1. Introductory remarks
Hominins evolution is not a linear process but the complex ramification of many lineages that diverged, coexisted and even sometimes partly merged. It is therefore difficult to define well delimited stages, as, at almost any given period of the past, several forms of Hominins coexisted on earth, usually in different parts of the globe, but sometimes in the same continental regions. Each of these lineages displayed its own mosaic of features, from anatomy to diet and from life history to social organization. Whenever one can analyze the evolution of such lineages, it is quite obvious that they display significant changes through time. For example, the endocranial organization of the earliest forms of Homo sapiens is closer from that of Neandertals than from that of late (so called “modern”) forms of Homo sapiens [1]. Furthermore, when analyzing hominin evolutionary processes, homoplasy (convergent evolution without recent common origins or reversal to primitive conditions) is a major issue that should not be overlooked. These changes are expected to originate from variations at the genome level which can be examined from entire sequences of fossil DNAs in recent forms such as Neandertals [2] and Denisovans [3] and/or by interpolation between extant apes and modern Humans. Even though, the deterministic relationships of precise genetic events which define brain circuits still largely escape our understanding, preliminary attempts have been made to more closely relate genome variations with the evolution of larger integrated entities that we have referred to as global “connectomic fundamentals” [4] such as brain size, long-range connectivity or extended postnatal synaptogenesis. Beyond genome, the intrinsic properties of developing tissues, interactions between adjacent anatomical regions and more widely integration phenomena also contributed to the neuroanatomy of different species of Hominins [5]. Finally, from a paleontological point of view, one can essentially deal with size, shape, patterns of development and cultural artefacts but brain connectivity and language are issues notoriously difficult to address. As a consequence, the present attempt to more closely relates paleoanthropology and brain evolution—even if of interest—shall remain partly speculative.
2. Australopiths
Australopiths represent a paraphyletic group documented since 4.2 My ago and from which the genus Homo arose ca. 2.8 My ago [6]. The last advances on Australopiths brain evolution demonstrate that this group of species is characterized by brain sizes in average 30% bigger than the means measured among common chimpanzees [7]. However, the anatomy of the basicranial foramina shows that brain blood flow rate was lower and therefore brain metabolic rate was lower than in extant great apes and much under the values observed in extant Homo sapiens [8]. For instance, between 4.4-million-year-old pre-Australopith Ardipithecus and Homo sapiens, brain volume increased 4.7-fold, but flow rate in the internal carotid arteries increased 9.3-fold, indicating an approximate doubling of metabolic intensity in brain tissue. By contrast, among haplorhine primates, flow rate in the internal carotid arteries is proportional to brain volume suggesting a constant volume-specific brain metabolic rate in these species.
In the course of Hominins evolution, the deviation from allometric relationships between brain size increases and brain blood flow rate indicates a marked increase of metabolic intensity of brain tissue in later forms [8]. Along these lines [9] it was emphasized that in Hominins brain energy metabolism relies not only on the number of neurons in the neocortex but the complexity of their dendritic arborization—the number of spines and synapses—which increases “more than linearly” in the ultimate steps of hominization. In particular, the high density of synaptic contacts on dendritic spines in humans which creates an electrochemical ion-gradient disruption upon activation imposing higher energy demands [10, 11, 12]. Given the existence of a tight metabolic coupling between neurons and glia, astrocytes in particular, the overall astrocyte/neuron ratio increases in humans. This happens together with a massive enhanced expression of genes involved in energy production in the human neocortex [13, 14], particularly those involved in the electron chain transfer of ATP-producing oxidative phosphorylation [15, 16]. All this might be viewed as a new connectomic fundamental, that we may referred to as “brain energetics”, essential in the general process of brain hominization, and which deserves further investigations along these lines.
In Australopiths, the pattern of cortical organization remains primitive and essentially ape-like (Figure 1). In particular one does not observe a reduction of the visual primary cortex and an expansion of the adjacent parietal areas, once believed to be already present in Australopiths [17]. However, also notable is the prolonged period of brain development relative to chimpanzees. This change in the life history pattern is likely to be related to the energetics requirements of a larger brain in a less productive environments. It provided a basis for the later evolution on a protracted human-like growth pattern critical to an extended brain plasticity and the establishment of a long period of childhood learning through synapse selection already considered as a major connectomic fundamental [4].
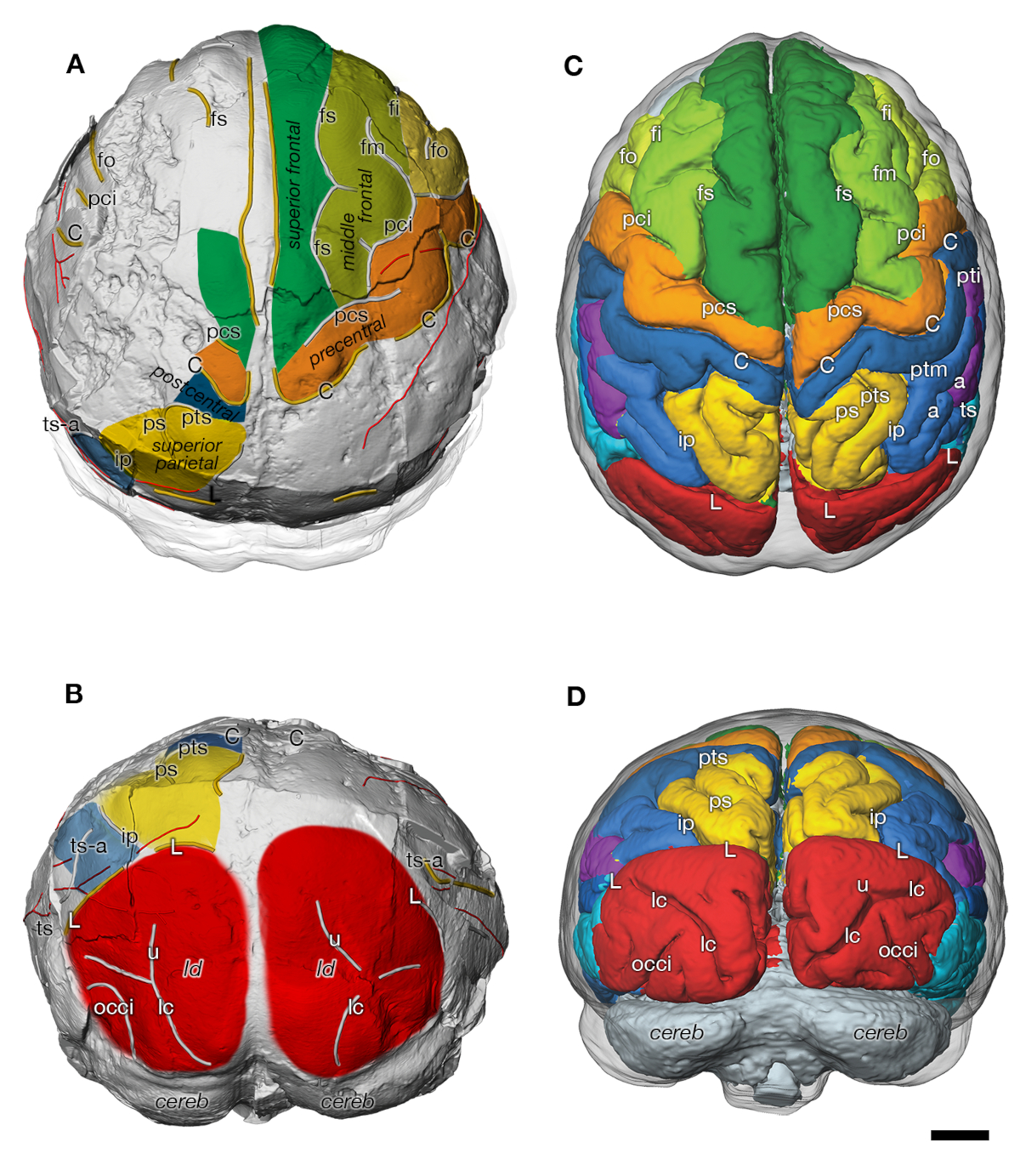
Virtual endocast of DIK-1-1 Australopithecus afarensis (A: superior view: B: posterior view) and endocranial surface of an immature chimpanzee (C: superior view: D: posterior view) (from [17]). Both species display a similar organization of sulci and gyri. C, sulcus centralis; fs, frontalis superior; fm, frontalis medius; fi, frontalis inferior; fo, fronto-orbitalis; h, horizontal ramus of pci; ip, s. intraparietalis; pci, praecentralis inferior; pcs, praecentralis superior; ps, parietalis superior; pti, postcentralis inferior; ptm, postcentralis medius; pts, postcentralis superior; L, s. lunatus; ts, temporalis superior; ts-a, ramus temporalis superior; tm, temporalis medius; occi, occipitalis inferior; lc, s. calcarinus lateralis; u, s. calcarinus ramus superior; cereb, cerebellum; ld, lambdoidal suture. Scale bar is 1 cm. Masquer
Virtual endocast of DIK-1-1 Australopithecus afarensis (A: superior view: B: posterior view) and endocranial surface of an immature chimpanzee (C: superior view: D: posterior view) (from [17]). Both species display a similar organization of sulci and gyri. C, sulcus ... Lire la suite
From the point of view of behaviour and social organization, one cannot find extant models for Australopiths in present-days large Primates. It is important however to underline, that the strong canine reduction and the decrease of dental sexual dimorphism documented among Hominins since their emergence, are almost fully achieved in Australopiths. These features are consistent with a reduction of interindividual aggressivity (especially between males) and reproductive behaviours as well as social organisations departing from those observed among living apes. In other words, Australopiths would already show cooperative relationships reminiscent of later human societies as manifested by the first evidence of the prolonged period of childhood epigenetic imprinting and social learning unique to humans.
3. Early Homo
Several species of early Homo have been identified in the Early Pleistocene fossil record of Africa: Homo habilis, Homo rudolfensis and early Homo erectus (“Homo ergaster”). They widely overlap chronologically and represent distinct lineages sharing a common ancestor but displaying their own evolutionary trajectories. Among them, only Homo erectus survived after 1.4 million years ago and is most generally considered ancestral to later Hominins. Before this date, absolute brain sizes are not much different between the different species of early Homo and range between 510 and 850 ml, with individuals of the three taxa plotting in the lower values of this range [18]. In term of cortical organization, early forms of the genus Homo continue to retain a primitive pattern, in particular with a great ape–like organization of the frontal lobe. The cerebellum also remains relatively small compared to that of recent Homo sapiens.
4. Homo erectus
The oldest representatives of Homo erectus sensu lato are dated circa 1.9 million years before present in east and south Africa. This date also corresponds to major behavioural changes observed in the archaeological record, in particular regarding the increase of predation on mid- to large size mammals, access to aquatic food and related dietary habits. 100,000 years later one also finds the first undisputable evidence for the presence of the genus Homo outside of Africa and the development of new stone technologies including, in Africa, the production of large cutting tools such as Acheulean bifaces.
The Homo erectus fossil record covers a very long span of time, from 1.9 million years before present in Africa to maybe as late as 125,000 years ago in Java, and a huge geographical range over all Africa and the southern and middle latitudes of Eurasia. Understandably, the phenotype of the species displays large variations, probably still underestimated. Regarding brain size, the largest values are reached by terminal Indonesian specimens (1250 ml in Solo 5), in strong contrast with the oldest forms of the species (546 ml in Dmanisi 4500). Derived conditions of the cortical organization seem to develop between 1.7 and 1.5 million years ago [19], later than the first dispersals of Homo from Africa. They include the differential enlargement and reorganisation of the frontal lobe specially the inferior prefrontal cortex and Brodmann area (BA) 45/47, in tandem with the posterior parietal cortex, the temporal lobe and to some extent the occipital lobe (Figure 2). Together with BA44, BA45 make up Broca’s area, known to be involved in language processing in Homo sapiens. Furthermore, in today’s humans patients lesions of BA45 lead to expressive aphasia and BA47 is implicated in the processing of syntax in oral and sign languages, musical syntax, and semantic aspects of language [20] together with the facial expression of emotions [21]. Such frontal lobe evolutions might have constituted the foundations of the “language-ready” brain [19] of later Homo species [22]. It might also represent some intermediate—still unknown—form of communication between chimp grunts, calls or signs and properly human language.
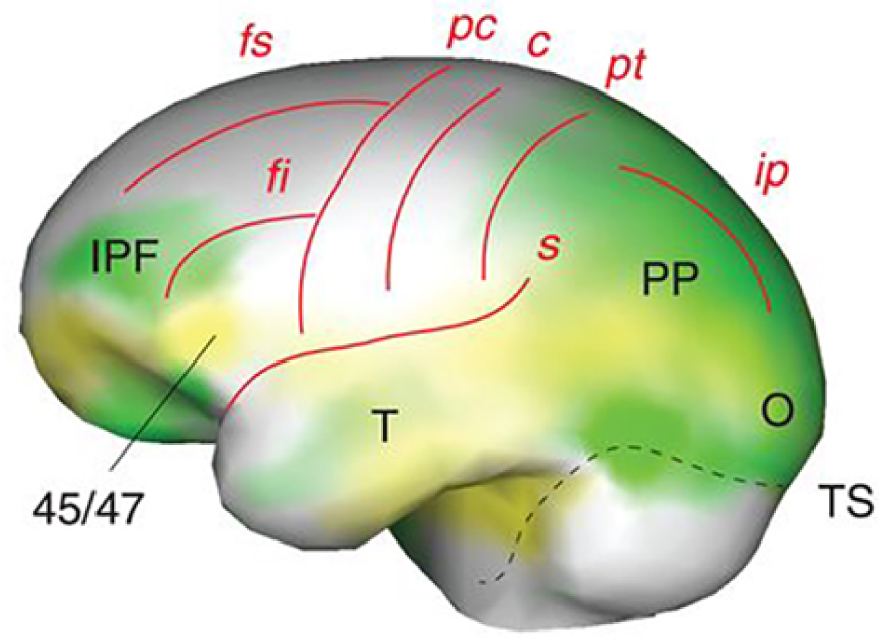
Left lateral view of endocranial morphology of early Homo (from [19]). Expanding areas are in green, bulging in yellow (IPF, inferior prefrontal cortex; 45/47, Brodmann areas 45/47; PP, posterior parietal cortex; T, temporal lobe, O, occipital lobe, TS, transverse sinus). Brain sulci are in red: fs/fi, superior/inferior frontal; pc/c/pt, precentral/central/postcentral; s, Sylvian; ip, intraparietal.
Life history of Homo erectus is another aspect of their evolution than have been much debated. For the oldest forms (>1.5 million years old), dental microstructures suggest a timing of development still reminiscent from that of extant apes and faster than that of recent humans [23]. Adulthood might have been reached by 12 years of age [24]. Evidence from neurocranial individual development also indicates a proportional volumetric growth of the brain at best intermediate between that of apes and that of Homo sapiens [4, 25, 26]. Neural maturation was therefore likely faster in Homo erectus than in later Hominins, with some implications on the length of the period of brain plasticity during growth. Later weaning period followed by faster somatic development and sexual maturation also have implications on the lesser degree of human-like cooperative breeding that early Homo erectus might have displayed.
It has been suggested that the variation in early Lower Paleolithic stone artifacts produced by Homo erectus may “reflect a heavier reliance on behaviors that reside within the zone of latent solutions than on behaviours that make use of high-fidelity social learning” [27]. This would imply that genuine cumulative and transmissible culture might have been put fully established in the course of human evolution only with the late representatives of Homo erectus.
5. Large brained hominins
During the Middle Pleistocene, several lineages evolved large brains close in size to present-days means or even above. They include the latest representatives of Homo erectus in Indonesia (Ngandong series), but they are primarily represented by a group sharing a common ancestor circa 650,000 years ago and include Homo sapiens, Neandertals and Denisovans. Brain volume increase occurred independently and in parallel in these lineages, most likely in relation with similar pressures of selection generated by the emergence of more complex behaviours.
Changes in the endocranial organisation through time are best documented in Neandertals and in Homo sapiens. Both lineages display a broadening of the frontal lobes, although it is more marked in the latter group. However, Neandertals (and likely Denisovans) essentially evolved larger brains along the same allometric trajectory than more primitive representatives of the genus Homo such as late Homo erectus [28]. In contrast, in the course of the last 300,000 years, Homo sapiens brain size have remained essentially constant [28, 29], but display a significant reorganisation in shape (Figure 3) and likely connectivity [28]. These changes are rather fast and affect in a concomitant manner altogether different parts of the brain and have resulted in a more globular shape of the brain in recent forms of Homo sapiens. The bulging of the parietal areas is one of the most visible changes in the endocranial shape, but does seem to be related to an increase of the parietal cortex surface. This bulging might instead be possibly related to the development of some internal structures (pre-cuneus and putamen have been discussed as possible candidates). Changes also affect the temporal lobes [30]. However, most spectacular is the reorganization of the cerebellum with an increase in size of some of its parts.
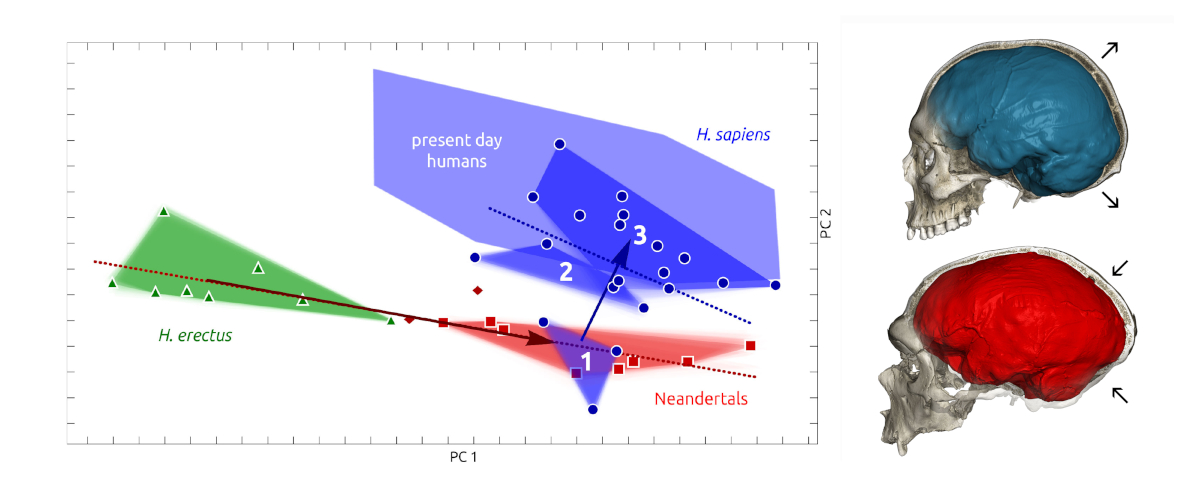
Left: comparative form analysis (PCA) incorporating a size factor (along PC1) of the endocranial shape of H. erectus, H. neanderthalensis and H. sapiens (from [28]). Successive chronological groups of H. sapiens (numbered 1–3) are represented by purple polygons. Neandertals are represented in red and H. erectus in green. Right: comparison of the endocranial shape of the La Chapelle-aux-Saints Neandertal (in red) with that of a recent H. sapiens (in blue).
Paleogenetic and genetic data further reveal that Neanderthal alleles on chromosomes 1 and 18 are associated with reduced endocranial globularity. These alleles influence expression of two nearby genes, UBR4 and PHLPP1, which are involved in neurogenesis and myelination of the cerebellum, respectively [31]. The analysis of transcriptomic data in the region of human genome that have been cleared by selection of Neandertal introgressions (“Neandertal genomic deserts”) indicate that structures outside de neocortex such as cerebellum, striatum and the mediodorsal nucleus of the thalamus have been under a strong positive selection [32]. Also suggesting a strong positive selection, a series of non-synonymous mutations affecting coding genes are absent in Neandertals and Denisovans but present in virtually all extant humans [2]. To date few of these changes have been deciphered, but several are involved in brain development and connectivity. The modern variants of KIF18a and KNL1 are proven to cause metaphase prolongation and fewer chromosome segregation errors during cell divisions in apical progenitors of developing neocortex [33]. The modern version of TKTL1 results in an increase in cortical neuron production and increases the ability of the basal radial glia to self-amplify [34]. Although rather fast, at the geological scale, these evolutionary changes seem to be gradual through time and it is only between 100,000 and 50,000 BP that endocranial shapes of fossil Homo sapiens start entering the present-day range of variation [28].
A significant inverse relationship of globularity and grey matter was noted in large parts of the frontal, temporal, and occipital gyri; parts of the cerebellum; and several subcortical regions (thalamus, putamen, hippocampus) [31] to the unmentioned benefit of the white matter (see also [35]). A plausible connectomic backbone which would integrate—“link together”—these widely distributed territories of the brain (and make the brain “more globular” or compact) might be hypothesized as an enhanced development of the long-range horizontal connectivity of the brain [4]. This high-order core network has been postulated to contribute to the global neuronal workspace (GNW) [36, 37] and suggested to be mobilized by conscious processing, higher cognitive functions and global integration [37, 38]. Interestingly the cerebellum—which was not mentioned in the original version of the GNW—might according to the above mentioned paleontological data [31] be viewed as one of its basic components (see also [39] in the case of the genetic predispositions to autism). This possibility was evoked quite a while ago [40] but still remains to be further demonstrated.
It is highly significant that the globular brain shape differenciating recent adult Homo sapiens from other Hominins results from the appearance of a new phase of development taking place during the first year of post-natal development [41]. During this critical period, the dendritic arborizations of cortical pyramidal neurons and the long-range connectivity actively develop together for up to 15 years and even later [42]. The development of the baby brain progresses as a multistep nested foliation resulting from successive waves of synapse outgrowth, stabilization, and elimination processes, which formally resemble an evolutionary “Darwinian” process [43, 44, 45, 46, 47]. In developing monolingual children, one-word utterances are produced between 12 and 18 months, and vocabulary development “explodes” at 18 months of age [48], then sentences develop from 2–3 years until complete sentences of 4–5 words at age 4. On the other hand, neither monkeys nor chimpanzees ever learn to combine words into a multiword “utterance” [49, 50]. The ability to process hierarchically structured sequences resulting in a new higher-order element been assigned to a subpart of Broca’s area, BA44 and a fronto-temporal language network connecting in the left hemisphere language-relevant regions via dorsally located white matter fiber tracts [4, 50]. Intriguingly, around 2 years, characteristic changes in the postnatal maturation of pyramidal projection neurons which originate from upper layers—specifically layer IIIc—in the human prefrontal cortex take place [4, 51, 52, 53]. These neurons exhibit a unique differential increase and epigenetic pruning in the number of segments and length of their basal dendrites between 16 months and 2.5 years especially in the prefrontal cortex (ref in [4, 51]). They are a major integrative element of cortical processing and might possibly contribute to the more globular shape of Homo sapiens brain. One may speculate that advanced theory of mind and high order language processing depend on the fine tuning of these long-range connections and thus possibly contribute to the observed difference with archaic hominin brains.
Brain asymmetry that underlies behavioral and anatomical hemispheric specialization for complex cognitive abilities such as speech production is commonly interpreted as “unique” to the human brain. Examination of endocranial shape in humans, chimpanzees, gorillas, and orangutans, respectively all reveal a mean deviation from symmetry. These findings also indicate a decreased correlation and thus higher variability of frontal, occipital, and cerebellar asymmetries in humans as compared to nonhuman apes [54]. This might possibly result from the differential postnatal out growth and synaptic epigenesis of some cortical territories typically illustrated with Broca’s area and language learning.
From a behavioral point of view, the second the Middle Pleistocene witnessed numerous changes. Although pre-existing, the use of fire by Hominins become gradually more common [55] and is well documented in the archaeological record after 400,000 BP. Gathering around a common fire may have enhanced oral communication and its diversification within members of the social groups. In Acheulean assemblages produced by groups ancestral to Neandertals and early Homo sapiens, the occurrence of highly symmetrical bifaces indicates the development of an esthetic sense and the high investment in some artifacts production beyond the functional needs. Late Middle and Upper Pleistocene Hominins were capable of advanced technical skills and, to variable extents, of “symbolic” comportments. However, the frequency of “non-utilitarian” objects in the archeological record of African Homo sapiens after 140,000 BP [56] remains, to date, unparalleled in other groups of large brained hominins of the same time period. After the last expansion of terminal (“modern”) Homo sapiens outside of Africa and south east Asia starting 50,000 years ago and the replacement of all other forms of Hominins, another major step was taken with the massive production of figurative “art” representing animals, humans and imaginary creatures [57]. This peculiar cultural trajectory proper to our species may have contributed to the selection of late features of human brain connectivity, eventually referred to as belonging to a “cultural brain” [4].
6. Late small brained Hominins
The increase in encephalization level is a major trend in hominin evolution since the Australopiths, with an acceleration in the course of the last million years. This increase has impacted many other aspects of Hominin adaptations ranging from life history to metabolic rate, and from technological development to social networking. However, in the past two decades, at least two lineages of fossil hominins escaping to this general pattern have been identified. One is represented by Homo floresiensis (from Flores Island, Indonesia), a minute creature with a stature circa 1.0 m and an endocranial capacity of 425 ml [58]. Homo floresiensis survived in isolation until the arrival of modern Homo sapiens of African origin in the region, 50,000 years ago [59]. It is generally interpreted as an example of insular dwarfism. The second example is represented by Homo naledi, discovered in two nearby caves in the vicinity of Johannesburg, South Africa. Its geological age is estimated around 300,000 years. Homo naledi was somewhat (ca. 1.45 m) taller than H. floresiensis, but, considering its age, it also displays a remarkably small cranial capacity ranging between 460–560 ml [60].
The presence of derived frontal lobe morphologies in both species, and the mosaic of skeletal features they display suggest that they possibly evolved from some earlier Homo erectus local populations. Alternatively, if the emphasis is put on some of their primitive features, these derived conditions may result from homoplasy. The cranial capacities of the two forms lay in the range of Australopiths and early Homo, indicating at best a stagnation (H. naledi) or even a regression (H. floresiensis) of their level of encephalization. Such trends might result from limitations in the capabilities of these species to extract high amounts of energy from their environments. Although this is a rather common situation in insular environments, this pattern is more puzzling in the case of Homo naledi. The latter species did not evolve in the complete geographical isolation of an island and must have been exposed to the competition of large brained primitive Homo sapiens documented in the southern part of the African continent. A peculiar ecological niche and/or dietary adaption [61] likely explain the oddity of this Hominin, which, so far, has not been found in direct association to lithic artefacts or any kind.
7. Conclusions
If most speculatively one can tentatively index the evolution of the human brain upon plausible neural signatures of language evolution one may distinguish there main “moments” within a highly complex ramification of many lineages. Australopith are characterized by brain sizes in average 30% bigger than the means measured among common chimpanzees with a pattern of cortical organization which remains primitive and essentially ape-like. Also notable is the first evidence for a prolonged period of brain development relative to chimpanzees anticipating the postnatal period of childhood learning unique to humans. Australopiths would also show early signs of cooperative relationships proper to human society. In other words, the connectomic fundamentals related to brain size increase and extended postnatal development look already at work.
During the following 2 My, the brain size increases dramatically, partly in relation to changes in the body format. This increase includes the differential enlargement and reorganization of the frontal lobe specially the inferior prefrontal cortex and Broca language areas BA 45/47, in tandem with the posterior parietal cortex, the temporal lobe and to some extent the occipital lobe. They produce the foundations of the “language-ready” brain of late H. erectus and following Homo species which developed a rich social life and a diversity of techno-cultures. The connectomic fundamental concerned by the increase of brain size and especially the increase of neurons numbers and cortical areas seem to dominate the picture.
In recent forms of Homo sapiens, there is a modest increase in brain size, however with a significant reorganisation resulting in a more globular shape of the brain affecting concomitantly altogether the frontal and temporal lobes, the parietal areas and the cerebellum. A plausible connectomic backbone might “link together” these widely distributed territories of the brain possibly due to an enhanced development of the long range—horizontal—connectivity of the brain. In Homo sapiens, the postnatal development of the baby brain maturation extends considerably up to 15 years and in particular between 1.5 and 2.5 years the dendritic arborization of layer IIIc pyramidal cells in the prefrontal cortex blossoms as an important connectomic fundamental plausibly related to the development of high order language processing and higher learning functions.
The genetic events related to the evolution of these basic connectomic fundamentals have been already discussed [4] yet without the precise timing and species attributions provided by the paleontological approach. The evidence that Neanderthal alleles on chromosomes 1 and 18 are associated with reduced endocranial globularity deserves further consideration. Indeed, it is claimed that these alleles modulate the expression of two nearby genes, UBR4 and PHLPP1. UBR4 encodes a ubiquitin ligase that regulates neurogenesis in the developing neocortex and, among other roles, promotes neuronal migration and PHLPP1 encodes a negative regulator of the PI3K/Akt growth-factor signaling pathway that drives myelination (14). Such “single structural genes” approach may indeed reveal the contribution of protein-coding genes in the hominization process. Yet, as discussed (4), such contribution may actually be part of a wider regulation of larger patterns of genes under the control of a small number of genetic regulatory events. Accordingly, by analogy with genetic predispositions of ASD (39), “gene networks would encode neuronal networks” that determine behavioral phenotypes. This perspective advocates the heritability of complex traits of the connectome (and of many diseases) is spread broadly across the genome, which implies that a substantial fraction of all genes contributes to it (4).
Sill much remains to be explored to the benefit of this joint neurobiological-paleontological approach. In any case, the evolutionary trajectory followed by Homo sapiens over the last 300,000 years contrasts sharply with that followed by other large-brained Pleistocene Hominins. In our species, the improvement in cognitive abilities did not ultimately result from the continuous volumetric increase observed previously. Its evolutionary divergence represented a truly novel adaptive response to the ever-increasing metabolic costs of a larger brain.
Conflicts of interest
Authors have no conflict of interest to declare.
Acknowledgments
This project has received funding from the European Union’s Horizon 2020 Framework Programme for Research and Innovation under Specific Grant Agreement No. 945539 (Human Brain Project SGA3).
The Max Planck Society has funded J-JH’s researches.
Version française
1. Remarques introductives
L’évolution des Hominidés n’est pas un processus linéaire mais résulte de la ramification complexe de nombreuses lignées qui ont divergé, coexisté et même parfois partiellement fusionné. Il est donc difficile de définir des étapes bien délimitées, car, à presque n’importe quelle période du passé, plusieurs formes d’Hominines coexistaient sur terre, généralement dans différentes parties du globe, mais parfois dans les mêmes zones continentales. Chacune de ces lignées présentait sa propre mosaïque de caractéristiques, de l’anatomie au régime alimentaire et du cycle de vie à l’organisation sociale. Lorsqu’on analyse l’évolution de ces lignées, il est évident qu’elles présentent des changements significatifs au fil du temps. Par exemple, l’organisation endocrânienne des formes les plus anciennes d’Homo sapiens est plus proche de celle des Néandertaliens que de celle des formes tardives (dites « modernes ») d’Homo sapiens [1]. En outre, lorsqu’on analyse les processus d’évolution des Hominines, l’homoplasie (évolution convergente sans origines communes proches ou retour à des conditions primitives) est un problème majeur qu’il ne faut pas négliger. On s’attend à ce que ces changements proviennent de variations au niveau du génome qui peuvent être examinées à partir de séquences entières d’ADN fossiles dans des formes récentes telles que les Néandertaliens [2] et les Denisovans [3] et/ou par interpolation entre les grands singes actuels et les Humains modernes. Bien que les relations déterministes des événements génétiques précis qui définissent les circuits cérébraux échappent encore largement à notre compréhension, des tentatives préliminaires ont été faites pour relier plus étroitement les variations du génome à l’évolution d’entités intégrées plus larges que nous avons appelées « fondamentaux connectomiques » globaux [4] tels que la taille du cerveau, la connectivité à longue distance ou la synaptogenèse postnatale prolongée. Au-delà du génome, les propriétés intrinsèques des tissus en développement, les interactions entre régions anatomiques adjacentes et plus largement les phénomènes d’intégration ont contribué également à la neuroanatomie des différentes espèces d’Hominines [5]. Enfin, d’un point de vue paléontologique, on peut essentiellement traiter de la taille, de la forme, des modèles de développement et des artefacts culturels, mais la connectivité cérébrale et le langage sont des questions notoirement difficiles à aborder. En conséquence, la présente tentative de relier plus étroitement la paléoanthropologie et l’évolution du cerveau — même si elle est intéressante — restera en partie spéculative.
2. Australopithèques
Les Australopithèques représentent un groupe paraphylétique documenté depuis 4,2 millions d’années et à partir duquel le genre Homo est apparu il y a environ 2,8 millions d’années [6]. Les dernières avancées sur l’évolution du cerveau des Australopithèques montrent que ce groupe d’espèces est caractérisé par des tailles de cerveau en moyenne 30% plus grandes que les moyennes mesurées chez les chimpanzés communs [7]. Cependant, l’anatomie des foramens basicrânien montre que le débit sanguin cérébral était plus faible que celui des grands singes actuels et, par conséquent, que le taux métabolique cérébral était inférieur et bien en dessous des valeurs observées chez l’Homo sapiens [8]. Par exemple, entre Ardipithecus, un pré-Australopithèque vieux de 4,4 millions d’années, et Homo sapiens, le volume du cerveau a été multiplié par 4,7, mais le débit des artères carotides internes a été multiplié par 9,3, ce qui indique un doublement approximatif de l’intensité métabolique du tissu cérébral. En revanche, chez les primates haplorhiniens, le débit des artères carotides internes est proportionnel au volume du cerveau, ce qui suggère un taux métabolique cérébral constant et propre au volume chez ces espèces.
Au cours de l’évolution des Hominines, la déviation des relations allométriques entre l’augmentation de la taille du cerveau et le débit sanguin cérébral indique une augmentation marquée de l’intensité métabolique du tissu cérébral dans les formes tardives [8]. Dans le même ordre d’idées [9], il a été souligné que chez les Hominines, le métabolisme énergétique du cerveau dépend non seulement du nombre de neurones dans le néocortex mais aussi de la complexité de leur arborisation dendritique — le nombre d’épines et de synapses — qui augmente « plus que linéairement » dans les étapes ultimes de l’hominisation. En particulier, la forte densité de contacts synaptiques sur les épines dendritiques chez l’homme crée une perturbation du gradient ionique électrochimique lors de l’activation, ce qui impose des demandes énergétiques plus élevées [10, 11, 12]. Étant donné l’existence d’un couplage métabolique étroit entre les neurones et la glie, les astrocytes en particulier, le rapport global astrocytes/neurones augmente chez l’Homme. Cela se produit en même temps qu’une augmentation massive de l’expression des gènes impliqués dans la production d’énergie dans le néocortex humain [13, 14], en particulier ceux impliqués dans le transfert de la chaîne d’électrons de la phosphorylation oxydative productrice d’ATP [15, 16]. Tout ceci pourrait être considéré comme un nouveau fondamental connectomique, que nous pourrions appeler « énergétique cérébrale », essentiel dans le processus général d’hominisation cérébrale, et qui mérite des investigations supplémentaires dans ce sens.
Chez les Australopithèques, le modèle d’organisation corticale reste primitif et essentiellement comparable à celui des grands singes (Figure 1). En particulier, on n’observe pas de réduction du cortex visuel primaire et d’expansion des zones pariétales adjacentes, que l’on a crues un temps déjà présent chez les Australopithèques [17]. Cependant, il faut souligner la période prolongée de développement du cerveau par rapport aux chimpanzés. Ce changement du modèle d’histoire de vie est probablement lié aux besoins énergétiques d’un cerveau plus grand dans un environnement moins productif. Il a servi de base à l’évolution ultérieure d’un modèle de croissance prolongée de type humain, essentiel pour la mise en place d’une plasticité cérébrale étendue et à l’établissement d’une longue période d’apprentissage pendant l’enfance par la sélection de synapses, déjà considérée comme un élément connectomique fondamental [4].
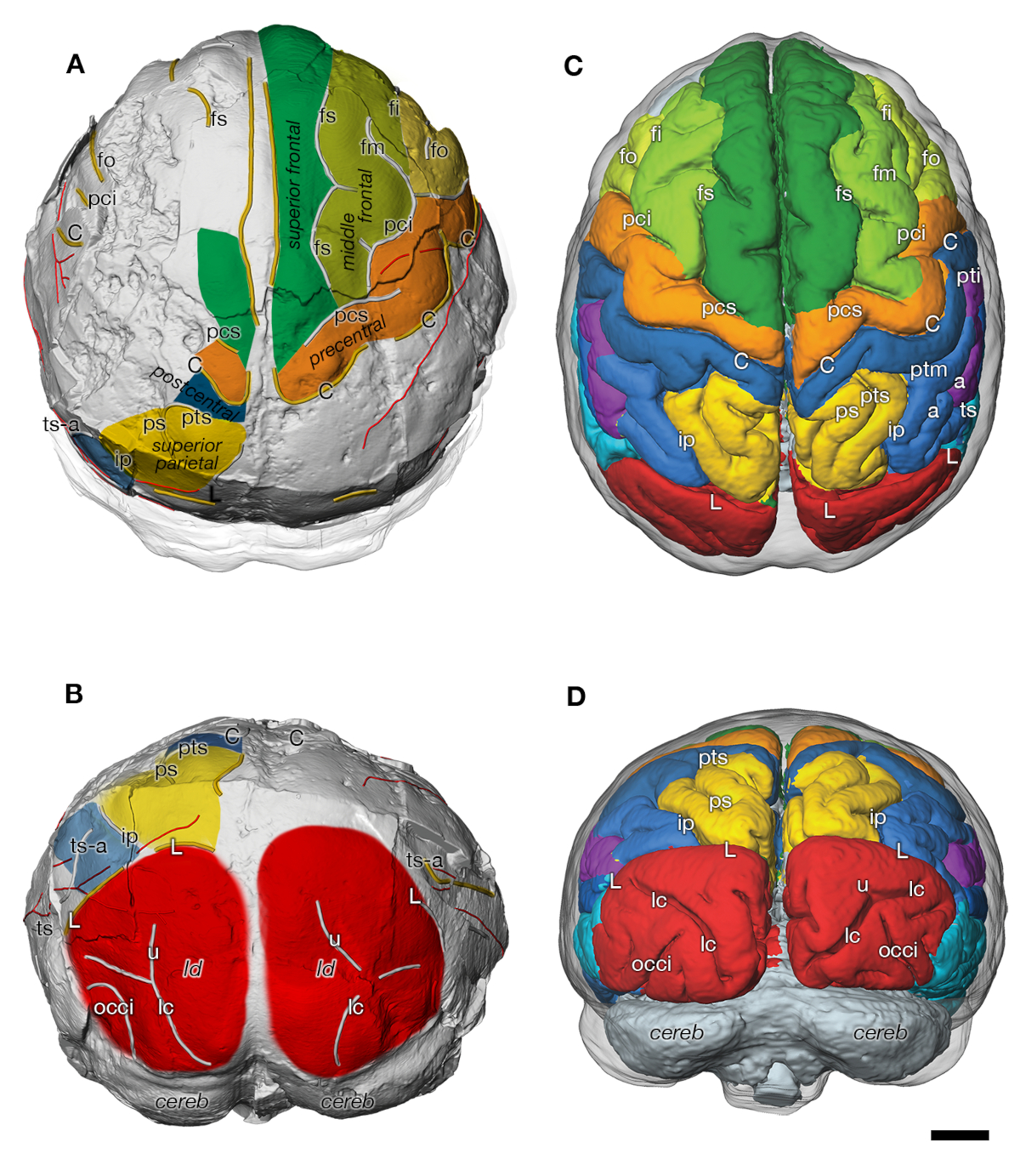
Endocrâne virtuel de l’Australopithecus afarensis DIK-1-1 (A : vue supérieure : B : vue postérieure) et surface endocrânienne d’un chimpanzé immature (C : vue supérieure : D : vue postérieure) (d’après [17]). Les deux espèces présentent une organisation similaire des sulci et des gyri. C, sulcus centralis ; fs, frontalis superior ; fm, frontalis medius ; fi, frontalis inferior ; fo, fronto-orbitalis ; h, ramus horizontal du pci ; ip, s. intraparietalis ; pci, praecentralis inferior ; pcs, praecentralis superior ; ps, parietalis superior ; pti, postcentralis inferior ; ptm, postcentralis medius ; pts, postcentralis superior ; L, s. lunatus ; ts, temporalis superior ; ts-a, ramus temporalis superior ; tm, temporalis medius ; occi, occipitalis inferior ; lc, s. calcarinus lateralis ; u, s. calcarinus ramus superior ; cereb, cerebellum ; ld, suture lambdoïde. La barre d’échelle est de 1 cm. Masquer
Endocrâne virtuel de l’Australopithecus afarensis DIK-1-1 (A : vue supérieure : B : vue postérieure) et surface endocrânienne d’un chimpanzé immature (C : vue supérieure : D : vue postérieure) (d’après [17]). Les deux espèces présentent une organisation similaire ... Lire la suite
Du point de vue du comportement et de l’organisation sociale, on ne peut pas trouver chez les grands primates actuels de modèles existants pour les Australopithèques. Il est cependant important de souligner que la forte réduction des canines et la diminution du dimorphisme sexuel dentaire documentées chez les Hominines depuis leur émergence, sont presque entièrement réalisées chez les Australopithèques. Ces caractéristiques indiquent une réduction de l’agressivité interindividuelle (surtout entre les mâles) et des comportements reproductifs ainsi que des organisations sociales qui s’écartent de celles observées chez les grands singes vivants. En d’autres termes, comme le montrent les premiers indices de la période prolongée de l’empreinte épigénétique infantile et de l’apprentissage social propres aux humains, les Australopithèques présenteraient probablement déjà des relations de coopération accrues qui seront ensuite la marque des sociétés humaines.
3. Premiers Homo
Plusieurs espèces primitives du genre Homo ont été identifiées au sein des fossiles du Pléistocène inférieur d’Afrique : Homo habilis, Homo rudolfensis et Homo erectus anciens (« Homo ergaster »). Elles se chevauchent largement chronologiquement et représentent des lignées distinctes partageant un ancêtre commun mais affichant leurs propres trajectoires évolutives. Parmi elles, seul Homo erectus a survécu au-delà de 1,4 million d’années avant le présent et est le plus généralement considéré comme l’ancêtre des Hominines plus tardifs. Avant cette date, la taille absolue du cerveau n’est pas très différente entre les différentes espèces d’Homo primitifs et se situe entre 510 et 850 ml, avec des individus des trois taxons se situant dans les valeurs inférieures de cette fourchette [18]. En termes d’organisation corticale, les formes précoces du genre Homo conservent un schéma primitif, avec notamment une organisation du lobe frontal semblable à celle des grands singes. Le cervelet reste également relativement petit par rapport à celui des Homo sapiens récents.
4. Homo erectus
Les plus anciens représentants d’Homo erectus sensu lato sont datés d’environ 1,9 million d’années avant le présent, en Afrique de l’Est et du Sud. Cette date correspond également à des changements comportementaux majeurs observés dans les archives archéologiques, notamment en ce qui concerne l’augmentation de la prédation sur les mammifères de taille moyenne à grande, l’accès à la nourriture aquatique et les habitudes alimentaires correspondantes. 100 000 années plus tard, on trouve également les premières preuves indiscutables de la présence du genre Homo en dehors d’Afrique et le développement de nouvelles technologies de la pierre, notamment, en Afrique, la production de grands outils coupants tels que les bifaces acheuléens.
Les fossiles d’Homo erectus couvrent une très longue période de temps, de 1,9 million d’années avant le présent en Afrique à peut-être 125 000 ans à Java, et une vaste étendue géographique couvrant toute l’Afrique et les latitudes méridionales et moyennes de l’Eurasie. Naturellement, le phénotype de l’espèce présente de grandes variations, probablement encore sous-estimées. En ce qui concerne la taille du cerveau, les plus grandes valeurs sont atteintes par les spécimens indonésiens terminaux (1250 ml chez Solo 5), en fort contraste avec les formes les plus anciennes de l’espèce (546 ml chez Dmanisi 4500). Des conditions dérivées de l’organisation corticale semblent se développer entre 1,7 et 1,5 millions d’années [19], plus tard donc que les premières dispersions d’Homo hors d’Afrique. Elles comprennent l’élargissement différentiel et la réorganisation du lobe frontal, en particulier le cortex préfrontal inférieur et l’aire de Brodmann (BA) 45/47, en tandem avec le cortex pariétal postérieur, le lobe temporal et, dans une certaine mesure, le lobe occipital (Figure 2). Avec la zone BA44, la BA45 constitue l’aire de Broca, connue pour être impliquée dans le traitement du langage chez l’Homo sapiens. De plus, chez l’homme actuel, les lésions de la BA45 entraînent une aphasie d’expression et la BA47 est impliquée dans le traitement de la syntaxe des langues orales et des signes, de la syntaxe musicale et des aspects sémantiques du langage [20] ainsi que dans l’expression faciale des émotions [21]. Ces évolutions du lobe frontal pourraient avoir constitué les fondations du cerveau « prêt pour le langage » [19] des espèces du genre Homo ultérieures [22]. Elles pourraient également représenter une forme intermédiaire — encore inconnue — de communication entre les grognements les appels ou les signes des chimpanzés et le langage proprement humain.
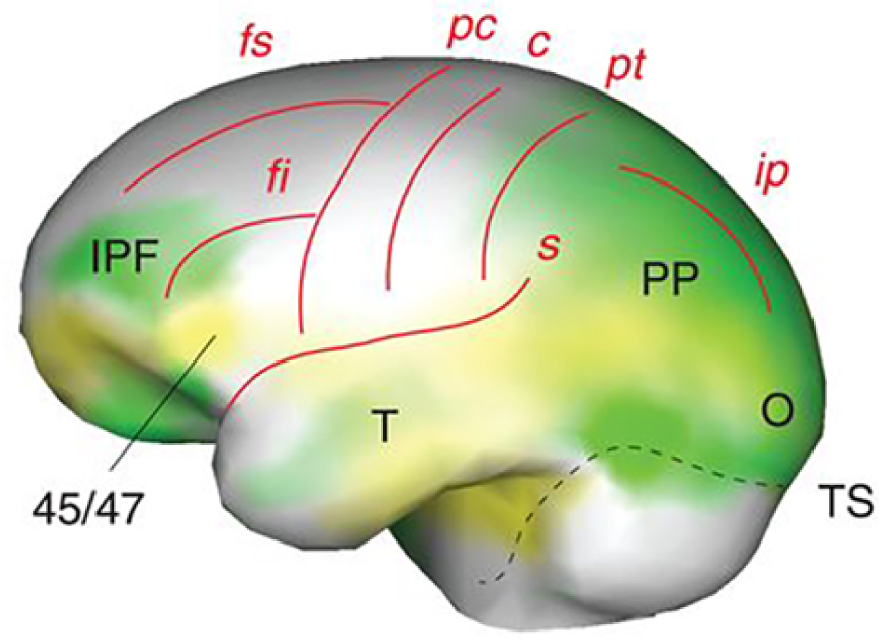
Vue latérale gauche de la morphologie endocrânienne des Homo anciens (d’après [19]). Les zones en expansion sont en vert, les zones en saillie en jaune (IPF, cortex préfrontal inférieur ; 45/47, zones de Brodmann 45/47 ; PP, cortex pariétal postérieur ; T, lobe temporal, O, lobe occipital, TS, sinus transverse). Les sillons cérébraux sont en rouge : fs/fi, frontal supérieur/inférieur ; pc/c/pt, précentral/central/postcentral ; s, sylvien ; ip, intrapariétal.
L’histoire de la vie des Homo erectus est un autre aspect de leur évolution qui a fait l’objet de nombreux débats. Pour les formes les plus anciennes (>1,5 million d’années), les microstructures dentaires suggèrent une période de développement qui rappelle encore celle des grands singes actuels et qui est plus rapide que celle des humains récents [23]. L’âge adulte aurait été atteint vers 12 ans [24]. Les données sur le développement neurocrânien individuel indiquent également une croissance volumétrique proportionnelle du cerveau, au mieux intermédiaire entre celle des grands singes et celle d’Homo sapiens [4, 25, 26]. La maturation neuronale était donc probablement plus rapide chez Homo erectus que chez les Hominines ultérieurs, ce qui a quelques implications sur la durée de la période de plasticité cérébrale pendant la croissance. Une période de sevrage plus tardive suivie d’un développement somatique et d’une maturation sexuelle plus rapides ont également des implications sur le moindre degré de reproduction coopérative de type humain que l’Homo erectus précoce aurait pu présenter.
Il a été suggéré que la variation des artefacts du Paléolithique inférieur ancien produits par l’Homoerectus pourrait « refléter un plus grand recours aux comportements qui résident dans la zone des solutions latentes que dans les comportements qui font appel à un apprentissage social de haute fidélité » [27]. Cela impliquerait qu’une véritable culture cumulative et transmissible n’a pu être pleinement établie au cours de l’évolution humaine qu’avec les derniers représentants de l’Homo erectus.
5. Les Hominines à gros cerveau
Au cours du Pléistocène moyen, plusieurs lignées ont évolué vers des cerveaux de grande taille, proches des moyennes actuelles, voire supérieures. Elles comprennent les derniers représentants de l’Homo erectus en Indonésie (série de Ngandong), mais elles sont surtout représentées par un groupe partageant un ancêtre commun il y a environ 650 000 ans et qui comprend l’Homo sapiens, les Néandertaliens et les Dénisoviens. L’augmentation du volume cérébral s’est produite indépendamment et en parallèle dans ces lignées, très probablement en relation avec des pressions de sélection similaires générées par l’émergence de comportements plus complexes.
C’est chez les Néandertaliens et chez Homo sapiens que les changements dans l’organisation endocrânienne au fil du temps sont le mieux documentés. Les deux lignées présentent un élargissement des lobes frontaux, qui se trouve plus marqué chez le dernier groupe. Cependant, les Néandertaliens (et probablement les Dénisoviens) ont essentiellement évolué vers des cerveaux plus gros, suivant la même trajectoire allométrique, que les représentants plus primitifs du genre Homo, comme les Homo erectus tardifs [28]. En revanche, au cours des 300 000 dernières années, la taille du cerveau de l’Homo sapiens est restée essentiellement constante [28, 29], mais a connu une réorganisation significative de sa forme (Figure 3) et probablement de sa connectivité [28]. Ces changements sont assez rapides et affectent de manière concomitante des parties totalement différentes du cerveau, ce qui a abouti à une forme plus globulaire du cerveau chez les formes récentes d’Homo sapiens. Le renflement des aires pariétales est l’un des changements les plus visibles de la forme endocrânienne, mais il ne semble pas être lié à une augmentation de la surface du cortex pariétal. Ce renflement pourrait plutôt être lié au développement de certaines structures internes (le précuneus et le putamen ont été évoqués comme candidats possibles). Les changements affectent également les lobes temporaux [30]. Cependant, le plus spectaculaire est la réorganisation du cervelet avec une augmentation de la taille de certaines de ses parties.
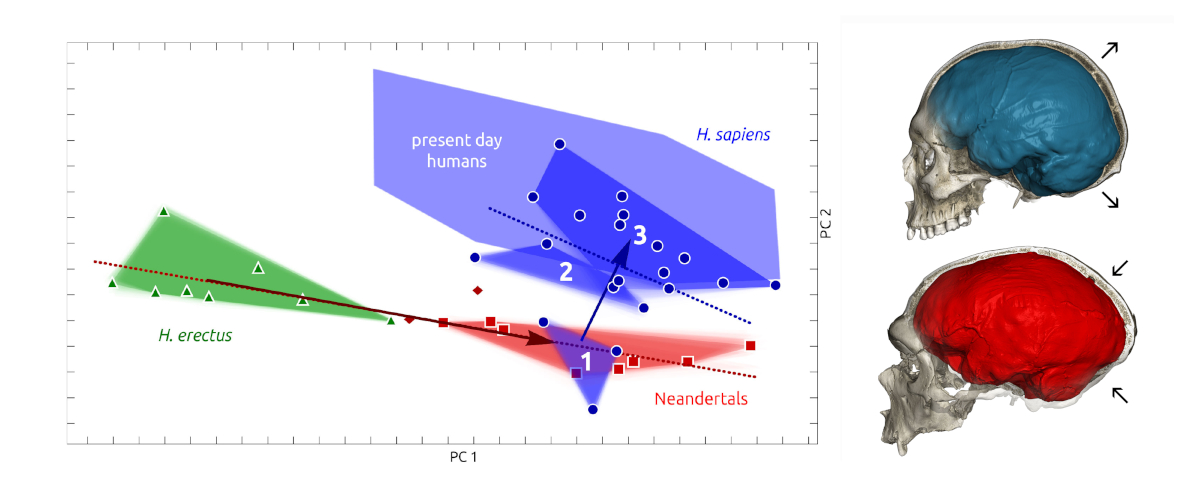
A gauche : analyse comparative des formes (ACP) intégrant un facteur de taille (selon PC1) de la forme endocrânienne d’H. erectus, H. neanderthalensis et H. sapiens (d’après [28]). Les groupes chronologiques successifs d’ H. sapiens (numérotés de 1 à 3) sont représentés par des polygones violets. Les Néandertaliens sont représentés en rouge et H. erectus en vert. A droite : comparaison de la forme endocrânienne du Néandertalien de La Chapelle-aux-Saints (en rouge) avec celle d’un H. sapiens récent (en bleu). Masquer
A gauche : analyse comparative des formes (ACP) intégrant un facteur de taille (selon PC1) de la forme endocrânienne d’H. erectus, H. neanderthalensis et H. sapiens (d’après [28]). Les groupes chronologiques successifs d’ H. sapiens (numérotés ... Lire la suite
Les données paléogénétiques et génétiques révèlent en outre que des allèles néandertaliens sur les chromosomes 1 et 18 sont associés à une globularité endocrânienne réduite. Ces allèles influencent l’expression de deux gènes proches, UBR4 et PHLPP1, qui sont impliqués respectivement dans la neurogenèse et la myélinisation du cervelet [31]. L’analyse des données transcriptomiques dans les régions du génome humain qui ont été purgées par la sélection d’introgressions néandertaliennes (« déserts génomiques néandertaliens ») indique que des structures en dehors du néocortex telles que le cervelet, le striatum et le noyau médio-dorsal du thalamus ont subi une forte sélection positive [32]. Suggérant également une forte sélection positive, une série de mutations non synonymes affectant des gènes codants sont absentes chez les Néandertaliens et les Dénisoviens mais présentes chez pratiquement tous les humains actuels [2]. A ce jour, peu de ces mutations ont été décryptées, mais plusieurs d’entre elles sont impliquées dans le développement et la mise en place de la connectivité du cerveau. Il a été démontré que les variantes modernes de KIF18a et KNL1 provoquent une prolongation de la métaphase et moins d’erreurs de ségrégation chromosomique lors des divisions cellulaires dans les progéniteurs apicaux du néocortex en développement [33]. La version moderne de TKTL1 entraîne elle une augmentation de la production de neurones corticaux et accroît la capacité de la glie radiaire basale à s’auto-amplifier [34]. Bien qu’assez rapides, à l’échelle géologique, ces changements évolutifs semblent être graduels dans le temps et ce n’est qu’entre 100 000 et 50 000 ans avant le présent que les formes endocrâniennes des Homo sapiens fossiles commencent à entrer dans la gamme de variations actuelles [28].
Une relation inverse significative entre la globularité et la matière grise a été notée dans de grandes parties des gyri frontaux, temporaux et occipitaux, dans certaines parties du cervelet et dans plusieurs régions sous-corticales (thalamus, putamen, hippocampe) [31], au profit de la substance blanche (voir également [35]). Une colonne vertébrale connectomique plausible qui intégrerait — « relierait » — ces territoires largement distribués du cerveau (et rendrait le cerveau « plus globulaire » ou compact) pourrait résulter d’un développement accru de la connectivité horizontale — à longue distance — du cerveau [4]. Il a été postulé que ce réseau central de haut niveau contribue à l’espace de travail neuronal global (ETNG) [36, 37] et qu’il est mobilisé par le traitement conscient, les fonctions cognitives supérieures et l’intégration globale [37, 38]. Il est intéressant de noter que le cervelet, qui n’était pas mentionné dans la version originale du ETNG, pourrait, selon les données paléontologiques susmentionnées [31], être considéré comme l’un de ses composants de base (voir également [39] dans le cas des prédispositions génétiques à l’autisme). Cette possibilité a été évoquée il y a un certain temps [40] mais reste encore à démontrer.
Il est très significatif que la forme globulaire du cerveau qui différencie l’Homo sapiens adulte récent des autres hominidés résulte de l’apparition d’une nouvelle phase de développement au cours de la première année du développement post-natal [41]. Au cours de cette période critique, les arborisations dendritiques des neurones pyramidaux corticaux et la connectivité à longue portée se développent activement jusqu’à 15 ans, voire plus tard [42]. Le développement du cerveau du bébé progresse sous la forme d’une foliation emboîtée à plusieurs étapes résultant de vagues successives de processus de croissance, de stabilisation et d’élimination des synapses, qui ressemblent formellement à un processus évolutif « darwinien » [43, 44, 45, 46, 47]. Chez les enfants monolingues en développement, des énoncés d’un seul mot sont produits entre 12 et 18 mois, et le développement du vocabulaire « explose » à 18 mois [48], puis les phrases se développent à partir de 2–3 ans jusqu’à des phrases complètes de 4–5 mots à 4 ans. En revanche, ni les petits singes ni les chimpanzés n’apprennent jamais à combiner des mots pour former une « énonciation » de plusieurs mots [49, 50]. La capacité de traiter des séquences hiérarchiquement structurées aboutissant à un nouvel élément d’ordre supérieur a été attribuée à une sous-partie de l’aire de Broca, BA44, et à un réseau linguistique fronto-temporal reliant, dans l’hémisphère gauche, les régions pertinentes pour le langage par des trajets de fibres de la substance blanche situés dorsalement [4, 50]. Il est intéressant de constater que vers l’âge de 2 ans, des changements caractéristiques se produisent dans la maturation postnatale des neurones de projection pyramidaux qui proviennent des couches supérieures — en particulier la couche IIIc — du cortex préfrontal humain [4, 51, 52, 53]. Ces neurones présentent une augmentation différentielle unique et un élagage épigénétique du nombre de segments et de la longueur de leurs dendrites basales entre 16 mois et 2,5 ans, en particulier dans le cortex préfrontal (réf. dans [4, 51]). Ils constituent un élément intégrateur majeur du traitement cortical et pourraient éventuellement contribuer à la forme plus globulaire du cerveau de l’Homo sapiens. On peut supposer que la « théorie de l’esprit » (theory of mind) et le traitement du langage de haut niveau dépendent du réglage fin de ces connexions à longue distance et qu’ils contribuent ainsi à la différence observée avec le cerveau des Hominines archaïques.
L’asymétrie cérébrale qui sous-tend la spécialisation hémisphérique comportementale et anatomique pour les capacités cognitives complexes telles que la production de la parole est généralement interprétée comme étant « unique » au cerveau humain. L’examen de la forme endocrânienne chez l’homme, le chimpanzé, le gorille et l’orang-outan, respectivement, révèle une déviation moyenne de la symétrie. Ces résultats indiquent également une corrélation moindre et donc une plus grande variabilité des asymétries frontales, occipitales et cérébelleuses chez les humains par rapport aux grands primates non humains [54]. Cela pourrait éventuellement résulter de la croissance postnatale différentielle et de l’épigenèse synaptique de certains territoires corticaux typiquement illustrés par l’aire de Broca et l’apprentissage du langage.
D’un point de vue comportemental, le deuxième Pléistocène moyen a connu de nombreux changements. Bien que préexistante, l’utilisation du feu par les Hominines est devenue progressivement plus courante [55] et est bien documentée dans les archives archéologiques après 400 000 ans. Le fait de se rassembler autour d’un feu commun a pu favoriser la communication orale et sa diversification entre les membres des groupes sociaux. Dans les assemblages acheuléens produits par des groupes ancestraux des Néandertaliens et des premiers Homo sapiens, la présence de bifaces hautement symétriques indique le développement d’un sens esthétique et l’investissement élevé dans la production de certains artefacts au-delà des besoins fonctionnels. Les Hominidés de la fin du Pléistocène moyen et du Pléistocène supérieur étaient capables de compétences techniques avancées et, à des degrés variables, de comportements « symboliques ». Cependant, la fréquence des objets « non-utilitaires » dans les archives archéologiques d’Homo sapiens africains après 140 000 BP [56] reste, à ce jour, sans équivalent dans les autres groupes d’Hominidés à gros cerveau de la même période. Après la dernière expansion de l’Homo sapiens terminal (« moderne ») en dehors de l’Afrique et de l’Asie du Sud-Est, il y a 50 000 ans, et le remplacement de toutes les autres formes d’Hominines, une autre étape majeure a été franchie avec la production massive d’ « art » figuratif représentant des animaux, des humains et des créatures imaginaires [57]. Cette trajectoire culturelle particulière propre à notre espèce a pu contribuer à la sélection de caractéristiques tardives de la connectivité du cerveau humain, que l’on a désigné sous le terme « cerveau culturel » [4].
6. Hominidés tardifs à petit cerveau
L’augmentation du niveau d’encéphalisation est une tendance majeure de l’évolution des Hominines depuis les Australopithèques, avec une accélération au cours du dernier million d’années. Cette augmentation a eu un impact sur de nombreux autres aspects de l’adaptation des Hominines, allant du mode de vie aux taux métaboliques, et du développement technologique aux réseaux sociaux. Cependant, au cours des deux dernières décennies, au moins deux lignées d’Hominidés fossiles échappant à ce schéma général ont été identifiées. La première est représentée par Homo floresiensis (de l’île de Flores, en Indonésie), une créature minuscule d’une stature d’environ 1,0 m et d’une capacité endocrânienne de 425 ml [58]. L’Homo floresiensis a survécu en isolement jusqu’à l’arrivée de l’Homo sapiens moderne d’origine africaine dans la région, il y a 50 000 ans [59]. Il est généralement interprété comme un exemple de nanisme insulaire. Le deuxième exemple est représenté par Homo naledi, découvert dans deux grottes voisines des environs de Johannesburg, en Afrique du Sud. Son âge géologique est estimé à environ 300 000 ans. Homo naledi était un peu plus grand (environ 1,45 m) que H. floresiensis, mais, compte tenu de son âge, il présentait également une capacité crânienne remarquablement faible, comprise entre 460 et 560 ml [60].
La présence de morphologies dérivées du lobe frontal chez les deux espèces et la mosaïque de caractéristiques squelettiques qu’elles présentent suggèrent qu’elles ont peut-être évolué à partir de populations locales d’Homo erectus plus anciennes. Cependant, si l’on met l’accent sur certaines de leurs caractéristiques primitives, ces conditions dérivées pourraient aussi résulter d’une homoplasie. Les capacités crâniennes des deux formes se situent dans la gamme des Australopithèques et des premiers Homo, indiquant au mieux une stagnation (H. naledi) ou même une régression (H. floresiensis) de leur niveau d’encéphalisation. De telles tendances pourraient résulter de limitations dans les capacités de ces espèces à extraire de grandes quantités d’énergie de leur environnement. Bien qu’il s’agisse d’une situation assez courante dans les environnements insulaires, ce schéma est plus déroutant dans le cas d’Homo naledi. Cette dernière espèce n’a pas évolué dans l’isolement géographique complet d’une île et a dû être exposée à la concurrence des Homo sapiens primitifs à gros cerveau documentés dans la partie sud du continent africain. Une niche écologique et/ou une adaptation alimentaire particulière [61] expliquent probablement la singularité de cet Hominine qui, jusqu’à présent, n’a pas été trouvé en association directe avec des artefacts lithiques d’aucune sorte.
7. Conclusions
Si l’on peut, de manière très spéculative, indexer l’évolution du cerveau humain sur des signatures neurales plausibles de l’évolution du langage, on peut distinguer des « moments » principaux dans une ramification très complexe de nombreuses lignées. Les Australopithèques se caractérisent par des tailles de cerveau en moyenne 30% plus grandes que les moyennes mesurées chez les chimpanzés communs avec un modèle d’organisation corticale qui reste primitif et essentiellement similaire à celui des grands singes. Il faut également noter les premiers signes d’une période prolongée de développement du cerveau par rapport aux chimpanzés, annonçant la période postnatale d’apprentissage de l’enfance propre aux humains. Les Australopithèques montreraient également les premiers signes de relations coopératives propres aux sociétés humaines. En d’autres termes, les principes fondamentaux de la connectomique liés à l’augmentation de la taille du cerveau et au développement postnatal prolongé semblent déjà à l’œuvre.
Au cours des deux millions d’années suivants, la taille du cerveau augmente de façon spectaculaire, en partie en relation avec des changements du format corporel. Cette augmentation comprend l’élargissement et la réorganisation différentielle du lobe frontal, en particulier le cortex préfrontal inférieur et les aires du langage de Broca BA 45/47, en tandem avec le cortex pariétal postérieur, le lobe temporal et, dans une certaine mesure, le lobe occipital. Ils constituent les fondements du cerveau « prêt pour le langage » de l’Homo erectus tardif et des espèces suivantes qui ont développé une vie sociale riche et une diversité de techno-cultures. Les fondements connectomiques concernés par l’augmentation de la taille du cerveau et surtout l’augmentation du nombre de neurones et des zones corticales semblent dominer le tableau.
Chez les formes récentes de l’Homo sapiens, il y a une augmentation modeste de la taille du cerveau, mais avec une réorganisation significative qui aboutit à une forme plus globulaire, affectant de manière concomitante l’ensemble des lobes frontaux et temporaux, les aires pariétales et le cervelet. Une colonne vertébrale connectomique plausible pourrait « relier » ces territoires largement distribués du cerveau, peut-être en raison d’un développement accru de la connectivité horizontale à longue distance du cerveau. Chez Homo sapiens, le développement postnatal et la maturation du cerveau de l’enfant s’étendent considérablement jusqu’à 15 ans et, en particulier, entre 1,5 et 2,5 ans, l’arborisation dendritique des cellules pyramidales de la couche IIIc dans le cortex préfrontal s’épanouit comme une importante base connectomique plausiblement liée au développement du traitement du langage de haut niveau et des fonctions d’apprentissage supérieures.
Les événements génétiques liés à l’évolution de ces bases connectomiques ont déjà été discutés [4], mais sans la chronologie précise et les attributions d’espèces fournies par l’approche paléontologique. La preuve que les allèles néandertaliens sur les chromosomes 1 et 18 sont associés à une globularité endocrânienne réduite mérite d’être approfondie. En effet, il est affirmé que ces allèles modulent l’expression de deux gènes voisins, UBR4 et PHLPP1. UBR4 code une ubiquitine ligase qui régule la neurogenèse dans le néocortex en développement et, entre autres rôles, favorise la migration neuronale, et PHLPP1 code un régulateur négatif de la voie de signalisation du facteur de croissance PI3K/Akt qui dirige la myélinisation (14). Cette approche « gènes de structure uniques » peut en effet révéler la contribution des gènes codant pour les protéines dans le processus d’hominisation. Cependant, comme nous l’avons discuté (4), cette contribution peut, en fait, faire partie d’une régulation plus large d’ensembles de gènes sous le contrôle d’un petit nombre d’événements régulateurs génétiques. En conséquence, par analogie avec les prédispositions génétiques des TSA (39), « les réseaux de gènes coderaient les réseaux neuronaux » qui détermineraient les phénotypes comportementaux. Selon cette perspective, l’héritabilité des traits complexes du connectome (et de nombreuses maladies) serait largement répartie sur le génome, ce qui impliquerait qu’une fraction substantielle de tous les gènes y contribue (4).
Il reste encore beaucoup à explorer pour tirer profit de cette approche conjointe génétique-neurobiologique-paléontologique. En tout état de cause, la trajectoire évolutive suivie par Homo sapiens au cours des 300 000 dernières années contraste fortement avec celle suivie par les autres hominidés pléistocènes à gros cerveau. Chez notre espèce, l’amélioration des capacités cognitives n’a finalement pas simplement résulté de l’augmentation volumétrique continue observée précédemment. Sa divergence évolutive a représenté une réponse adaptative très nouvelle aux coûts métaboliques toujours plus élevés d’un cerveau de plus grande taille.
Conflit d’intérêt
Les auteurs n’ont aucun conflit d’intérêt à déclarer.
Remerciements
Ce projet a reçu un financement du programme-cadre de recherche et d’innovation Horizon 2020 de l’Union européenne dans le cadre de la convention de subvention spécifique n° 945539 (Human Brain Project SGA3).
Les recherches de J-JH ont été financées par le Max Planck Institut.