1 Introduction
Stevia rebaudiana is a traditional therapeutic plant species, used for the healing of different illnesses; it belongs to family Asteraceae. It is propagated in countries of tropical and sub-tropical regions [1]. The medicinal importance of Stevia can be judged from the example of the Guarani tribes of Brazil and Paraguay, who used the leaves of this species in medicinal teas for the treatment of gastric problems and other related ailments [2]. During plant development, different compounds are synthesized in the leaves of Stevia, of which stevioside is produced in higher quantities [2–4]. Stevioside is one of the major active compounds of steviol glycosides, which are 300 times sweeter than cane and beetroot sugar and are widely applicable for diabetic patients. Stevioside was considered zero caloric as compared to commercial sugar. The literature is scarce regarding the receptors for stevioside absorption to human blood. Further, it has been reported that stevioside ingestion does not produce side effects like genotoxicity and mutagenicity [4]. Now, it is regularly cultivated in many countries as a food supplement [4,5].
Stevia rebaudiana is traditionally cultivated through many procedures, like stem cuttings and seed germination. But stem cutting gives a lesser number of plants because it needs higher primary stocks, which is laborious, and seed germination also provides a lesser number of plantlets because the seed size is very small, and it loses its capability of germination soon after collection [2,6,7]. To avoid these problems and to get desirable biomass and bioactive compounds in short periods of time, different in vitro techniques, like plant cell, tissue and organ cultures are widely applied [8–15]. Among different in vitro cultures, adventitious root culture provides a lot of chances for the accumulation of drugs and bioactive substances beneficial to human health without field cultivation [16–18]. Various biotic and abiotic elicitors are introduced into different culture media to enhance the accumulation of secondary metabolites by influencing their biosynthetic pathways.
Light quantity and quality are among the most important elicitors influencing the whole development of the plant, its morphology, and the production of medicinally important bioactive compounds [19]. It is reported that light directly triggers plant morphology, physiology, biochemistry, and its various developmental processes. Light also play a key role in primary and secondary metabolism and production of various bioactive compounds [20,21]. Light quality and intensity regulate synergistically or antagonistically the secretion mechanism of the secondary metabolites [22]. Using different lights could lead to higher production and accumulation of secondary metabolites, which are providing a way towards the industrial application of such medicinal plants.
This is the first successful report on the development of adventitious root culture in Stevia under the influence of various spectral lights for the accumulation of biomass and antioxidant secondary metabolites.
2 Materials and methods
2.1 Seeds preparation for germination
Seeds were collected from Stevia plants grown in Ground and Garden Nursery, The University of Agriculture, Peshawar. Black coated seeds with matured embryos were surface sterilized by using the protocol of Ahmad et al. [23]. The seeds were treated with ethanol (70%) and mercuric chloride (HgCl2; 0.2%) for 1 min, and then washed many times with autoclaved distilled water. The Murashige and Skoog (MS; 1962) [24] medium was prepared without plant growth regulators for seed germination. The medium was prepared using 30 g/l sucrose; for solidification 7–8 g/l agar was used; the pH was kept at 5.8; finally, the medium was autoclaved at 121 °C for 20 min. Then the sterilized seeds were inoculated into the MS media and were placed under 16/8 h photoperiod with an illumination intensity of 40 μmol m−2·s−1; finally, the cultures were maintained in growth chambers at room temperature for 30 days. Within 30 days, the seeds were germinated into plantlets and the explant was collected for the required culture development.
2.2 Explant collection for callus development
From in vitro grown plantlets, leaves were collected as explants for callus development. For culture development, the MS medium was prepared using a mixture of 6-benzyladenine (BA; 2.0 mg/l) and 2, 4-dichlorophenoxy acetic acid (2, 4-D; 2.0 mg/l). The inoculums (leaf explants, ∼4–5 mm2 pieces) of in vitro grown plantlets were introduced into the MS medium for one month. Friable calli appeared in the inoculated flasks after a few days of inoculation. These calli cultures were used for the development of adventitious roots culture.
2.3 Development of adventitious root culture
Accurately, 250 mg of 30-day-old calli were transferred into 100 ml Erlenmeyer flasks for the development of adventitious roots. The MS-basal media was supplemented with naphthalene acetic acid (0.5 mg/l), 7–8 g/l agar and 30 g/l sucrose, and the pH was adjusted to 5.8. Fine roots appeared after 15 days of calli inoculation. The tips (0.5 cm) of these roots were transferred into liquid media containing similar concentrations of NAA. These cultured flasks were placed in an orbital shaker (120 rpm; Gallenkamp, England; 25 °C) for 15 days in dark to get inoculum stock.
2.4 Spectral lights treatment
An inoculum of adventitious roots obtained from callus cultures was divided into six equal parts by weight. Six flasks were taken and covered with various colored glass sheets for absorbing specific illuminations. The wavelength of the blue light was 380–560 nm, green 480–670 nm, violet 350–400 nm, red 610–715 nm, yellow 530–780 nm; white light (uncovered flask) was used as control. Each flask was supplemented with a known amount of inoculum.
2.5 Establishment of growth curve
After placing inoculated flasks in an orbital shaker (120 rpm and at 25 °C) for a period of 30 days under exposure to different illumination sources, the growth curve for biomass accumulation was investigated. For growth kinetics, data collection was made at 3-day intervals for a period of 30 days. In response to different lights spectrums, the growth curve was established, including lag phases, log phases, and decline phases for the biomass accumulation of the fast-growing adventitious root cultures.
2.6 Determination of biomass
To determine adventitious root fresh weight (FW), the roots were carefully removed from the inoculated flasks one by one. Sterilized distilled water was used for washing the de-flasked roots. The roots were gently pressed using filter paper (Whatman ltd., England) for the elimination of media particles and excess water and were weighed (Sartorius digital balance; Germany). The same procedure was followed for dry weight (DW) determination. For drying, the fresh weighed roots were further placed in an electrical oven (thermo scientific; Germany, using 50 °C) for drying, and subsequently weighed for dry biomass investigation.
2.7 Analytical methods
By using mortar and pestle, the dried adventitious roots were grinded for extract preparation. Exactly 10 mg of the powdered from each sample was added with 10 ml of ethanol in falcon tubes. During about 7 days, the solution was regularly vortexed and followed by centrifugation at 10,000 rpm for 10 min to get the supernatant for the determination of the phenolics, of the flavonoids, and of the antioxidant activity.
2.8 Total phenolic content and production
To determine the total phenolic content (TPC) in each sample, the procedure of Ahmad et al. [23] was utilized. Accurately, a 0.03 ml extract was added with 0.1 ml (2 N) of Folin–Ciocalteu reagent and diluted with 2.55 ml of sterilized distilled water. The mixture was centrifuged at 10,000 rpm for 10 min, which was followed by incubation for a few minutes in the dark to avoid oxidation. A 45 μm membrane was used in a UV–visible spectrophotometer (Shimadzu-1650; Japan) cuvette for filtration of the mixture. At a wavelength of 760 nm, the absorbance of the resulting mixture was tested. For plotting a standard calibration curve, gallic acid (Sigma; 1.0–10 mg/ml; R2 = 0.9878) was used. By using the following equation, the gallic acid equivalent (GAE), in mg/g of dry root biomass (DRB), was inferred from % TPC:
The total phenolic production (TPP) was calculated from the total phenolic content. For production, we multiplied the dry biomass by the total phenolic content.
2.9 Total flavonoid content and production
To determine the total flavonoid content (TFC) in each sample, the procedure of Ahmad et al. [23] was used. 0.25 ml of ethanol extract of each sample were added to 1.25 ml of sterilized distilled water and 0.075 ml of 5% (w/v) AlCl3. Then the solution was added to 0.5 ml of NaOH (1 M), which was followed by incubation for 10 min; finally, the mixture was centrifuged at 10,000 rpm for 10 min. Through UV–visible spectrophotometry (Shimadzu-1650PC, Japan) at 510 nm, the absorbance was checked. For plotting the standard calibration curve, Rutin (Sigma; 1.0–10 mg/ml; R2 = 0.9866) was used. TFC results were expressed in (RE) mg/g-DRB of extracts.
2.10 Antioxidant activity determination
For the determination of DPPH-free radical scavenging activity (DRSA) the protocol of Ahmad et al. [23] was followed. Here, 1.0 ml of ethanol extract (5 mg per 20 ml) of each sample was added to 2.0 ml of DPPH-free radical solution (0.25 mg per 20 ml × 4). Then the medium was incubated in the dark up to 30 min to form stable complexes. Through UV–visible spectrophotometry (Shimadzu-1650PC, Japan), the absorbance of the resulting mixture was checked at a wavelength of 517 nm at room temperature. Finally, by using the given equation, the DRSA was calculated as the percentage of DPPH discoloration:
The total flavonoid production (TFP) was calculated from the total flavonoid content. For production, we multiplied the dry biomass by the total flavonoid content.
2.11 Statistical analysis
The design of the experiments was totally randomized, and each step was repeated two times. Data regarding different parameters were recorded in triplicates. The Statistix software (v. 8.1; USA) was used for ANOVA and least significant differences. For the determination of mean values and standard errors, the same software was used. For graphical presentation, the Origin software (v. 9.0) was used.
3 Results
3.1 Spectral light-induced adventitious roots biomass accumulation
The data regarding the biomass accumulation were taken for a period of 30 days, with 3-day intervals. The cultures were established under different illuminations, including violet, red, blue, yellow, and green lights. Beside this, one culture was established under white light (Fig. 1). In this study, a known amount of inoculum was shifted into flasks containing MS media supplemented with 0.5 mg·l−1 of NAA. These cultured flasks were covered with various colored sheets and placed in a shaker under continuous illumination. During growth kinetics, the lag phases were observed to be shorter in all established cultures, ranging from day 1 to day 6. This was followed by log phases, which were found to be longer than lag phases, ranging from day 9 to day 27. These were the actual phases where the major contribution to biomass accumulation was observed. They were followed by a very short stationary phase, ranging from day 27 to day 30; a decrease in biomass was observed during these phases in all established cultures. In terms of highest biomass accumulation, the violet light produced 2.495 g/flask of fresh biomass on day 27 in the log phase (Fig. 2). Violet light was followed by green light (1.94 g/flask), yellow (1.895 g/flask), blue (1.702 g/flask), control (1.625 g/flask), and red (1.025 g/flask) light as shown in Fig. 2a–e. The current results lead us to conclude that violet light is more effective for biomass accumulation than other colored lights as well as control.
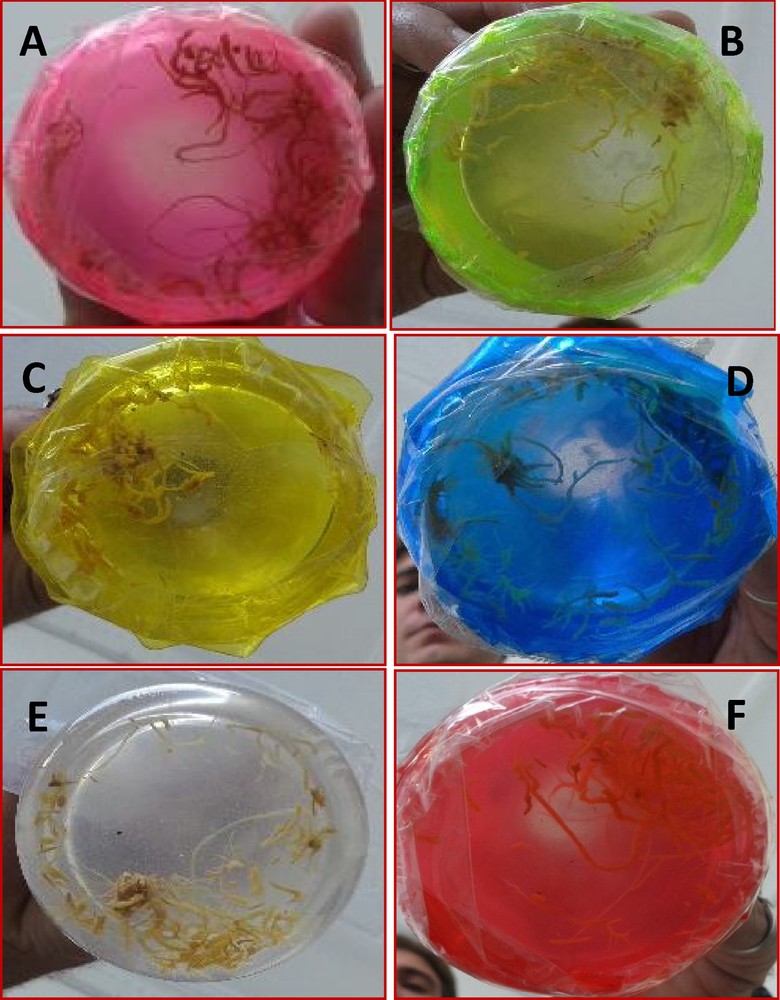
Effect of different illuminations on biomass accumulation (A) Violet light, (B) green light, (C) yellow light, (D) blue light, (E) white, (control) light, and (F) red light.
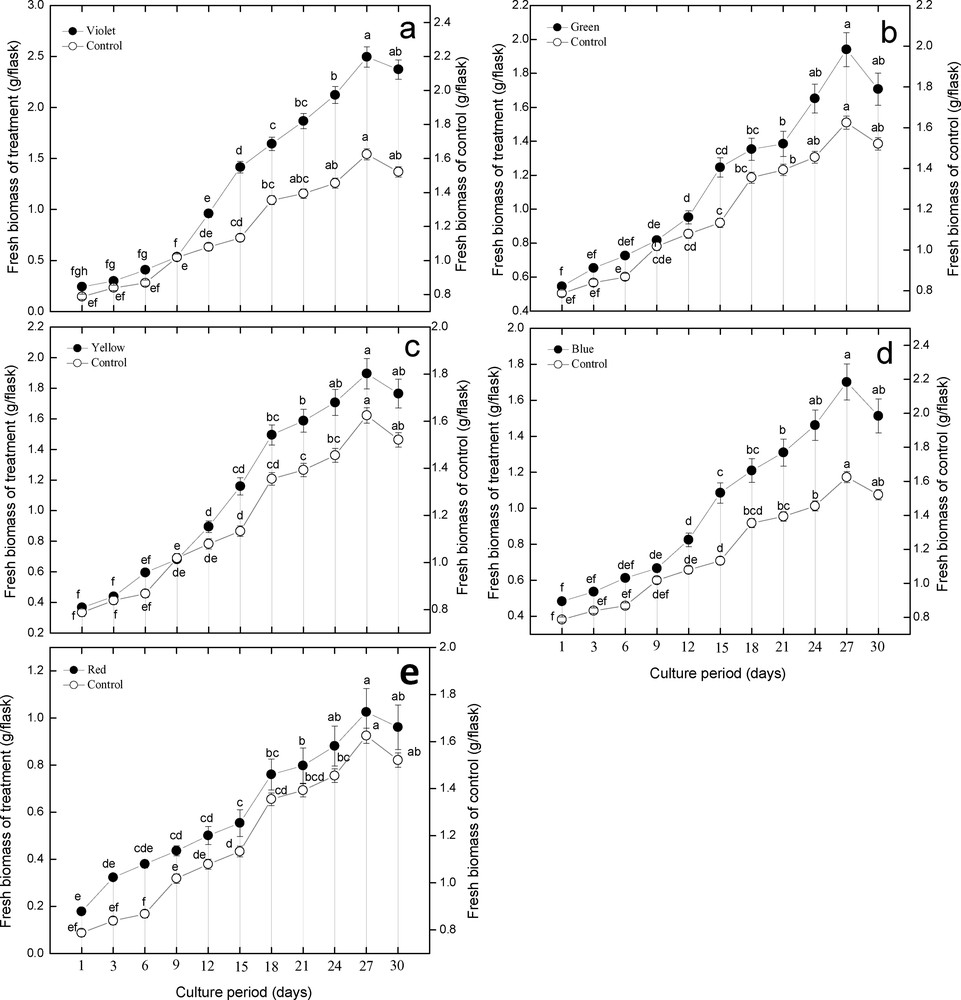
Effect of different illuminations on fresh biomass accumulation during growth kinetics of adventitious root cultures in Stevia rebaudiana in comparison with control (a) violet light, (b) green, (c) yellow, (d) blue, (e) red light. The growth kinetics of adventitious roots was studied for a period of 30 days. Mean data with common alphabets and standard errors are significantly different at P < 0.05.
3.2 Colored light-triggered phenolics content and production, and its correlation with dry biomass
This study was carried out to determine the effect of different spectral lights on total phenolic and total flavonoid accumulation in adventitious root cultures of S. rebaudiana. Among the different spectral lights, blue light induced the highest accumulation of TPC (6.56 mg GAE/g DW), followed by red light (6.46 mg GAE/g DW), yellow (5.98 mg GAE/g DW), green (5.70 mg GAE/g DW), control (5.44 mg GAE/g DW), and violet (4.55 mg GAE/g DW), as shown in Fig. 3. Looking at the correlation between TPC, TPP, and dry biomass, it can be observed that there is a strong correlation between TPC, TPP, and dry biomass up to some extent (Figs. 3 and 4). The maximum TPC (6.56 mg GAE/g DW), maximum production of phenolics and dry biomass (0.19 g/flask) was observed in roots treated with blue light. The second highest dry biomass of 0.15 g/flask and TPC (6.46 mg GAE/g DW) was observed in cultures grown under red light. Further, the dry biomass and TPC were also in correlation, but showed little deviation. The current results suggest that blue light is most suitable for production of TPC, TPP and dry biomass in adventitious root cultures of S. rebaudiana.
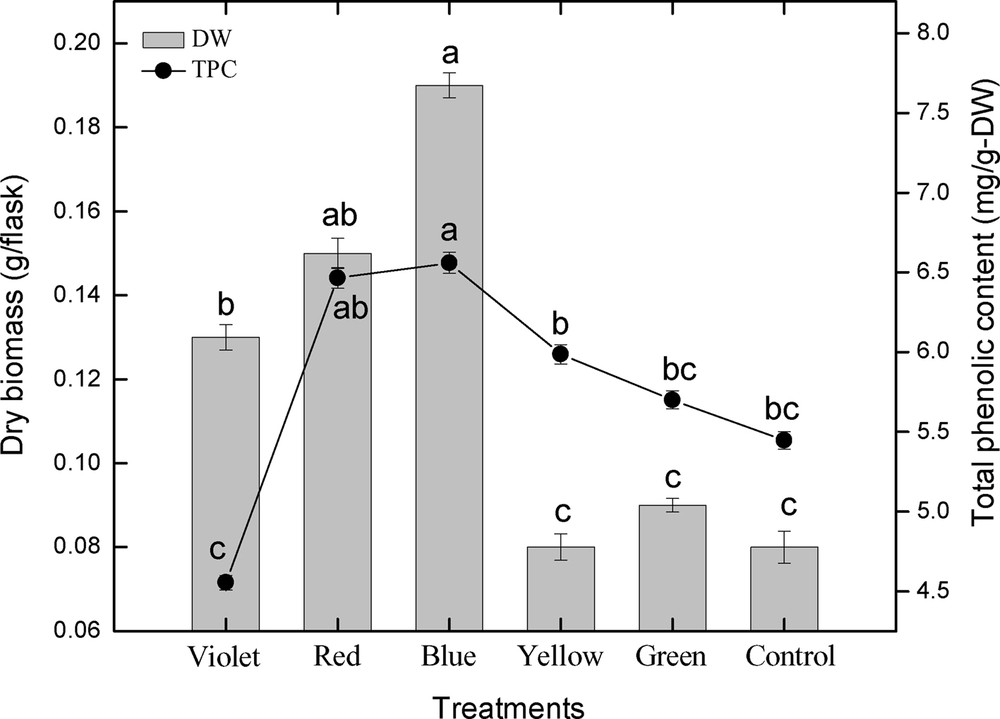
Correlation of total phenolic content with dry biomass in adventitious root cultures of S. rebaudiana. Mean data with common alphabets and standard errors are significantly different at P < 0.05.
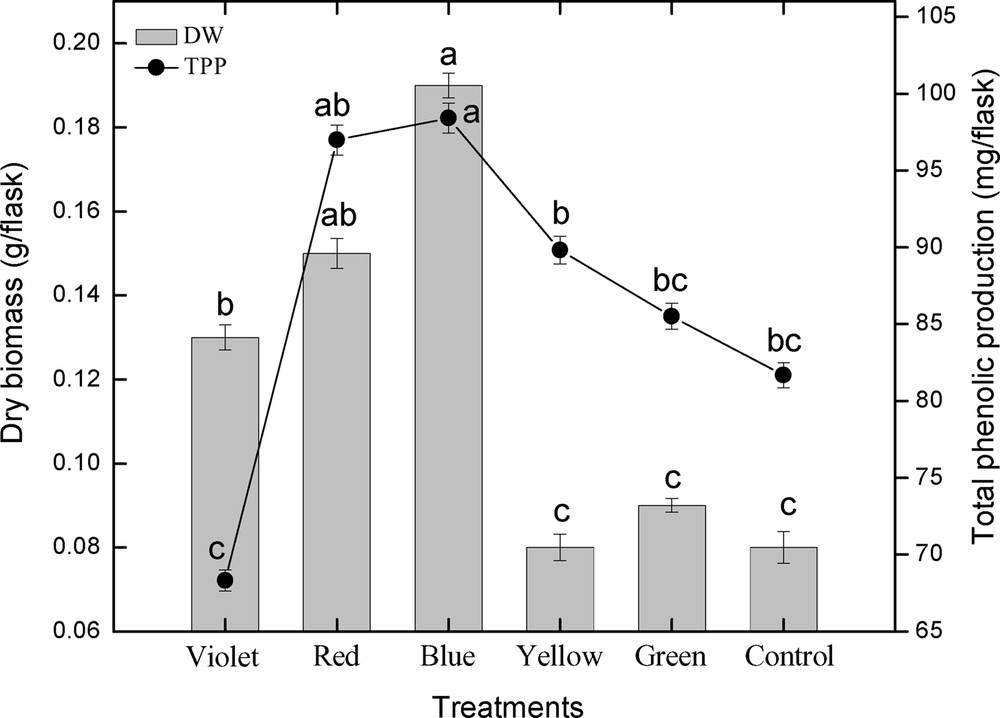
Correlation of total phenolic production with dry biomass in adventitious root cultures of S. rebaudiana. Mean data with common alphabets and standard errors are significantly different at P < 0.05.
3.3 Colored light-triggered flavonoid content and production, and its correlation with dry biomass
The effect of different spectral lights on total flavonoid accumulation in adventitious root cultures of S. rebaudiana was investigated. Among different spectral lights, the blue light induced the highest accumulation (4.33 mg RE/g DW) of TFC, followed by red light (4.20 mg RE/g DW), violet (4.14 mg RE/g DW), control (4.04 mg RE/g DW), green (4.01 mg RE/g DW), and yellow (3.63 mg RE/g DW), as shown in Fig. 5. A similar pattern of biomass accumulation was observed in TFP using similar lights (Fig. 6). These results showed that blue light caused the highest accumulation of TFC and TFP, while yellow light produced the least TFC and TFP, which concludes that yellow is the inhibitory light among the other used colored lights as well as control light (Figs. 5–6). The correlation between TFC, TFP and dry biomass was also determined. From the current results, it can be observed that there is a good correlation between TFC, TFP, and dry biomass of adventitious root cultures after the application of different spectral lights (Fig. 5–6). The maximum TFC (4.33 mg RE/g DW) and the maximum production of dry biomass (0.19 g/flask) was observed in roots treated with blue light. The second highest dry biomass of 0.15 g/flask and TFC (4.20 mg RE/g DW) were observed in cultures grown under red light. Similarly, the third highest dry biomass of 0.13 g/flask and TFC (4.14 mg RE/g DW) were observed in cultures grown under violet light. The current results suggest that blue light is most suitable for TFC, TFP and dry biomass production in adventitious root cultures of S. rebaudiana, The TFC and TFP increases as biomass increases, and vice versa.
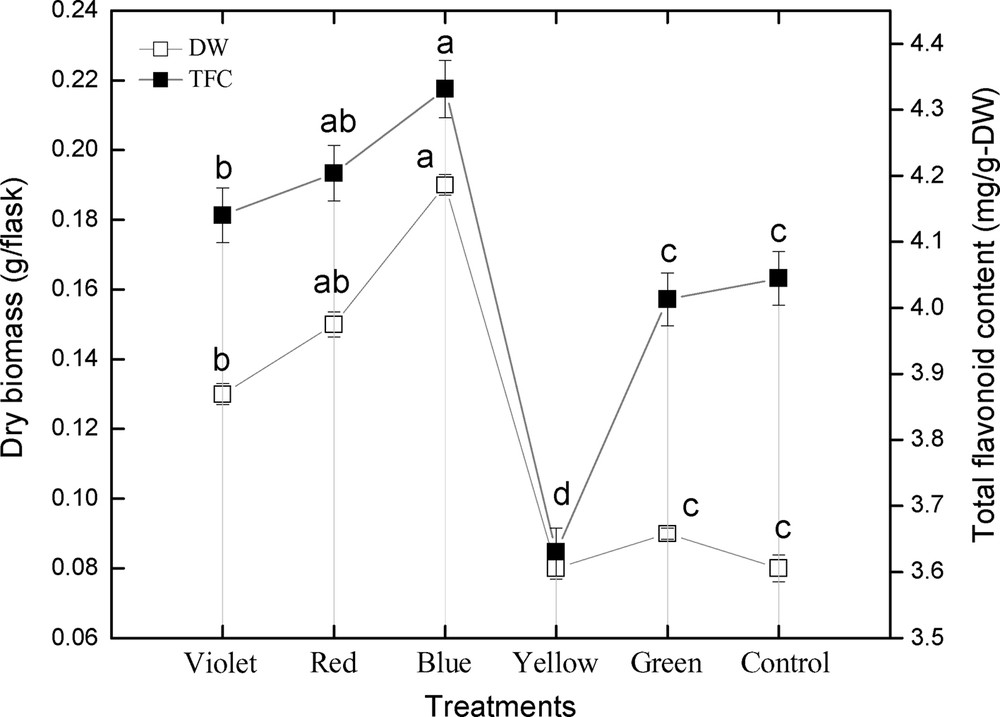
Correlation of total flavonoid content with dry biomass in adventitious root cultures of Stevia rebaudiana. Mean data with common alphabets and standard errors are significantly different at P < 0.05.
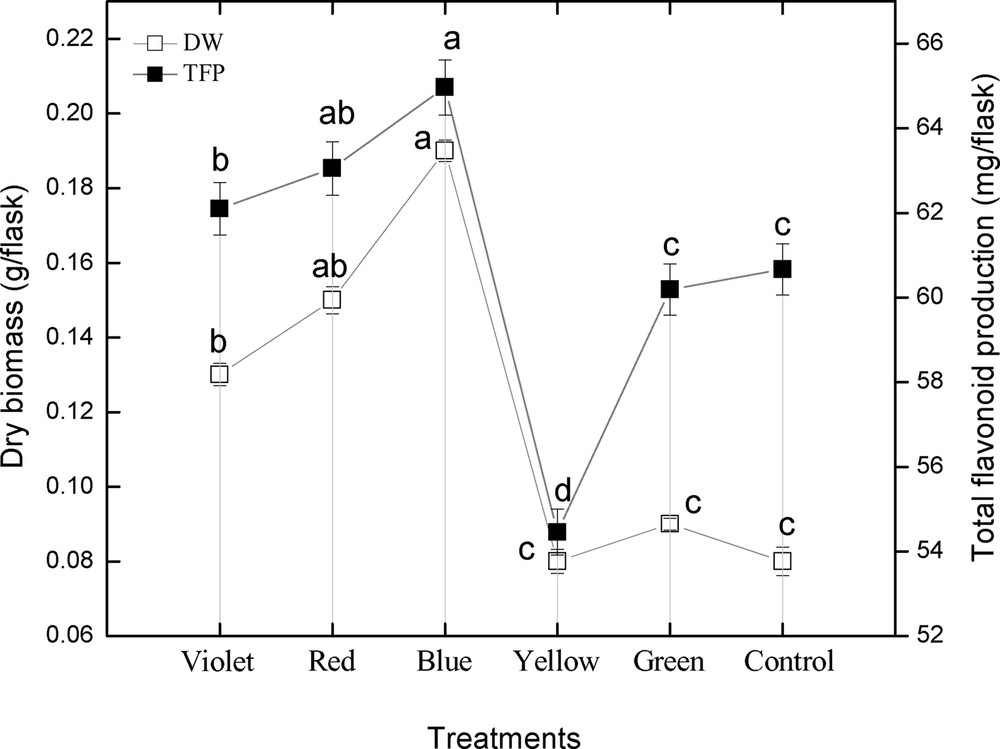
Correlation of total flavonoid production with dry biomass in adventitious root cultures of Stevia rebaudiana. Mean data with common alphabets and standard errors are significantly different at P < 0.05.
3.4 The effect of spectral lights on the DPPH radical scavenging activity and its correlation with polyphenolics content
The DRSA of adventitious root cultures of S. rebaudiana in response to various spectral lights was also investigated. Among the spectral tested lights, violet light showed the highest DRSA (79.72%), followed by blue light (78.32%), green and red light (76.60%), yellow (75.21%), and control (73.81%). These results showed that violet light showed the highest DRSA, while control cultures caused the lesser DPPH activity, which leads us to conclude that spectral lights make the biochemical pathways fluctuate, which leads to higher antioxidant activity (Figs. 7 and 8). The DRSA in this study was found independent of the TPC, TPP, TFC, and TFP (Figs. 7–8). The highest DPPH activity was observed under violet light, while the highest TPC, TPP, TFC and TFP values were observed under blue light. Likewise, the second highest DPPH activity was observed under blue light, while the second highest TPC and TFC were observed under red light. Further results also confirmed that there is no correlation of DPPH activity with TPC, TPP, TFC and TFP (Figs. 7–8).
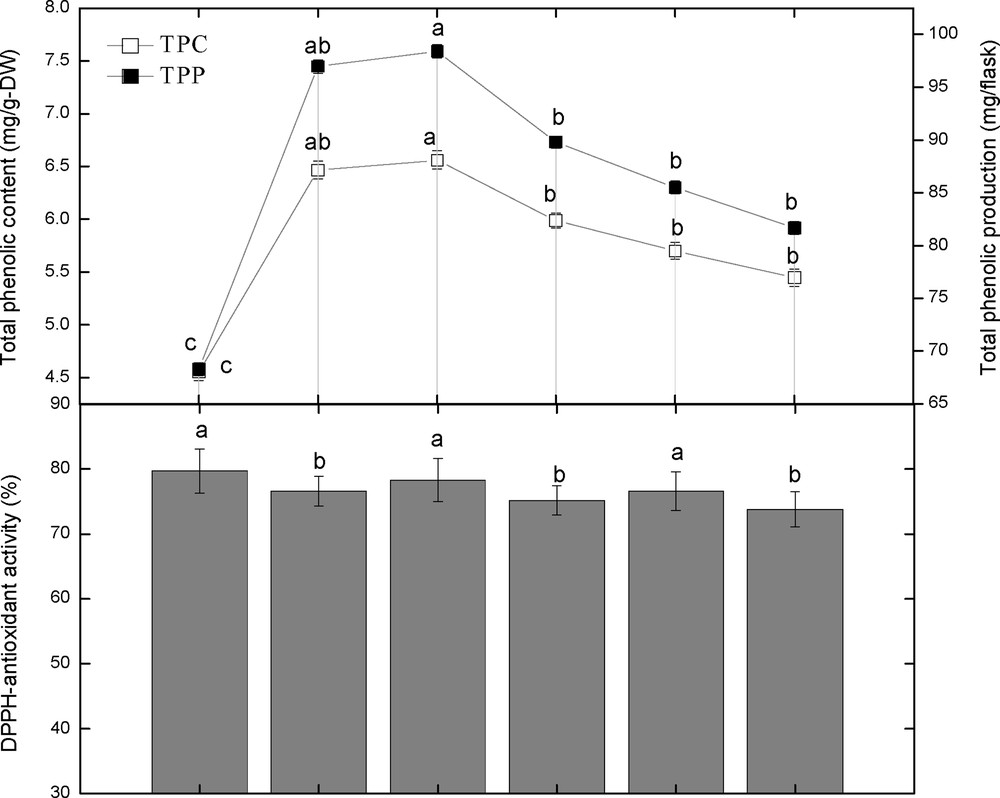
Correlation of total phenolic content and production with DPPH activity in adventitious root cultures of Stevia rebaudiana. Mean data with common alphabets and standard errors are significantly different at P < 0.05.
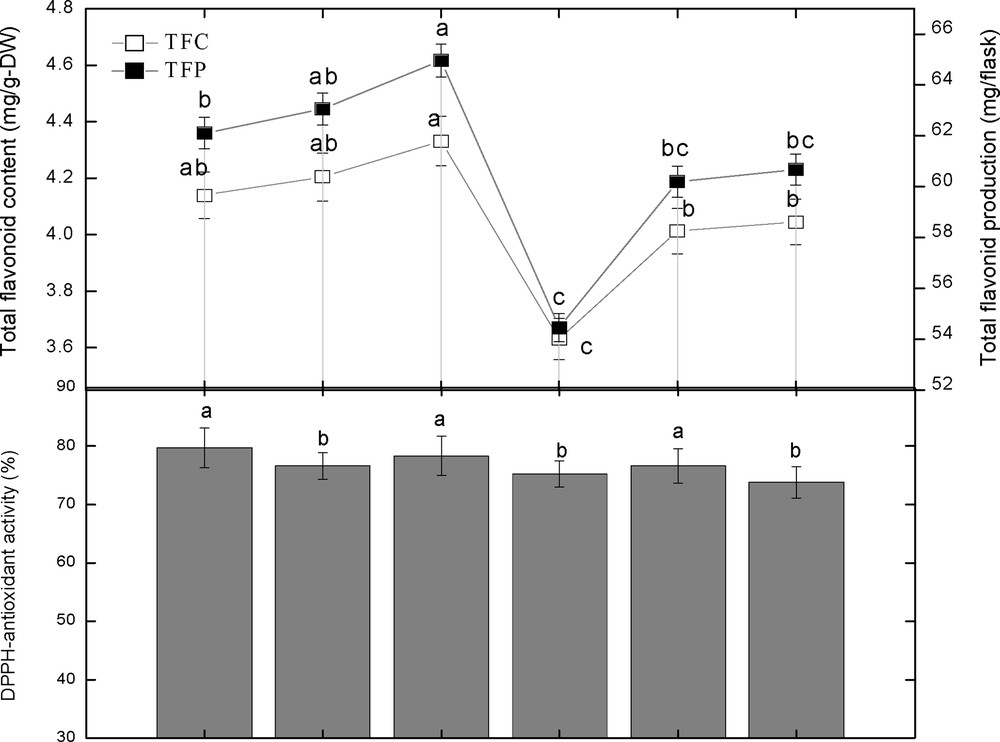
Correlation of total flavonoid content and production with DPPH activity in adventitious root cultures of Stevia rebaudiana. Mean data with common alphabets and standard errors are significantly different at P < 0.05.
4 Discussion
This study was carried out to determine the effect of different spectral lights on biomass accumulation and phenolics and flavonoids production in adventitious root cultures of S. rebaudiana. Among the different lights, the blue one induced the highest accumulation of TPC (6.56 mg GAE/g DW) as compared to control, while the least accumulation was observed in a flask exposed to violet light (4.55 mg GAE/g DW). Among the different tested lights, blue light induced the highest accumulation of TFC (4.33 mg RE/g DW) than control (4.04 mg RE/g DW), while the least biomass accumulation than control was observed for yellow light (3.63 mg RE/g DW). These results showed that blue light produced the highest accumulation of TFC while yellow produced the lesser TFC, which leads us to conclude that yellow has an inhibitory role among the other used spectral lights as well as the control light in Stevia root cultures. These results are in agreement with the studies conducted by Evans and Halliwell [25], who found that diosgenin production was boosted in the callus culture of Dioscorea deltoidea by blue light. Similarly, Ahmad et al. [26] studied the callus cultures of S. rebaudiana produced maximum accumulation of TPC and TFC under blue lights as compared to control. They also studied the correlation between TPC and TFC, which is similar to the current study.
The reports of spectral lights on TPC and TFC vary from species to species; this might be due to the differences in light sources and intensities and varies according to the explant source [19]. Green and yellow lights as compared to control enhanced the total phenolic and flavonoid production. In contrast, Fazal et al. [27] found that blue light boosted the total phenolic content (TPC; 23.9 mg/g-DW) and total flavonoid content (TFC; 1.65 mg/g-DW) in in vitro cultures of Prunella vulgaris. They also found that calli grown under green, red and blue lights enhanced the DPPH-free radical scavenging activity (DRSA; 91.3%, 93.1% and 93%) compared with the control one (56.44%).
In the present study, the effects of white light and of different monochromatic lights such as violet, red, blue, yellow, and green on biomass accumulation have been determined in adventitious root cultures of Stevia rebaudiana. It has been concluded that violet light produced 2.495 g/flask of fresh biomass on day 27 in log phase, which is the highest biomass accumulation, followed by green light (1.94 g/flask), yellow (1.895 g/flask), blue (1.702 g/flask), control (1.625 g/flask), and red (1.025 g/flask) light. The current study makes us conclude that spectral lights, especially violet light, are more effective for biomass accumulation than control. In contrast, Fazal et al. [27] observed that maximum biomass accumulation was induced by yellow light (3.67 g/100 ml) from leaf explants in comparison to control, while from petiole explant, violet lights induced the maximum biomass accumulation (3.49 g/100 ml). Similarly, Ahmad et al. [26] studied that the maximum callogenic response (92.73%) was observed by using control light compared to yellow, blue, green, and red lights. However, the similarity between the present study and that of Ahmad et al. [26] is that red light was found less effective in callus induction or biomass accumulation in S. rebaudiana. Different results regarding the effect of spectral lights in biomass accumulation and plant growth were observed by Senger [28], who found that chloroplast development, stomata opening, and formation of chlorophyll has been positively affected by blue light. Moreover, the growth of lettuce was enhanced by blue light too. Further, it was found that the morphogenesis of plants such as wheat and cymbidium was enhanced by red and blue light [29,30]. In another study, it was found that blue light boosted growth as compared to white light [31]. Similar results were found in the hairy root cultures of Artemisia annua, in which red light enhanced the biomass accumulation and production of artemisinin [32]. Moreover, Fazal et al. [27] found that yellow and violet lights boosted fresh biomass accumulation in Prunella cultures. Yellow light was found to be the most effective in biomass accumulation (3.67 g/100 ml) as compared to control (1.27 g/100 ml). Similarly, the biomass accumulation from petiole explants was enhanced by violet light.
5 Conclusion
Stevia rebaudiana is a sweet herb, which is famous for the biosynthesis of calorie-free sweet steviol glycosides. But their growth is limited by poor seed germination and lesser survival of stem cuttings. Plant cell, tissue organ culture is a good approach for plantlet development as well as secondary metabolites production. In this study, various spectral lights were applied to test their effects on biomass accumulation as well as secondary metabolite biosynthesis. Herewith, violet light was found to be the best source for maximum biomass accumulation (2.495 g/flask) as compared to control (1.63 g/flask). Blue light was found effective for phenolics synthesis and production. Blue light also improved the accumulation of flavonoids and total flavonoid production as compared to control. In a fashion similar to biomass accumulation, violet light also enhanced the antioxidant activity. In conclusion, spectral lights, especially blue and violet lights, were effective sources for biomass as well as for biosynthesis of secondary metabolites in adventitious root cultures of S. rebaudiana.