1 Introduction
Transcription factors are important intracellular regulators and mediators of the action of extracellular stimuli on ovarian functions. The gene constructs of transcription factors may serve as important tools to study their roles and for the regulation of reproductive processes and treatment of reproductive disorders [1,2]. The transcription factor cAMP response element-binding protein type 1 (CREB-1) is involved in the upregulation of ovarian cell proliferation [3] and viability [4] and downregulation of their apoptosis [5]. Its importance in the transcriptional activation of ovarian steroidogenic enzymes (StAR, aromatase) and subsequent estradiol and progesterone release [6–12] is well studied. However, the involvement of CREB-1 in the control of other non-steroidal ovarian hormones is questionable.
The phosphorylated isoform of CREB-1 represents only a small proportion of the intracellular CREB-1. Studies have shown the association between the increased level of CREB-1 in ovarian cells and sexual maturation [13], ovarian follicle development [8], gonadotropin treatment [3,9–12,14], and growth factor treatment [9,12,15–17]. Only the phosphorylated isoform of CREB-1 is thought to exert its physiological function. This hypothesis is confirmed by the fact that the transfection with the wild type CREB-1 cDNA construct (CREB-1 WT) had the opposite effect to that observed with the transfection of the nonphosphorylatable mutant of CREB-1 (CREB-1 M1) on cAMP responsible element in embryonal carcinoma F9 cells [18], the proliferation and survival of β cells [19], cAMP production by adrenocortical cells [20], and StAR and progesterone production by Leydig cells [21–23]. No studies have reported the role of CREB-1 phosphorylation in the control of other processes (e.g., apoptosis, release of non-steroidal hormones) and regulation of ovarian cell functions. The significance of CREB-1 phosphorylation may be elucidated by comparing the action of CREB-1 WT and CREB-1 M1 constructs on these processes.
Here, we examined the role of CREB-1 in the control of proliferation, apoptosis, and hormonal release of human ovarian cells and studied the significance of CREB-1 phosphorylation in the regulation of these processes. For this purpose, we examined and compared the effects of CREB-1 WT and CREB-1 M1 transfection on the proliferation and apoptosis of human ovarian granulosa cells and release of hormones such as progesterone, oxytocin, prostaglandin F2 alpha (PGF2), prostaglandin E2 (PGE2), and insulin-like growth factor I (IGF-I).
2 Materials and methods
2.1 Preparation, culture, and processing of ovarian cells
Granulosa cells were harvested 1–5 days after spontaneous ovulation from women aged 36–42 years with normal ovarian cycles and morphology. These women were undergoing ovariectomy at the Faculty Hospital Nitra, owing to the non-metastatic cancer of the cervix uteri. Informed consent was obtained from patients in accordance with EU and Slovak ethical and medical regulations under the official agreement and supervision of the local ethical committee. Granulosa cells were isolated from follicles 2–8 mm in diameter without visible signs of atresia and processed as previously described [24,25]. CREB-1 WT and CREB M1 constructs were produced by Prof. R. Goodman and cloned by Dr. J.M. Kornhauser (Howard Hughes Medical Institute, Oregon Health and Science University, Portland, USA). FlagCREB was subcloned into pcDNA3, wherein the cytomegalovirus (CMV) promoter was replaced by a Rous sarcoma virus (RSV) promoter to generate pCD/RSV-FlagCREB for mammalian expression. In addition, FlagCREB was subcloned into pET16b to generate pET16b-FlagCREB for bacterial expression. The characteristics of these constructs are described by Kornhauser et al. [26]. The following plasmids were subcloned from pcDNA3/pcCREB-1 WT-SN3 and used for the transfection of granulosa cells:
- • an expression vector for CREB-1 WT construct containing ampicillin resistance gene;
- • a vector for CREB M1;
- • a “scramble” control plasmid vector without CREB-1 insertion kindly provided by Prof. N.D. Perkins (University of Dundee, Dundee, UK);
- • a marker reporter plasmid pEGFP-N1 for green fluorescent protein (EGFP) and resistance to kanamycin (Clontech, Mountain View, CA, USA).
These plasmids were multiplied as previously described [24]. Granulosa cells were processed and cultured as it was described previously [25,27]. Briefly, after aspiration from ovarian follicles, granulosa cells were suspended in DMEM/Ham's F-12 1:1 mixture (Gibco-Invitrogen, Carlsbad, CA, USA) and the suspension (2 × 106 cells/mL, determined by hemocytometer) was placed in 16-well chamber slides (Nunc International, Naperville, USA, 100 μL/well). Immediately after the placement into the chamber slides, an experimental group of cells was transfected with a gene construct encoding EGFP and CREB-1 WT or CREB M1. A control group of granulosa cells was transfected with a gene construct encoding EGFP and the same but insert-less “scramble” plasmid vector. Transfection was performed using transfection reagent Roti-Fect (Carl Roth, Karlsruhe, Germany) according to the manufacturer's instructions. After transfection, cells were diluted with culture medium DMEM/Ham's F-12 1:1 mixture supplemented with 10% bovine fetal serum and 1% antibiotic-antimycotic solution (all from Gibco-Invitrogen) to 0.5 × 106 cells/mL and cultured 48 h in 200 μL of culture medium/well. After incubation, cell numbers and viability were determined by the trypan blue staining using a hemocytometer; the viability was 70–80%. No statistically significant differences were observed in these indices between control and experimental groups. The medium from the chamber slides was removed and frozen at −18 °C for enzyme immunoassay (EIA) and immunoradiometric assay (IRMA). The cell monolayer at the well bottom was fixed for subsequent immunocytochemical analysis [25,27].
2.2 Immunocytochemical analysis and western immunoblotting
We detected total and phosphorylated CREB-1 as well as the marker and promoter of proliferation (proliferating cell nuclear antigen [PCNA]) [28] and cytoplasmic apoptosis (bax) [29,30] with immunocytochemistry [25,27,31]. Primary mouse monoclonal antibodies against non-phosphorylated and phosphorylated CREB-1, PCNA, or bax (Santa Cruz, Santa Cruz, CA, USA; dilution 1:500) that cross-reacted with the corresponding antigens of human, rat, mouse, pig, and yeast origin were used. Secondary porcine polyclonal antibodies against mouse IgGs, labeled with fluorescein isothiocyanate (FITC, Sevac; dilution 1:1000) were used. Cells processed without the primary or secondary antibody were used as negative controls. Cells containing FITC and EGFP were detected using a fluorescence microscope (Leica GmbH, Wetzlar, Germany). The cells containing EGFP, non-phosphorylated CREB-1, PCNA, and bax are presented in Fig. 1. The presence and specificities of the antigens in the selected cultures and corresponding antisera were validated by western immunoblotting by using the antisera listed above, secondary polyclonal rabbit antibodies raised against mouse, labelled with horseradish peroxidase (Sevac, Prague, Czech Republic; dilution 1:1000), Upstate Visualizer Western Blot (Temecula, CA, USA) and ECL Hyper-film (Amersham International plc, Little Chalfont, Bucks, UK) as previously described [16,27].
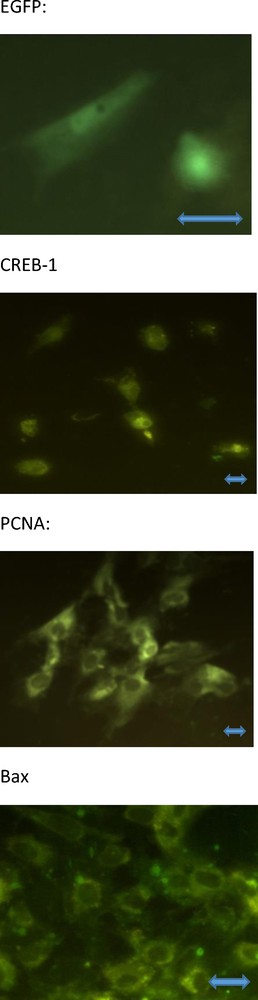
The cultured human ovarian granulosa cells containing EGFP (A), non-phosphorylated CREB-1 (B), PCNA (C), and bax (D); green fluorescence. No signal from phosphorylated CREB-1 has been visible (the invisible cells are not shown). Fluorescence microscopy. Magnification ×800 (A), ×400 (B, C) and ×600 (D). The scale bars indicate 10 μm.
2.3 Immunoassays
Progesterone, oxytocin, PGF, and PGE were assayed using 25–100 μL of the incubation medium with our own EIA systems [32–34]. The concentration of PGF metabolite (PGFM) in the medium reflects PGF2 secretion (r = 0.92; P < 0.001) from cultured cells [33]. Rabbit polyclonal antisera against OT, PGE2, and PGFM were kindly donated by Professors G. Kotwica (University of Warmia and Mazury, Olsztyn, Poland), W.W. Thatcher (University of Florida, Gainesville, FL), and W.J. Silvia, (University of Kentucky, Lexington, USA), respectively. IGF-I was assayed using IRMA kits from DSL (Webster, USA), according to the manufacturer's instructions. The characteristics of these assays are presented in Table 1.
Characteristics of the immunoassays used in experiments.
Substance assayed | Specificity of assay (cross-reactivity of antiserum) | Sensitivity of assay (ng/mL) | Coefficient of variation | |
Intra-assay | Inter-assay | |||
Progesterone | < 0.001% to cortisol, pregnenolone, oestradiol, 5β-Pregnandione, 16α-, 17α-, 6β-Hydroxyprogesterone, testosterone | 0.05 | 5.8 | 9.0 |
IGF-I | < 0.001% to insulin, proinsulin, IGF-II and GH. | 2,0 | 6.3 | 6.8 |
PGF | < 0.01% to PGA-1, PGA-2, PGB-1, PGB-2, < 0.1% to PGE-1, PGE-2, 66% to PGF-1, 100% to PGF-2 | 0.003 | 10.5 | 5.6 |
PGE | < 28.0% to PGA-1, < 7.0% to PGA-2, < 0.6% to PGB-1, < 1.4% to PGB-2, < 5.0% to PGF-1, < 1.5% to PGF-2, 165% to PGE-1, 100% to PGE-2 | 0.015 | 7.5 | 4.0 |
Oxytocin | < 0.01% to arginine-vasopressin, lysine.-vasopressin, arginine-vasotocin, ghrelin, leptin and FSH | 0.12 | 13.1 | 8.0 |
2.4 Statistics
Each experiment was repeated thrice, and the data are shown as the means of values obtained from four separate experiments performed on separate days with separate groups of granulosa cells, each obtained from two women (four ovaries). For quantitative immunocytochemistry, at least 100 cells were scored in each chamber (three per group) and each value represented the mean of nine replicates (at least 1000 cells in total). In EIA/IRMA, each group was represented by four culture wells and the data represent the mean of 12 replicates. Assays evaluating the hormonal content of the incubation medium were performed in duplicates. After EIA/IRMA, the values of blank control (medium cultured without cell) were subtracted from the values determined in the cell-conditioned serum-supplemented medium to exclude any non-specific background (less than 10% of total values). The rate of substance secretion was calculated for 106 cells/day. Variance homogeneity and significant differences between the experiments and groups were evaluated using one-way analysis of variance (ANOVA) followed by Wilcoxon–Mann–Whitney test using Sigma Plot 11. 0 software (Systat Software, GmbH, Erkhart, Germany). A value of P < 0.05 was considered statistically significant.
3 Results
At the end of the experiment, cultured ovarian cells contained markers of transfection (EGFP and non-phosphorylated CREB-1), proliferation (PCNA), and apoptosis (bax). Microscopic inspection revealed the localization of EGFP and CREB-1 in cytoplasm and nuclei, while PCNA and bax were mainly localized in the cytoplasm; no visible differences between the groups in this localization has been found (Fig. 1). Immunocytochemistry and western immunoblotting failed to detect any visible signal for phosphorylated CREB-1 in either cell group (invisible cells and gel fractions without signal have not been shown).
In comparison with the control cells (transfected with the “scramble” control plasmid), those transfected with either CREB-1 WT or CREB-1 M1 cDNA construct showed a significant increase in the percentage of cells containing non-phosphorylated CREB-1 and PCNA. The proportion of cells containing bax significantly decreased in cells transfected with CREB-1 M1, but not in cells transfected with CREB-1 WT construct. CREB-1 WT significantly reduced the progesterone release; on the contrary, CREB-1 M1 failed to alter the release of progesterone. Both CREB-1 constructs reduced oxytocin output. The release of PGF was decreased by both CREB-1 WT and CREB-1 M1 construct. PGE output dramatically reduced in cells transfected with CREB-1 WT or CREB-1 M1. The release of IGF-I significantly reduced upon transfection with CREB-1 WT or CREB-1 M1 construct (Fig. 2).

Effect of transfection with CREB WT and CREB M1 cDNA constructs on the percentage of cultured human granulosa cells containing non-phosphorylated CREB-1 (A), phosphorylated CREB-1 (B), the proliferation marker PCNA (C), the apoptosis marker bax (D) and the release of prostaglandin F 2 alpha (E), prostaglandin E2 (F), progesterone (G), oxytocin (H) and IGF-I (I) as determined by immunocytochemistry (A–D) and EIA/RIA (E–I). Data are the mean ± S.E.M. *–effect of transfection, significant (P < 0.05) differences between the cells transfected and not transfected with CREB WT or CREB M1 gene constructs.
4 Discussion
4.1 Was our transfection successful?
The accumulation of the marker protein EGFP within the cells suggests the incorporation and expression of the cDNA construct. Thus, the transfection was successfully performed. The increase in the percentage of cells containing non-phosphorylated CREB-1 upon transfection with either CREB-1 WT or CREB-1 M1 construct demonstrate that both constructs induced the overexpression of total CREB-1. On the other hand, no signal of phosphorylated CREB from control cells and those transfected with the two constructs, as observed with immunocytochemistry and western immunoblotting, suggests that no or only a small proportion of CREB-1 was phosphorylated.
4.2 How does CREB-1 affect ovarian cell proliferation?
The overexpression of either CREB-1 WT or CREB-1 M1 was associated with an increase in the percentage of cell proliferation (PCNA-positive). Our observations provide the first evidence that CREB-1 may upregulate ovarian granulosa cell proliferation. These observations are in line with those reported by Vazquez-Cuevas et al. (2010) using human theca-interstitial cells, wherein proliferation was promoted by a construct encoding nonphosphorylatable CREB-1. Linnert et al. (2008) also showed that CREB-1 suppression with RNA interference reduced the proliferation of human ovarian tumor cells. The available data suggest that CREB-1 promotes the proliferation of various ovarian cell types. As ovarian cancer is associated with CREB-1 augmentation, CREB-1 overexpression may cause over-multiplication and malignant transformation of ovarian cells [35,36].
4.3 How does CREB-1 affect ovarian cell apoptosis?
We observed that CREB-1 M1, not CREB-1 WT, construct promoted granulosa cell apoptosis (bax accumulation). This observation is in line with the report on the ability of CREB-1 M1 to reduce the viability of rat granulosa cells [4]. In addition, CREB-1 WT and CREB-1 M1 were shown to promote apoptosis in human and sheep ovarian surface epithelium, but not human ovarian cancer cells [5,35]. These studies suggest the pro-apoptotic action of CREB-1 on various types of ovarian cells. However, the pro-apoptotic action of CREB-1 may be inapplicable for ovarian cancer treatment.
4.4 How does CREB-1 affect ovarian hormones?
Studies have highlighted the stimulatory action of CREB-1 WT and the inhibitory action of CREB-1 M1 on human and rat ovarian progesterone production [6–12]. In our experiments, CREB-1 WT (but not CREB-1 M1) inhibited progesterone release, thereby opposing the previously reported results.
Transfection with CREB-1 WT or CREB-1 M1 construct decreased oxytocin, PGF, PGE2, and IGF-I release. These observations represent the first demonstration of the involvement of CREB in the control of ovarian non-steroidal hormone release. As these hormones, like steroids, play a key role in the control of ovarian cell proliferation, apoptosis, oogenesis, folliculogenesis, and fecundity [37], CREB-1 may be involved in the control of these hormone-dependent processes. The pro-proliferative and pro-apoptotic action of CREB-1 demonstrated in our and previous experiments may be explained by the influence of CREB-1 on these hormones. The action of CREB-1 on ovarian folliculogenesis and fecundity and its possible hormonal mediators requires further studies, which may be important from molecular biology, reproductive medicine, and assisted reproduction point of view.
4.5 Is phosphorylation necessary for CREB-1 action on the ovarian functions?
The presence of total CREB-1 in human ovarian cells and the failure to detect visible phosphorylated CREB-1 in our experiments indicate that only a small proportion of total CREB-1 (if any) was phosphorylated. Both CREB-1 WT and CREB-1 M1 constructs induced CREB-1 overexpression, promoted cell proliferation, and decreased oxytocin, PGF, PGE2, and IGF-I release. In some cases, the effect of CREB-1 M1 was larger than that of CREB-1 WT. The weak CREB-1 phosphorylation and similar action of phosphorylatable and nonphosphorylatable CREB-1 on the majority of the evaluated parameters suggest that CREB-1 phosphorylation has no decisive role in the control of these parameters. On the other hand, apoptosis was affected only by CREB-1 M1, and progesterone was influenced only by CREB-1 WT, suggestive of the importance of CREB-1 phosphorylation/dephosphorylation for mediating action on these processes. The different or opposite action of phosphorylatable and nonphosphorylatable CREB-1 on these parameters suggests that phosphorylation of CREB-1 may be important in some ovarian cell functions. On the other hand, taken together, CREB-1 phosphorylation/dephosphorylation had no effect on six of the eight parameters studied in our experiments. Two of the three unaffected parameters were influenced only by dephosphorylated CREB-1. Our observations are in line with those of Vazquez-Cuevas et al. [3] who reported promotion of human ovarian cell proliferation by a construct encoding nonphosphorylatable CREB-1. These observations indicate that phosphorylation plays no decisive role in mediating CREB-1 action on the majority of ovarian processes and that, in some cases, dephosphorylation itself makes CREB-1 biologically active. Further detailed studies on CREB-1 phosphorylation and its consequences may be required to explain these phenomena. Thus, CREB-1 gene constructs are deemed useful for the understanding and practical application of CREB-1 action on ovarian and non-ovarian functions.
Taken together, our study confirms the involvement of CREB-1 in the control of ovarian cell proliferation, apoptosis, and steroidogenesis. This is the first study to demonstrate the involvement of CREB-1 in the control of the release of ovarian non-steroidal hormones—oxytocin, PGF2, PGE2, and IGF-I. The similarity in the effects of CREB-1 WT and CREB-1 M1 and the biological activity of only CREB-1 M1 suggest that phosphorylation has no effect on the action of CREB-1 on the majority of analyzed human ovarian cell functions.
Author's contribution
A.V. Sirotkin generated the general concept of the study and the resulted manuscript, A. Benčo performed the in vitro experiments, M. Mlynček provided biological material for experiments, A.H. Harrath and S. Alwasel provided funding, contributed to the generation and editing of the manuscript, J. Kotwica performed hormone analysis.
Funding
This work was supported by Slovak Research and Development Agency (APVV) under the contract numbers APVV-0854-11, APVV-14-001, and APVV-15-0296 and by the Slovak Grant Agency of the Ministry of Education, Science and Sport and of the Slovak Academy of Science (VEGA), projects VEGA 1/0392/17, VEGA 1/0022/15, and VEGA 1/0327/16. The authors would also like to extend their sincere appreciation to the Deanship of Scientific Research at King Saud University for funding this research RG-164.
Disclosure of interest
The authors declare that they have no competing interest.
Acknowledgements
The authors would like to thank Ms. K. Tothová and Ž. Kuklová for their technical assistance and Prof. Dr. N. Perkins (University of Dundee, Dundee, UK) for providing them with the control plasmids. The authors would also like to thank Dr. J.M. Kornhauser (Oregon Health and Science University, Portland, USA) for providing with CREB-1 WT and CREB-1 M1 constructs as well as DR. D. Vasicek (Research Institute of Animal Production, Nitra, Slovakia) for their cloning. Furthermore, the authors express their gratitude to Professors G. Kotwica (University of Warmia and Mazury, Olsztyn, Poland), W.W. Thatcher (University of Florida, Gainesville, FL), and W.J. Silvia, (University of Kentucky, Lexington, USA) for kind provision of antisera against OT, PGE2, and PGFM.