | 0.000 |
Between forms | |||
A–B | 0.014 | 0.016 | 0.011 |
A–C | 0.005 | 0.006 | 0.005 |
A–D | 0.005 | 0.006 | 0.005 |
B–C | 0.014 | 0.015 | 0.011 |
B–D | 0.014 | 0.015 | 0.011 |
C–D | 0.000 | 0.000 | 0.000 |
4 Discussion
Metaphase karyotypes of A. crawfordi from two different locations (eastern region, Chanthaburi Province; southern region, Phang Nga Province) in Thailand were investigated by Baimai et al. [7]. The results revealed karyotypic variation via a gradual increase of extra heterochromatin on the X (X1, X2) and Y (Y1, Y2) chromosomes, which gave rise to two karyotypic forms [forms A (X1, X2, Y1) and B (X1, X2, Y2)]. These metaphase karyotypes could be distinguished based on size, shape, amount, and distribution of constitutive heterochromatin on the sex chromosomes. Likewise, the four distinct karyotypic forms [forms A (X1, X2, X3, Y1), B (X1, X2, X3, Y2), C (X2, Y3), and D (X2, Y4)] of A. crawfordi detected among the 29 isolines from six and two locations in Thailand and Cambodia, respectively, were due to the addition of extra heterochromatin on the sex chromosomes. Obviously, the above information indicated the possibility of a cytological mechanism for the karyotypic evolution of the Oriental Anopheles by gradually adding extra heterochromatin onto the arms of sex chromosomes, which is in keeping with hypothesis of Baimai [22]. Additionally, such chromosome distinction is very useful for the cytotaxonomic study of closely related species, especially sibling species and/or subspecies of Anopheles, as exemplified in other groups of Oriental anophelines [8,11,23–32].
Regarding the distribution of the four karyotypic forms of A. crawfordi, forms A and B appear to be common in all locations of both Thailand and Cambodia, whereas forms C and D are confined to Trang Province, southern Thailand. Remarkably, form A (10 isolines) was detected only in Phang Nga Province, whereas all karyotypic forms were obtained from eight isolines in Trang Province, despite these two provinces being separated by approximately 190 km. This is the first substantial evidence that supports the richness of ecological diversity in Trang Province, which seems to be the main key for supporting specific microhabitats that favor the karyotypic evolution of A. crawfordi.
Cross-mating experiments using isoline colonies of anopheline mosquitoes, which relate to results of cytology and molecular analysis to determine post-mating barriers, have proven to be an efficient technique for identifying sibling species and/or subspecies within Anopheles [8,11,23–32]. Regarding this matter, cross-matings among the four allopatric karyotypic forms of A. crawfordi were performed intensively. The absence of post-mating reproductive isolation through F2 generations strongly suggests that the four cytological races are conspecific. Low intra-specific sequence divergence (mean genetic distance = 0.000–0.018) of ITS2, COI, and COII of the four forms provides good supportive evidence. The maximum intra-species K2P values based on COI barcoding sequences obtained from this study were similar to that reported for Anopheles pallidus (0.0184) [33]. Kumar et al. [33] denoted that the K2P values were > 0.02 between different species for Culicidae. Our findings are in agreement with the results of cross-matings among karyotypic forms of other anophelines previously reported by several investigators, i.e., Anopheles vagus [34], A. pullus (= Anopheles yatsushiroensis) [35], A. sinensis [36–39], Anopheles aconitus [25], Anopheles barbirostris A1 and A2 [11,29], Anopheles campestris-like [30], Anopheles peditaeniatus [31,32], and Anopheles paraliae [40].
Until now, numerous studies have used ribosomal and mitochondrial DNA markers for phylogenetic analysis to determine the relationships among sibling species and/or subspecies of Anopheles [11,27,29,30,41–48]. Recently, Ngo et al. [49] reported that Anopheles dangi is deemed to be a synonym of A. crawfordi based on low mean genetic distance (0.006) of COI, COII and Cyt-b genes of mtDNA and the D3 gene of rDNA derived from specimens collected in south-central Vietnam. However, there have been no reports of evolutionary relationships among different karyotypic forms of A. crawfordi. Thus, our report is the first on the phylogenetic relationships among four karyotypic forms of Thai and Cambodian A. crawfordi populations. The comparison of our COI and COII sequences with those reported from Ngo et al. [49] were also performed in this study. Both Bayesian trees revealed that they are the same species. This study provides important information on the distribution of this species across different geographic regions, and highlights that the four karyotypic forms represent a single species. In addition, this is the first multidisciplinary approach based on cytological markers and DNA sequences to investigate different populations of A. crawfordi.
Disclosure of interest
The authors declare that they have no conflicts of interest concerning this article.
Acknowledgements
This work was supported by the Thailand Research Fund (TRF Senior Research Scholar: RTA5480006) and the Diamond Research Grant of Faculty of Medicine, Chiang Mai University to Wej Choochote and Atiporn Saeung. The authors would like to thank Dr. Wattana Navacharoen, Dean of the Faculty of Medicine, Chiang Mai University, for his interest in this research.