1 Introduction
Cadmium (Cd) is one of the most toxic pollutants found in the air, water and soil and is regarded as a non-essential metal without any known physiological function, although a cadmium-containing carbonic anhydrase has been described in diatoms [1]. This ion induces complex changes in plants at the genetic, biochemical and physiological levels leading to phytotoxicity [2]. The presence of Cd in plants results in many physiological alterations affecting both nitrogen and carbohydrate metabolism [3], and induces alterations in the functionality of membranes, by triggering changes in their lipid composition [4]. It was shown that Cd-induced toxicity involves some other senescence-like processes, such as the participation of oxidative stress [5,6]. During seed germination, heavy metal damage is suspected to be related to oxidative processes, as indicated by high levels of reactive oxygen species-scavenging enzymes as well non enzymatic antioxidant components such as glutathione and tocopherols [7–11]. The reduced form of cytosolic NADP is produced in the pentose phosphate pathway and plays critical roles in the generation of reactive oxygen species (ROS) and in the production of anti-oxidants as ROS scavengers [12,13]. This work aimed to examine the impact of imbibition with CdCl2 on germination rate, embryonic axis growth, NAD(P) levels and NAD(P)H oxidase activities in mitochondrial and peroxisomal fractions of cotyledons and embryonic axis.
2 Materials and methods
2.1 Germination conditions and cadmium treatment
Germination conditions, Cd treatment and extraction of protein from pea (Pisum sativum L. cv. douce province) seeds were as previously described [14].
2.2 Extraction and quantification of coenzymes
Reduced forms (NADPH and NADH) and oxidized ones (NADP+ and NAD+) were extracted according to the method of [15]. For the coenzymes quantification, enzyme cycling assays were employed [16].
2.3 Enzyme assays
The activities of NADH oxidase (EC 1.6.99.3) and NADPH oxidase (EC 1.6.99.6) [17] were measured spectrophotometrically in a total volume of 500 μL at 25 °C. Each of the above assays was used only in a range in which the rate of reaction was proportional to the amount of extract added.
2.4 Statistics
The data reported are the mean values (± S.E.) of six replicates. Statistical analysis was performed by ANOVA test (p < 0.05).
3 Results
3.1 Effect of Cd on seed germination
The sensitivity to Cd was examined with regard to (a) the germination rate for 5 days and (b) the behaviour of redox and oxidative properties. During the first day after the beginning of imbibition with metal solution a marked decrease in seed germination success was observed, and after 5 days reached a 60% of decrease from the control (Fig. 1). Analysis of embryo length showed significant differences between seeds germinating on water and Cd. After 5 days, embryo length reached a 75% of decrease from the control. The inhibitory effect of metal increased in a time-dependent manner (Fig. 1).
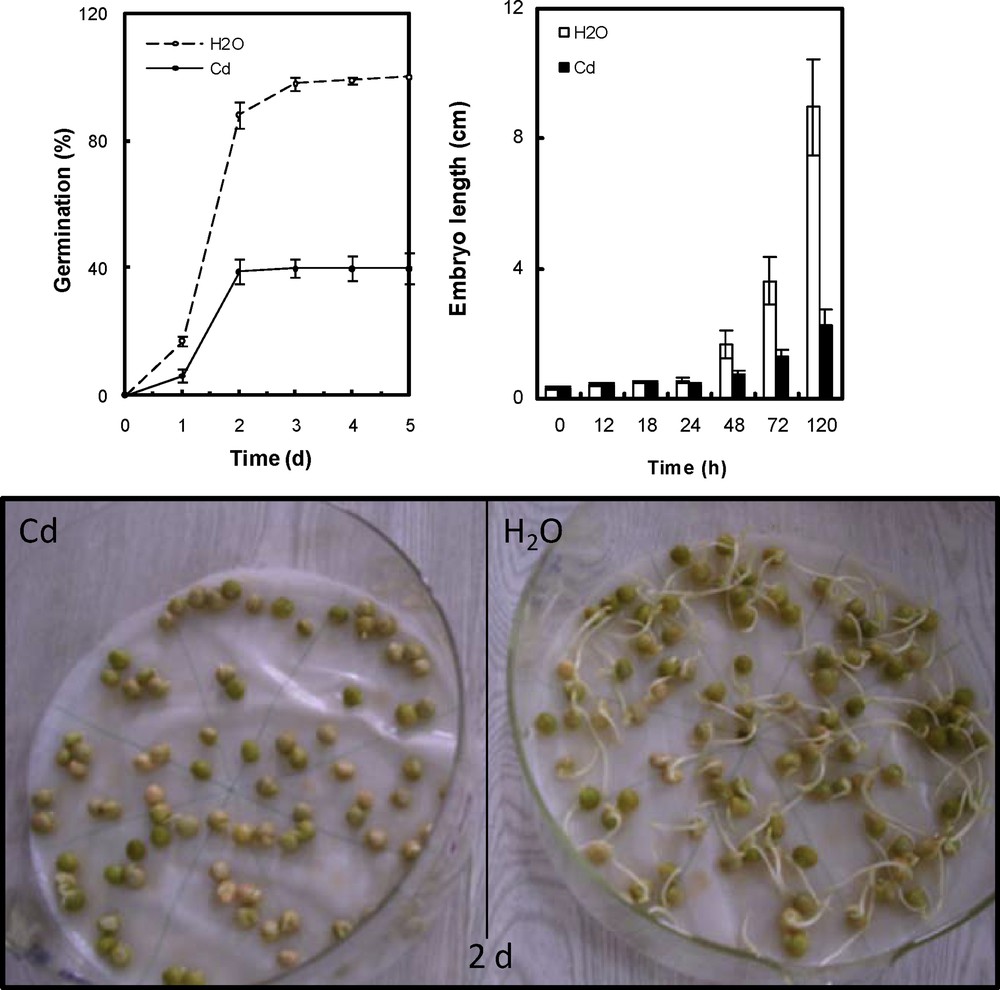
Germination rate and embryonic axes length of P. sativum seeds after imbibition with H2O or 5 mmol L−1 CdCl2. Data are the mean of two independent experiments. Each experiment was carried out with 80 germinating seeds.
3.2 Effect of Cd on the NAD(P) patterns
The NAD(P) levels of cotyledons and embryonic axis are seen to undergo a significant increase during H2O imbibition (Figs. 2 and 3). Cd affected the oxidized nicotinamide (NAD(P)+) pools in the peroxisome of both cotyledons and embryonic axis and in the mitochondria of the embryo axis, but not the cotyledons (Fig. 2). In the embryos, the NAD(P)+ content in Cd-treated mitochondria and peroxisome was ∼35% lower than that of control one after 5 days of Cd exposure (Fig. 2b,d). In the cotyledons, it was ∼35% lower in Cd-treated peroxisome, but ∼55% higher in Cd-treated mitochondria (Fig. 2a,c).

Content of NAD(P)+ in peroxisomal fractions (A, cotyledons; B, embryonic axis) and mitochondria (C, cotyledons; D, embryonic axis) of P. sativum seeds during germination after imbibition with H2O or 5 mmol L−1 CdCl2. Data are the means (± S.E.) of 6 replicates. Each measurement was performed in an extract obtained from several germinating seeds.
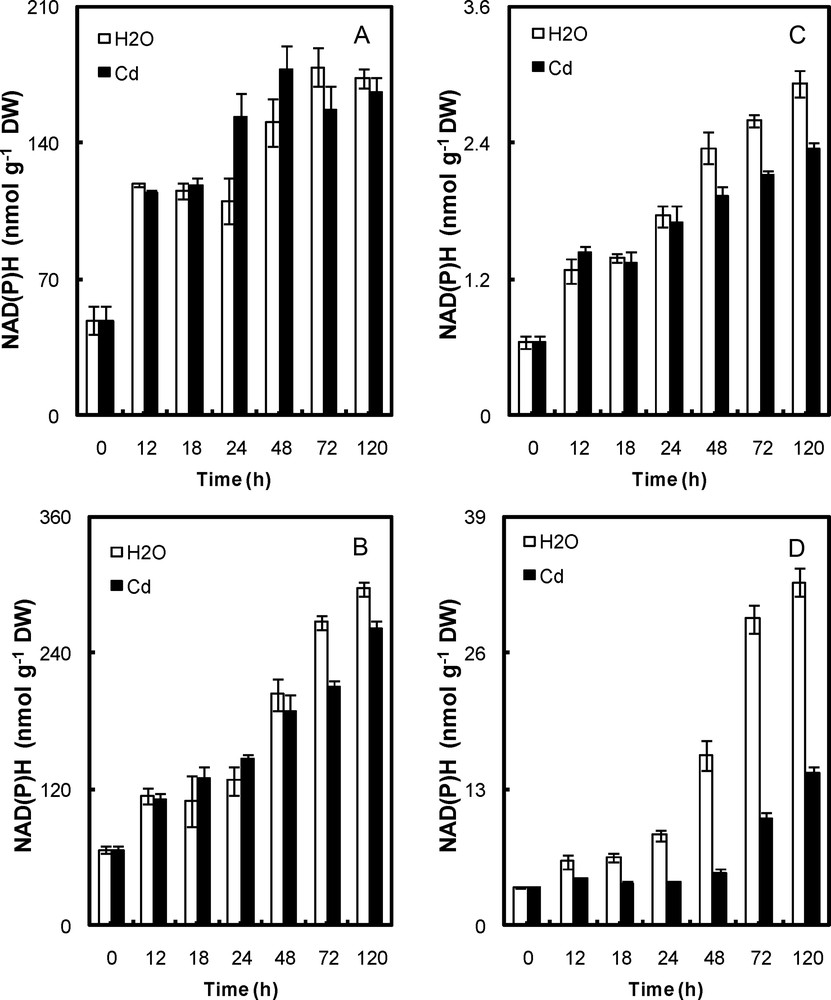
Content of NAD(P)H in peroxisomal fractions (A, cotyledons; B, embryonic axis) and mitochondria (C, cotyledons; D, embryonic axis) of P. sativum seeds during germination after imbibition with H2O or 5 mmol L−1 CdCl2. Data are the means (± S.E.) of 6 replicates. Each measurement was performed in an extract obtained from several germinating seeds.
Cd affected the reduced nicotinamide (NAD(P)H) pools in the mitochondria of both cotyledons and embryonic axis and in the peroxisome of the embryo axis, but not the cotyledons (Fig. 3). The NAD(P)H content in Cd-treated mitochondria and peroxisome of embryos was ∼30–50% lower than that of control ones (Fig. 3b,d). In cotyledons, it was ∼12% lower in Cd-treated mitochondria, but did not change in Cd-treated peroxisome (Fig. 3a,c).
3.3 Effect of Cd on the NAD(P)H- oxidase activity
In Cd-treated peroxisomal fractions, the NADH- oxidase activity was ∼150–789% higher than that of control (water-treated) in embryonic axes and cotyledons, respectively, after 5 days of exposure (Fig. 4a,b). In Cd-treated mitochondria, it was ∼38–98% higher than that of control (water-treated) (Fig. 4c,d). The NADPH- oxidase activity in Cd-treated mitochondrial and peroxisomal fractions was ∼200% higher than that of control (water-treated) fractions of both tissues after 5 days of exposure (Fig. 5).

NADH oxidase activity in peroxisomal fractions (A, cotyledons; B, embryonic axis) and mitochondria (C, cotyledons; D, embryonic axis) of P. sativum seeds during germination after imbibition with H2O or 5 mmol L−1 CdCl2. Data are the means (± S.E.) of 6 replicates. Each measurement was performed in an extract obtained from several germinating seeds.
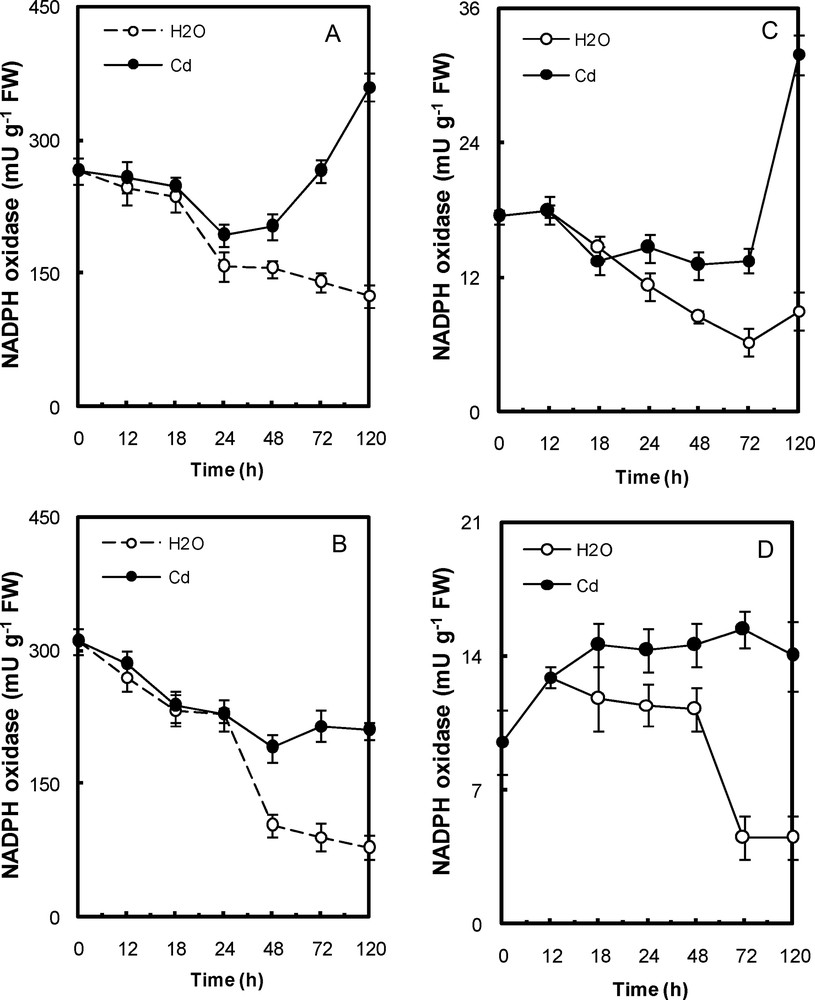
NADPH oxidase activity in peroxisomal fractions (A, cotyledons; B, embryonic axis) and mitochondria (C, cotyledons; D, embryonic axis) of P. sativum seeds during germination after imbibition with H2O or 5 mmol L−1 CdCl2. Data are the means (± S.E.) of 6 replicates. Each measurement was performed in an extract obtained from several germinating seeds.
4 Discussion
To focus on the mechanisms of heavy metals toxicity, capacity of moisture, activity of hydrolases in germinating seeds of Pisum sativum and Phaseolus vulgaris cultivars have been previously studied by our co-workers [18,19]. Moreover, previous study has demonstrated that Cd inhibited several mitochondrial enzyme activities and, consequently, delayed the resumption of respiration in germinating pea seeds [14,20,21]. Here, we demonstrated that the alterations in redox status can also explain the deleterious effects of cadmium stress.
The coenzyme levels show a number of significant features in relation to seed germination. Of the two nicotinamide forms, NAD(P)+ was the most abundant (Figs. 2 and 3). It increased from 30 and 100 nmol mg−1 DW (in the mitochondria and peroxisome, respectively) in dry seeds to 300 and 1000 nmol mg−1 DW at 5 days of germination. The concentrations of NAD(P)H increased from 3 and 60 nmol mg−1 DW in dry seeds to 30 and 300 nmol mg−1 DW at 5 days. The high increase in NAD(P) content at germination may represent a reducing equivalent available for the rapid and extensive increase in respiratory activity that accompanies germination. These high NAD(P) concentrations are consistent with the rapid rise in ATP level noted for germinating seeds following imbibition [22,23].
Alteration of coenzyme patterns can be due to the disorder in respiratory activity in mitochondria under Cd-stress condition (Figs. 2 and 3). Engagement of NADH dehydrogenases would allow efficient generation of ATP from NADH [24]. In previous work, activities of malate- and succinate-dehydrogenases and NADH- and succinate-cytochrome c reductases were rapidly inhibited in Cd-treated mitochondria [14,21]. The mitochondrial NAD(P) appears to play a fundamental role in controlling redox status in animals and plants subjected to abiotic stress [25,26]. Nevertheless, cytosol/mitochondrion exchanges should be considered as an important aspect of the redox state of mitochondria in Cd treated plants. Reducing power can be supplied via secondary NAD(P)H-recycling dehydrogenase activities. Cd stimulated the NADPH-generating enzyme activities of the pentose phosphate pathway, glucose-6-phosphate- and 6-phosphogluconate-dehydrogenases, as well as enzyme activity of fermentation, alcohol dehydrogenase [14,21]. During recent years, the existence of a regulatory network that modulates cytosol/mitochondrion exchanges has been argued [24,27]. In addition to the classical complexes, all plant mitochondria possess alternative enzymes that confer on them the capacity to oxidize external or internal NADH independently of complex I and to transfer electrons directly from reduced ubiquinone to oxygen via an alternative oxidase [28]. Alternative pathways are expected to provide additional flexibility to mitochondrial metabolism in situations of stress [29].
Moreover, plant peroxisomes have the capacity to reduce NADP+ to NADPH for its re-utilization in the peroxisomal metabolism, particularly in the antioxidative ascorbate-glutathione cycle of these organelles. It has been suggested that the tolerance to heavy metal toxicity is enormously dependent on the availability of reduced cell metabolites, such as NAD(P)H [30–32]. In the adult pea, it has been also demonstrated that peroxisomes respond to Cd toxicity by increasing the activity of antioxidative enzymes involved in the ascorbate-glutathione cycle and the NADP-dependent dehydrogenases located in these organelles [33–35].
On other hand, Cd caused a significant increase in oxidative stress parameters such as NAD(P)H oxidase activity. The NAD(P)H oxidase is involved in plant defense reactions to various abiotic stresses, such as Cd [34]. It appears that both NADP and NAD can be implicated in the response to Cd stress. The decrease in reduced nicotinamide would be a consequence of an oxidation reaction imposed by Cd. Treatment with metal resulted in significant increase in NAD(P)H oxidase activity (Figs. 4 and 5). We cannot exclude the possible role of the oxidase activity in the co-enzyme depletion. The particularly high activity of NAD(P)H oxidase may be the cause of the decrease in the coenzyme pools in Cd-poisoned tissues of the germinating pea seeds.
5 Conclusion
Environmental stress is a major factor that limits plant productivity. An increase in the NAD(P)H oxidase activity has been observed during the germination under Cd stress. The generation of an intracellular oxidative stress after Cd treatment caused a negative change in the cellular redox homeostasis, responsible for the decrease in the embryonic axes growth and delay in seed germination.
Conflict of interest statement
No conflicts of interest
Acknowledgments
Financial support for this work was received from the Tunisian Ministry of Higher Education, Scientific Research, and Technology (99/UR/09-18) and INRA-Henri Poincaré University (French). We also thank the anonymous reviewers for helpful comments on the manuscript.