1 Introduction
The histone H1 class contains a set of proteins, i.e. non-allelic subtypes, commonly known as chromatin components critical for the formation of its higher-order structure and, therefore, engaged in the modulation of global and individual gene expression [2,3]. Due to a functional redundancy, the histone H1 activity is realized by a total set of subtypes or may be implemented individually [4], depending on the subtype-specific properties attributable mainly to its terminal domains [5]. Histone H1 globular domain (∼ 80 amino acids) binds the DNA to stabilize nucleosome structure, while N-terminal (25–30 amino acids) and C-terminal (∼ 100 amino acids) domains contribute to chromatin binding affinity and participate in chromatin folding, respectively [6]. Both histone H1 terminal domains are also the sites of post-translational modifications [7] and polymorphic variations [8].
Unlike the most organisms that usually have from four to six histone H1 subtypes, mammalian and avian histone H1 demonstrate the highest heterogeneity, manifesting itself by the presence of eleven (H1.1-H1.5, H1t, H10, H1LS1, H1T2, H1X and H1oo) and nine (H1.a, H1.a′, H1.b, H1.b′, H1.c, H1.c′, H1.d, H1.z and H5) subtypes, respectively [8]. Eight avian somatic histone H1 subtypes, i.e. H1.a, H1.a′, H1.b, H1.b′, H1.c, H1.c′, H1.d, H1.z, exhibit a similar expression during cell maturation, while the remaining subtype H5 undergoes gradual accumulation, achieving maximal expression level in the terminally differentiated erythrocyte [9]. Whereas among histone H1 subtypes outweighed are those which have mRNA 3′-hairpin loop structure and expressed in the S-phase only, expression of histone H5 encoded by a polyadenylated mRNA is independent of the cell cycle [10]. These features allow one to classify histone H5 as a replacement H1 subtype, which, contrary to most other vertebrate H1 histones, evolved from the gene ancestor that occurred in the invertebrates and plants [11].
Histone H5 is a small protein (∼20.5 kDa) composed of 189 amino acids comprising predominantly the basic residues of Arg and Lys. These amino acids are spaced unevenly in the distinct H5 domains [12], whose globular domain contributes to DNA binding, while the N-terminal and C-terminal domains carried binding and folding of the chromatin fiber, respectively [9]. In the terminally differentiated erythrocyte, histone H5 is abundantly deposited, in a ratio of 1.4 molecule per nucleosome, representing over a half of all histone H1 subtypes. The ability of histone H5 to create folded chromatin is the result of the effective neutralization of the DNA charge due to a high content of positively charged Arg residues. Besides, the histone H5 stable dimer formed via aromatic residues of His and Tyr maintains the adjacent nucleosomes in close contact. The histone H5-dependent cooperative DNA binding coupled with subsequent dimerization generates a stem-like structure required for efficient folding of chromatin. Thus, unlike the rest of H1 histones, histone H5 is a tissue-specific subtype responsible for the organization and stabilization of densely compacted, transcriptionally inactive, erythrocyte chromatin [9].
The previous findings confirmed the presence of chicken histone H5 polymorphic variants possessing disparate amino acid sequence regions belonging to the N-terminal domain [1]. In this work, the pheasant histone H5 phenotypic diversity is presented in order to compare the histone H5 heterogeneity between species. As revealed in the electrophoretic screening, the pheasant histone H5 is represented by two phenotypes only in a population. The comparably abundant and differently frequent histone H5 phenotypes, rare H5a and more common H5b, are variously situated both in an acetic acid–urea polyacrylamide gel (AU-PAGE) and in a sodium dodecyl sulfate polyacrylamide gel (SDS-PAGE). Based on the electrophoretic pattern of peptide maps obtained by N-bromosuccinimide (NBS) cleavage and α-chymotrypsin digestion, the pheasant histone H5 phenotypes contain different amino acid sequence region localized in the portion of its molecule that encompass the N-terminal part of the C-terminal domain and the entire N-terminal domain. This finding together with a similarly positioned amino acid sequences that differ between histone H5 allelic variants in chicken indicates a more universal character of histone H5 heterogeneity, which is not confined to a single species only.
2 Materials and methods
2.1 Animal material
The pheasant (Phasianus colchicus L.) individuals were housed at a farm of the Department of Poultry Breeding and Animal Material Evaluation, UTP University Science and Technology, Bydgoszcz, Poland.
2.2 Extraction of histone H5
Isolation of erythrocyte from whole blood and extraction of H1 histones from erythrocyte nuclei was performed according to the procedure of Neelin et al. [13]. Blood samples were taken from wing veins of individual birds into separate tubes supplemented with a solution containing 0.15 M NaCl and 0.015 M sodium citrate. The erythrocytes were obtained from blood sample by several consecutive suspensions in the same solution and subsequent centrifugation. Erythrocyte nuclei were isolated with 3% saponin in a 0.1 M sodium phosphate buffer (pH 7.0). To obtain H1 histones, erythrocyte nuclei were repeatedly washed with a 0.1 M sodium phosphate buffer (pH 7.0) and doubly extracted with a perchloric acid solution (1 M and 0.5 M). The proteins, precipitated with 20% trichloroacetic acid, were washed with pure acetone, acidified with HCl, and dried.
2.3 Electrophoretic analysis
Histone H1 preparations, prepared by dissolving 1 mg of protein in 200 μL of a sample buffer (8 M urea, 0.9 M acetic acid and 10% 2-ME), were separated in the AU-PAGE and SDS-PAGE as described by Kowalski and Pałyga [14]. AU-PAGE was conducted in the 15% polyacrylamide gel containing 8 M urea and 0.9 M acetic acid. SDS-PAGE proceeded in 13.5% polyacrylamide gel in the presence of 0.1% SDS. The proteins were visualized after a routine staining with the solutions of Coomassie Blue R-250 dye. For a better visualization of the faintly stained histone H5 N-peptides, especially those that were derived from the NBS, the gel fragments were extra stained with the silver nitrate solution.
2.4 Processing of gel images and quantification of histone H5 phenotypes
The electrophoretic patterns of histone H5 phenotypes recorded with the Doc-Print II gel imaging system (Vilber Lourmat) were processed by using ImageJ 1.44c software (www.rsbweb.nih.gov/ij). An area (square millimeters) and a raw integrated density (a sum of the values of the pixels in the selected area) were determined to estimate the intensities of histone H5 phenotypes protein bands. The measurements were conducted in ten replicates (n = 10) for individual histone H5 preparations belonging to phenotypes H5a and H5b.
2.5 Statistical analysis
The difference between the levels of histone H5 phenotypes was evaluated with the use of Student's t-test, assuming a statistical significance at P-value < 0.05. The coefficient of variation, indicating a relative variability, was calculated as a ratio of standard deviation and the mean. Values of the coefficient of variation below 0.25 were regarded as corresponding to low variability.
2.6 Peptide mapping
To prepare histone H5 peptide maps, on average four protein bands corresponding to histone H5 individual phenotype resolved by AU-PAGE were concentrated in the SDS-PAGE gel after two successive incubation steps (2 × 15 min) in an adaptation buffer containing 2.1% SDS, 2% 2-ME, 10% glycerol and 0.124 M Tris–HCl, pH 6.8. Both NBS cleavage and α-chymotrypsin digestion were made as described by Kowalski et al. [15], with the use of 0.1% NBS dissolved in 50% acetic acid and 0.01% α-chymotrypsin buffered with the solution of 0.1% SDS, 10% glycerol, 0.125 M Tris–HCl, pH 6.8 and 1 mM EDTA. The products of chemical cleavage and enzymatic digestion of histone H5 phenotypes were detected by SDS-PAGE.
3 Results and discussion
3.1 Identification of erythrocyte histone H5 phenotypic variation in the pheasant population
In order to ascertain whether histone H5 is heterogeneous in the pheasant population, the histone H1 preparations obtained by extraction of erythrocyte nuclei with perchloric acid solutions were subjected to screening by AU-PAGE and SDS-PAGE. In the SDS-PAGE study of total histone H1 subtypes, histone H5 was detected as a rapidly roaming single-protein band (Fig. 1A) possessing different rates of electrophoretic migration (Fig. 1A and B, left module). From the 46 pheasant individuals analyzed, a faster moving H5 band representing the rare phenotype H5a (frequency 0.26) was found in the 12 birds only. The remaining 34 birds owned a slowly migrating form of histone H5 corresponding to the abundant phenotype H5b (frequency 0.74). A variable in-gel location of histone H5 phenotypes was also detected after resolving histone H1 preparations in the AU-PAGE. In these electrophoretic gels, a histone H5 protein band corresponding to phenotype H5a possessed a retarded migration, as compared to a more mobile band of histone H5 belonging to phenotype H5b (Fig. 1B, right module). Based on the obtained electrophoretic patterns, the protein bands constituting histone H5 phenotypes seem to be different as regards both net charge and molecular weight. A more positively charged phenotype H5b seems to have greater molecular weight, contrary to phenotype H5a, which possesses a less negative net charge and a lower molecular weight (Table 1).
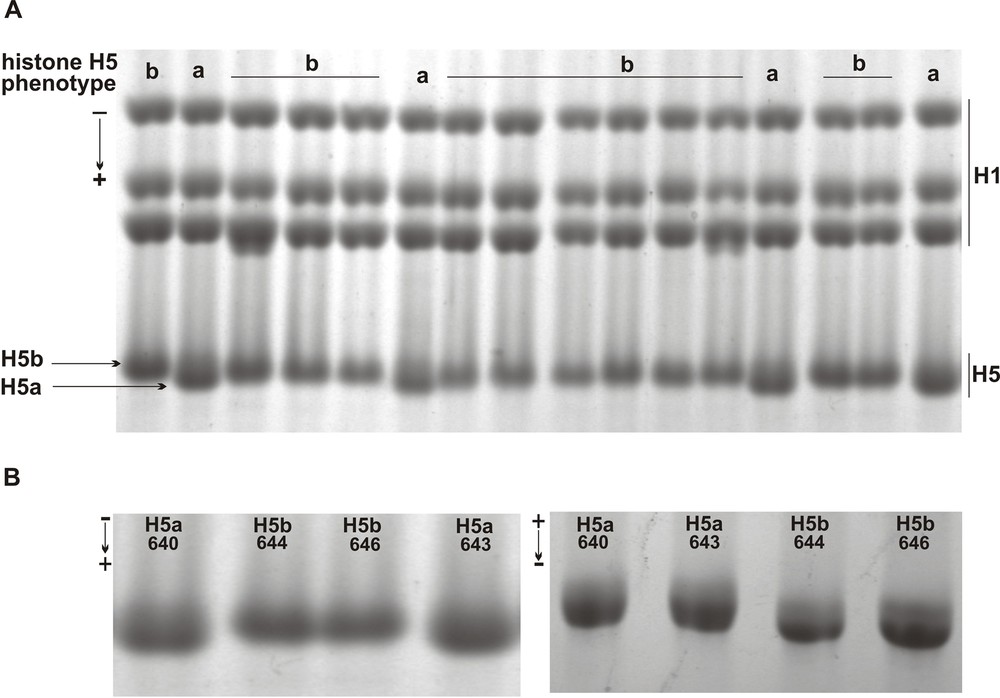
Electrophoretic identification of the pheasant erythrocyte histone H5 phenotypes. A. Slower migrating histone H1 subtypes (H1) and faster moving histone H5 phenotype H5a and H5B resolved in the SDS-PAGE. B. Electrophoretic mobility of histone H5 phenotype H5a, individuals 640 and 643, and H5b, individuals 644 and 646, in the SDS-PAGE (left) and in the AU-PAGE (right).
Electrophoretic and population characteristics of pheasant histone H5 phenotypes.
Histone H5 phenotype | H5a | H5ab | H5b |
Electrophoretic analysis | |||
Mobility in SDS-PAGE/AU-PAGE | Fast/slow | Slow/fast | |
Net charge/molecular weight | More negative/lower | More positive/higher | |
Population analysis | |||
Number of individuals obs./exp.a | 12/3.1 | 0/17.7 | 34/25.2 |
Frequency of phenotype obs./exp.b | 0.26/0.068 | 0/0.384 | 0.74/0.548 |
a Difference in the phenotypes distribution χ2 = 43.32, P < 0.0001.
b Fixation index F = 1 (Hobs 0, Hexp 0.386)
Quantification obtained by measuring an area and the integrated density of histone H5 phenotypes protein bands revealed its comparable intensities in both AU-PAGE and SDS-PAGE patterns (Table 2). Whereas the coefficient of variation computed for phenotypes H5a and H5b was below 0.25, the relative variability of the corresponding protein bands is low (Table 2). Likewise, no significant difference (P > 0.05) between the levels of histone H5 phenotypes was detected with the usage of Student's t-test after comparison of the measurements of the protein band area (P = 0.475 for H5 bands resolved in the AU-PAGE and P = 0.067 for H5 bands separated in the SDS-PAGE) and of the integrated density (P = 0.078 for H5 bands resolved in the AU-PAGE and P = 0.084 for H5 bands separated by SDS-PAGE).
Quantity (mean ± standard deviation) and variability (coefficient of variation) of histone H5 phenotypes. The calculation was conducted using measurements from an area and the integrated density of protein bands belonging to ten (n = 10) histone H5 preparations of a given phenotype.
Histone H5 phenotype | H5a | H5b |
AU-PAGE | ||
Area/coefficient of variation | 5187.3 ± 550.17/0.106 | 5201.3 ± 454.71/0.087 |
Integrated density/coefficient of variation | 468633.1 ± 63599.3/0.135 | 437194.1 ± 22480.3/0.051 |
SDS-PAGE | ||
Area/coefficient of variation | 2035.4 ± 185.3/0.091 | 1924.8 ± 136.96/0.071 |
Integrated density/coefficient of variation | 218162.4 ± 25111.6/0.115 | 200604.0 ± 29522.0/0.147 |
The cases in which avian histone H1 allelic variants differ in the net charges, i.e. duck and chicken histone H1.a [16] and pheasant histone H1.c [17], are scarce. Usually, the polymorphism of histone H1 subtypes is detected in relation to a molecular mass difference between allelic variants. It refers to several H1 subtypes, as guinea fowl H1.b and H1.c [15], duck H1.b and H1.z [18], as well as quail H1.b and H1.z [19], including also the chicken histone H5 [1]. Although the chicken histone H5 phenotypes were identified based on a distinct mobility in the SDS-PAGE, they were also detected as being differently located in the patterns of AU-PAGE. The altered mobility of chicken H5 phenotypes in the AU-PAGE study occurs probably due to modification(s) that influences their electrophoretic migration [1]. The histone H5 phenotypic variation presented in this work seems to resemble that which was identified previously in the chicken [1]. A different location of the pheasant histone H5a and H5b in the SDS-PAGE reflects its molecular mass difference, while the shift in the AU-PAGE may be triggered by a modification reducing the positive charge, i.e. acetylation, and/or adding an extra negative net charge, i.e. phosphorylation. Moreover, an anomalous electrophoretic move may be also linked to an oxidation process increasing the density of positive charge [20]. Whereas acetylated, oxidized and phosphorylated residues of Lys/Thr, Met, and Thr/Ser, respectively, were detected in chicken histone H5 [21], it is likely that these modifications may also occur in pheasant histone H5, causing an aberrant electrophoretic migration of its phenotypes. Therefore, acetylation may correspond to a delay in the migration of phenotype H5a, while oxidation and/or phosphorylation may result in an increased mobility of phenotype H5b.
Except for one chicken line in which no phenotype H5a was detected, the histone H5 genetic variants form three differently frequent phenotypes in the remaining six avian breeds analyzed [1], similarly to those bird populations in which both homo- and heterozygous histone H1 phenotypes are found according to a varying frequency [15,16]. However, the certain histone H1 phenotypes are very rare and may be absent in a population [8]. A substantial disproportion in the frequency of histone H1.z phenotypes was noted in the duck population, in which phenotype H1.z2 was detected in two of the five screened lines only at a frequency below 0.005 [22]. Whereas histone H1.a1 was common in the duck and chicken groups tested, histone H1.a2, which was present in the duck breeding groups, was missing in the conservative ones, and occurred in the progeny of purposely mated heterozygous parents only [16]. Moreover, none of the phenotypes H1.b2 and H1.z2 was found in all screened duck lines representing both breeding and conservative groups [18]. A lack of histone H1 phenotype may be also due to the inbreeding or drift operated on small-sized populations, as in the case of white-feathered guinea fowl strain in which a heterozygous phenotype H1.b1b2 and H1.c1c2 was not detected in general [15]. Likewise, an extreme departure from Hardy–Weinberg equilibrium (P < 0.0001) and a positive fixation index (F = 1) (Table 1) indicates that inbreeding may act in a pheasant population. A probable cause of homozygosity in the pheasant population might have been a relocation of wild-living individuals to the husbandry, which led to the removal of heterozygous individuals from a small-sized population. Whereas inbreeding results in the reduction of heterozygosity with a concomitant increase of the homozygotes proportion relative to the random expectations [23], the loss of heterozygosity observed in the breeding conditions corresponds to an increased frequency of the homozygous phenotypes relative to the wild population [24].
3.2 Identification of an altered molecule sequence region between the pheasant erythrocyte histone H5 phenotypes
To establish a region of histone H5 molecule that comprises an altered sequence responsible for a phenotypic variation, the peptide maps of histone H5 phenotypes were prepared by enzymatic digestion with α-chymotrypsin and chemical cleavage with NBS. Initially, the protein bands of particular histone H5 phenotype resolved in the AU-PAGE were concentrated separately in the SDS-PAGE to obtain a sufficient amount of protein for subsequent chemical and enzymatic reaction. The reaction with NBS solution was conducted by in-tube incubation of the gel stripes containing appropriate histone H5 phenotype with subsequent detection of the products in the SDS-PAGE. α-Chymotrypsin digestion was performed in a stacking SDS-PAGE gel, on which the gel pieces with the corresponding H5 phenotype protein band were applied and loaded with the enzyme solution.
Both NBS and α-chymotrypsin peptide maps contain a pattern of histone H5 phenotype-derived peptides in which the slowly moving peptide bands possess a similar in-gel location, while a higher and lower migration rate is characteristic of peptide bands migrating faster (Fig. 2A). In regard to the specificity of the reaction conducted by α-chymotrypsin, which digests the peptide bond at a single Phe93 residue in the chicken and duck histone H5 (Fig. 3), slow-migrating peptides correspond to the histone H5 sequence fragment stretching from the Arg94 residue to the C-terminal Lys residue. A peptide that moves faster belongs to a sequence region covering the first 93 amino acids, i.e. is from the N-terminal amino acid residue to the 93 residue of Phe (Fig. 2A and Fig. 3). NBS, which cleaves the peptide bond at the Tyr residue located in the position 28, 53 and 58 of chicken and duck histone H5 (Fig. 3), gives two slow-migrating peptides (Fig. 2A). A lower rate of the electrophoretic mobility has peptide extends from the Ser29 residue to the C-terminal Lys residue and a higher migration rate correspond to the peptide that covers amino acids located between the Ile54 residue and/or Lys59 and the C-terminal residue of Lys. A single peptide, which moves faster than the C-peptides, produced by NBS, encompasses a sequence region from the N-terminal amino acid residue to the Tyr residue at the position 28 (Fig. 2A and Fig. 3). Based on a difference in the electrophoretic migration between N-terminal peptides of histone H5a and H5b (Fig. 2A and 2B), referred to a highly conserved chicken and duck histone H5 sequence (Fig. 3), it seems that the pheasant histone H5 phenotypes might have other amino acid sequence regions located in a whole N-terminal domain (residues 1–25) and in the adjacent portion of the globular domain (residues 26–93).
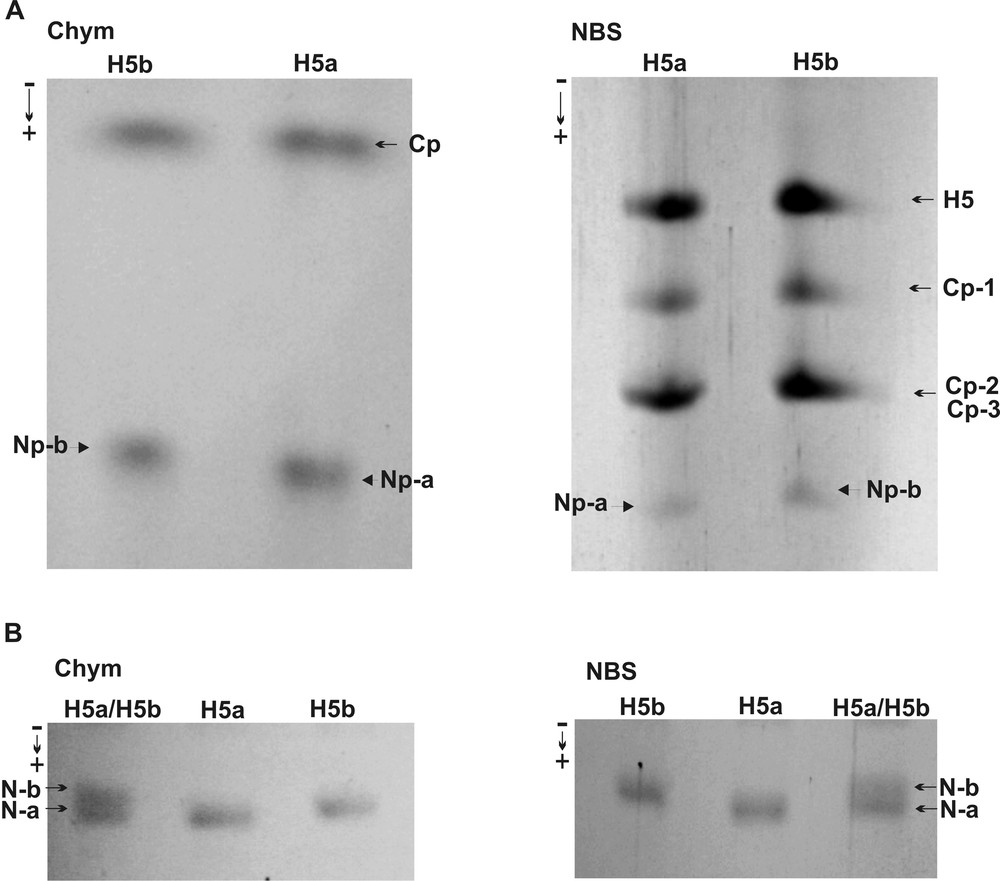
Polypeptide maps of histone H5 phenotypes. A. α-Chymotrypsin (Chym) digestion (left) and NBS cleavage (right) of histone H5 phenotypes. N-p and C-p indicate the N-terminal and C-terminal peptides, respectively. B. Migration rate of N-terminal (N-a and N-b) peptides digested by α-chymotrypsin (left) and cleaved with NBS (right), electrophoresed separately, H5a and H5b, and co-electrophoresed, H5a/H5b, in the SDS-PAGE.
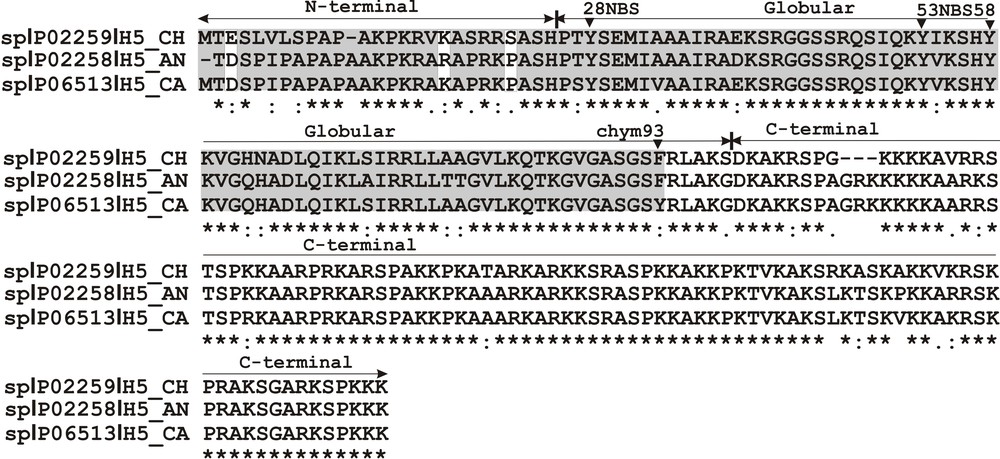
Sequence alignment of chicken (H5_CH P02259) and duck, Anser anser (H5_AN P02258) and Cairina moschata (H5_CA P06513), histone H5. A conservation degree observed between sequences (∼ 80%) is designated as: (*) identical residues, (:) highly conserved residues, (.) weakly conserved residues. The arrows indicate histone H5 domains (N-terminal, globular and C-terminal) and the arrowheads depict the sites of α-chymotrypsin digestion (Phe93) and the NBS cleavage (Tyr28, Tyr53 and Tyr58). The shaded sequence area depicts a region containing the expected amino acid sequence difference between histone H5 phenotypes. The white bars show an amino acid substitution, i.e. E/D, K/R, and S/P.
While altered protein sequence fragments are usually localized in the C-terminal domain of avian histone H1 polymorphic variants [3,8], only quail histone H1.b [25] and chicken histone H5 [1] possess different amino acids situated in the N-terminal domain. So, pheasant histone H5 is a rare histone H1 subtype whose heterogeneity is related to the phenotypes that have altered sequence stretches positioned in the N-terminal portion of a protein. Whereas the globular domain is the most conserved region of histone H1 [26], it can be expected that the sequence variation of pheasant histone H5 phenotypes should be attributable to a more variable N-terminal domain. As opposed to the C-terminal domain known as responsible for formation and stabilization of a higher-order chromatin structure and as being engaged in a majority of histone H1 interactions [27], the N-terminal domain is important in both subtype specific [28] and overall [29] binding of histone H1 to chromatin. Besides, the binding properties of the N-terminal domain are influenced by some structural transition related to intrinsic disorder propensity [30,31] and also by post-translational modifications, mainly acetylation and phosphorylation [26]. It seems that histone H1 N-terminal domain functioning may also be diversified due to the occurrence of phenotypic variants that differ in their amino acid sequence. Amino acid substitutions, i.e. K/R, S/P and E/D, occurring in the histone H5 N-terminal domain (Fig. 3) may set a more positive and/or more negative charge of histone H5 phenotype, leading to its altered interaction with DNA and chromatin. Moreover, a subtle change in the amino acid composition related to the polymorphism of a single amino acid residue, strongly influences the histone H1 binding affinity that leads to form its varied structural states chromatin [32].
In summary, the results obtained in this work indicate that histone H5 heterogeneity does not concern only a single species, but might be related to a phenotype-specific influence on the organization and/or function of avian chromatin. The chromatin effects might be determined individually by a peculiar histone H5 phenotype sequence region localized in the N-terminal portion of its molecule.
Disclosure of interest
The author declares that he has no competing interest.