1 Introduction
Many potential optical and electronic applications have been proposed for conjugated polymers, which have stimulated dynamic research activities on polymers with extended π-conjugations [1,2]. The frontier of conjugated polymer research is moving from linear to dendritic or hyperbranched structures [3−7]. Compared to their dendritic congeners [8,9], hyperbranched polymers enjoy such advantages as ready preparations in large scales by one-pot, single-step reaction procedures [10–12].
Different synthetic strategies have been employed for the preparation of hyperbranched polymers [10–12]. The most commonly adopted approach is self-condensations of ABn–type monomers with n ≥ 2 [13–16]. This type of polymerization can be carried out in a concurrent mode or by slow addition of the monomer or even in the presence of a core molecule of Bf (f ≥ 3). Another approach is copolymerization of A2 monomers with Bn comonomers (n ≥ 3) [17–19]. The stoichiometric requirements between the pairs of the functional comonomers are, however, practically difficult to meet, which often limits the growth of propagating species, resulting in the formation of oligomeric products. If the condensation proceeds in an incomplete or imperfect fashion, polymers with low degrees of branching will be produced.
We are interested in synthesizing hyperbranched polymers by transition metal-catalyzed alkyne polycyclotrimerizations [20,21]. This kind of [2 + 2 + 2] polycycloaddition reaction involves a single monomer species, suffers no stoichiometric constraints, and can thus potentially produce polymers with very high molecular weights. The resultant polymers should be highly branched because the polycyclotrimerization mechanism is intolerant of the formation of linear repeat units inside the hyperbranched cores. In our previous work, we have studied the homopolymerizations of aliphatic α,ω-diynes such as 1,8-nonadiyne and 1,9-decadiyne catalyzed with a binary mixture of TaCl5 and Ph4Sn [22, 23]. Under optimal polymerization conditions, completely soluble diyne homopolymers with high molecular weights (up to ~1.4 × 106) and predominant 1,2,4-benzenetriyl core structures are obtained in high isolation yields (up to 93 wt%) [22–28]. The hyperbranched poly(alkenephenylene)s (HPAPs) show outstanding thermal stability and lose little of their weights at temperatures as high as ~500 °C, although the phenyl rings are separated by the alkyl chains (Fig. 1).
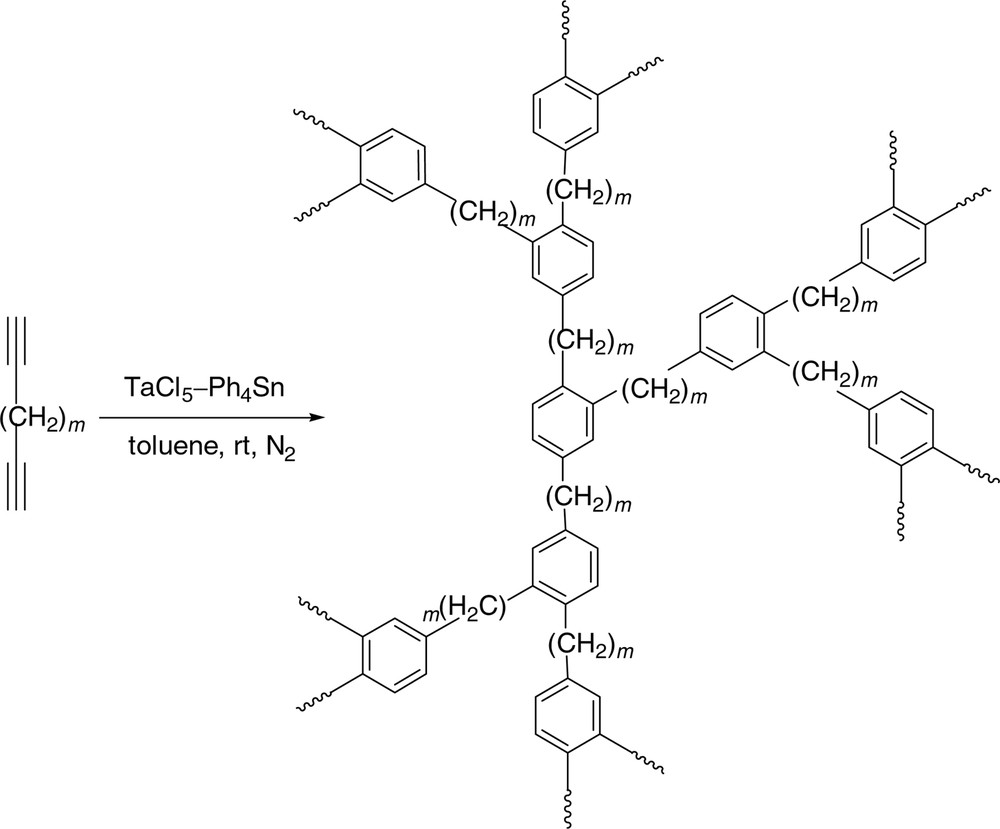
Homopolycyclotrimerizations of aliphatic α,ω-diynes.
In this work, we have extended our research efforts to the polycyclotrimerizations of aromatic diynes. In this account, we will briefly summarize our results on the homopolycyclotrimerizations of organic and organometallic diynes (Fig. 2) and their copolycyclotrimerizations with aromatic and aliphatic monoynes (Fig. 3). Different from the isolated benzene rings in the HPAPs discussed above, here the ‘new’ benzene rings formed by cyclotrimerization and the ‘old’ aromatic moieties from the monomers are interconnected in the hyperbranched polyarylenes (HPAs). This enhances the electronic communications in the HPAs and endows them with efficient light-emitting and excellent optical-limiting properties.
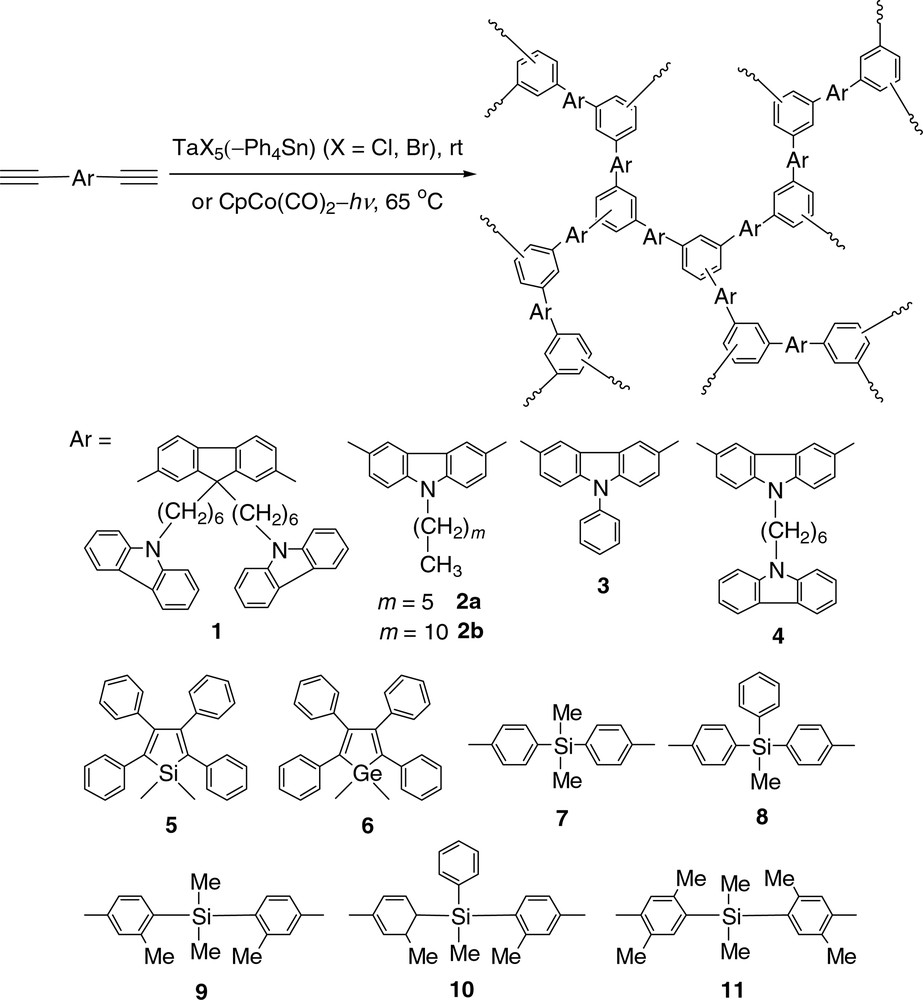
Homopolycyclotrimerizations of aromatic diynes.
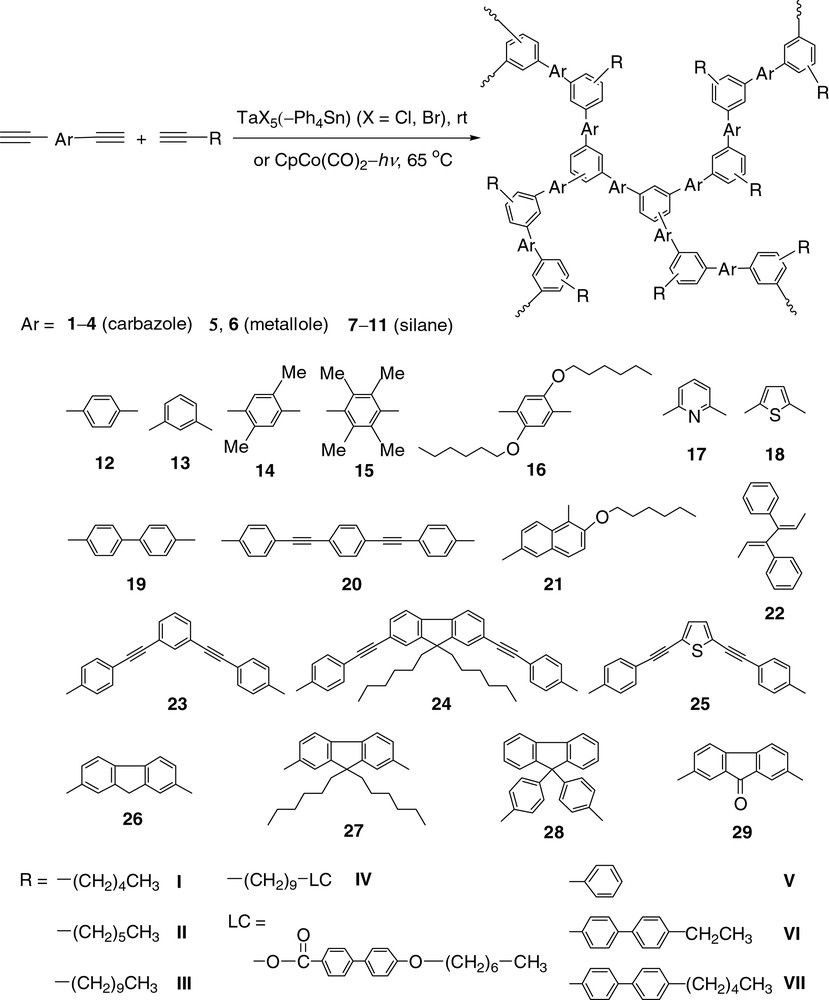
Copolycyclotrimerizations of aromatic diynes with aliphatic and aromatic monoynes.
2 Synthesis
We designed and synthesized a number of organic and organometallic diynes (1–29) and monoynes (I–VII) with varying aromatic rings and functional groups [29–39]. Most of the monomers are prepared in high isolation yields by palladium-catalyzed coupling reactions of silylacetylenes with dihaloarenes followed by base-catalyzed desilylations. We first checked the homopolymerization behaviors of the diynes. As can be seen from Table 1, the diynes containing carbazole functional groups (1–4) can be homopolymerized with the cobalt catalyst under UV irradiation into their corresponding HPAs (P1–P4) in high isolation yields (up to 99.9 wt %). The metallole-containing organometallic diynes (5 and 6) can be polymerized with TaCl5–Ph4Sn at room temperature (Table 1, Nos. 5 and 6). The polymerizations of the silyldiynes are effected by TaBr5, giving HPAs P7–P11 with high molecular weights (Mw up to ~2.1 × 105; noting that the Mw here is calibrated by polystyrene standards and that the real absolute value can be much higher than the underestimated relative value [22]). The homopolymerizations of aromatic diynes 12–29 all proceeded rapidly but the resultant polymeric products are partially or completely insoluble due to the involved crosslinking reactions. The large free volumes and the irregular molecular structures created by the nonlinear carbazolyl, metallolyl, and silyl groups may have conferred the excellent solubility on homo-HPAs P1–P11.
Homopolycyclotrimerizations of aromatic diynesa
No. | HPA | Catalyst | Yield (wt %) | Mwb | Mw /Mnb |
1 | P1 | CpCo(CO)2 − hν | 70.1 | 7800 | 3.8 |
2 | P2a | CpCo(CO)2 − hν | 71.3 | 5000 | 1.8 |
3 | P3 | CpCo(CO)2 − hν | 99.9 | 2700 | 3.9 |
4 | P4 | CpCo(CO)2 − hν | 83.7 | 4000 | 2.9 |
5 | P5 | TaCl5−Ph4 Sn | 83.0 | 5300 | 1.6 |
6 | P6 | TaCl5−Ph4 Sn | 47.0 | 5200 | 1.8 |
7 | P7c | CpCo(CO) 2 − hν | 28.1 | 11 800 | 1.4 |
8 | P7c | TaBr5 | 83.6 | 44 600 | 4.2 |
9 | P8 | TaBr5 | 80.3 | 42 200 | 3.5 |
10 | P9 | TaBr5 | 94.1 | 47 300 | 4.2 |
11 | P10 | TaBr5 | 81.2 | 209 400 | 10.7 |
12 | P11 | TaBr5 | 39.4 | 15 100 | 2.2 |
To suppress the crosslinking reactions and to improve the polymer solubility, we copolymerized the aromatic diynes with monoynes. All the copolycyclotrimerizations underwent smoothly, giving completely soluble co-HPAs of high molecular weights (Mw up to ~1.8 × 105) in high yields (up to 99.7%). 1-Alkynes (I–IV) are generally better comonomers than 1-arylacetylenes (V–VII) when the molecular weights of the HPAs are concerned. For example, when 4,4′-biphenylyldiyne (19) copolymerizes with 1-octyne (II) in the presence of TaCl5–Ph4Sn, HPA P19/II of high molecular weight is produced (Table 2, No. 12). Similarly, a high molecular weight HPA (P19/IV) is obtained from the cobalt-catalyzed copolymerization of 19 with IV, a liquid crystalline 1-alkyne [39]. On the other hand, none of the copolymerizations of 19 (an aromatic diyne) with aromatic monoynes V–VII results in the formation of high molecular weight HPAs (Table 2, Nos. 14–16). This is possibly due to the electronic effects in the copolycyclotrimerization reactions. The electron-donating alkyl groups make the triple bonds of 1-alkynes electronically rich, which are likely to favorably interact with the electron-poor aromatic diynes [40], thus promoting the formation of high molecular weight HPA products.
Copolycyclotrimerizations of diynes with monoynesa
No. | HPA | Catalyst | Yield (wt %) | Mwb | Mw /Mnc |
1 | P1/II | TaBr5−Ph4 Sn | 84.6 | 163 400 | 3.0 |
2 | P2a/II | TaBr5−Ph4 Sn | 88.3 | 13 200 | 3.7 |
3 | P3/II | TaCl5−Ph4 Sn | 84.4 | 28 700 | 7.0 |
4 | P4/II | TaCl5−Ph4 Sn | 96.6 | 6800 | 3.8 |
5 | P5/II | TaCl5−Ph4 Sn | 85.3 | 5800 | 1.7 |
6 | P6/II | TaCl5−Ph4 Sn | 41.0 | 4500 | 8.5 |
7 | P12/III | TaCl5−Ph4 Sn | 76.1 | 67 200 | 9.6 |
8 | P13/I | TaCl5−Ph4 Sn | 94.7 | 97 300 | 3.5 |
9 | P14/I | TaCl5−Ph4 Sn | 86.6 | 37 410 | 3.7 |
10 | P16/V | TaBr5−Ph4 Sn | 75.4 | 21 000 | 3.1 |
11 | P18/II | TaCl5−Ph4 Sn | 65.5 | 33 500 | 8.1 |
12 | P19/II | TaCl5 −Ph4 Sn | 68.9 | 183 100 | 5.6 |
13 | P19/IV | CpCo(CO)2 − hν | 84.9 | 28 600 | 5.8 |
14 | P19/V | CpCo(CO)2 − hν | 73.6 | 4300 | 1.6 |
15 | P19/VI | TaCl5−Ph4 Sn | 31.3 | 7000 | 3.8 |
16 | P19/VII | TaCl5−Ph4 Sn | 72.5 | 9900 | 6.0 |
17 | P20/III | CpCo(CO)2 − hν | 42.0 | 12 200 | 3.7 |
18 | P21/V | TaBr5−Ph4 Sn | 98.1 | 10 500 | 3.1 |
19 | P22/II | TaCl5−Ph4 Sn | 60.0 | 32 700 | 6.7 |
20 | P23/II | TaCl5−Ph4 Sn | 97.0 | 28 600 | 1.7 |
21 | P24/III | CpCo(CO)2 − hν | 97.3 | 27 200 | 3.8 |
22 | P25/II | CpCo(CO)2 − hν | 62.7 | 15 300 | 2.6 |
23 | P26/III | TaCl5−Ph4 Sn | 70.1 | 72 300 | 5.7 |
24 | P27/III | TaCl5−Ph4 Sn | 74.1 | 93 400 | 8.7 |
25 | P28/II | TaBr5−Ph4 Sn | 99.7 | 28 100 | 5.0 |
26 | P29/III | CpCo(CO)2 − hν | 79.6 | 14 100 | 2.7 |
3 Structure
All the HPAs give analytic data satisfactorily corresponding to their expected molecular structures [32,35,41–56]. Fig. 4 shows an example of the IR spectrum of co-HPA P16/V; the data for one of its monomers, i.e., 16, is also given in the same figure for the purpose of comparison. The ≡C−H and C≡C stretching vibrations of 16 occur at 3270 and 2100 cm–1, respectively (Fig. 4A); these peaks are completely disappeared in the spectrum of its polymer (Fig. 4B). The absorption peaks associated with the aromatic =C−H and C=C stretching at 3100 and 1600 cm–1 are, however, clearly intensified, due to the transformation of the triple bonds of 16 to the benzene rings of P16/V.
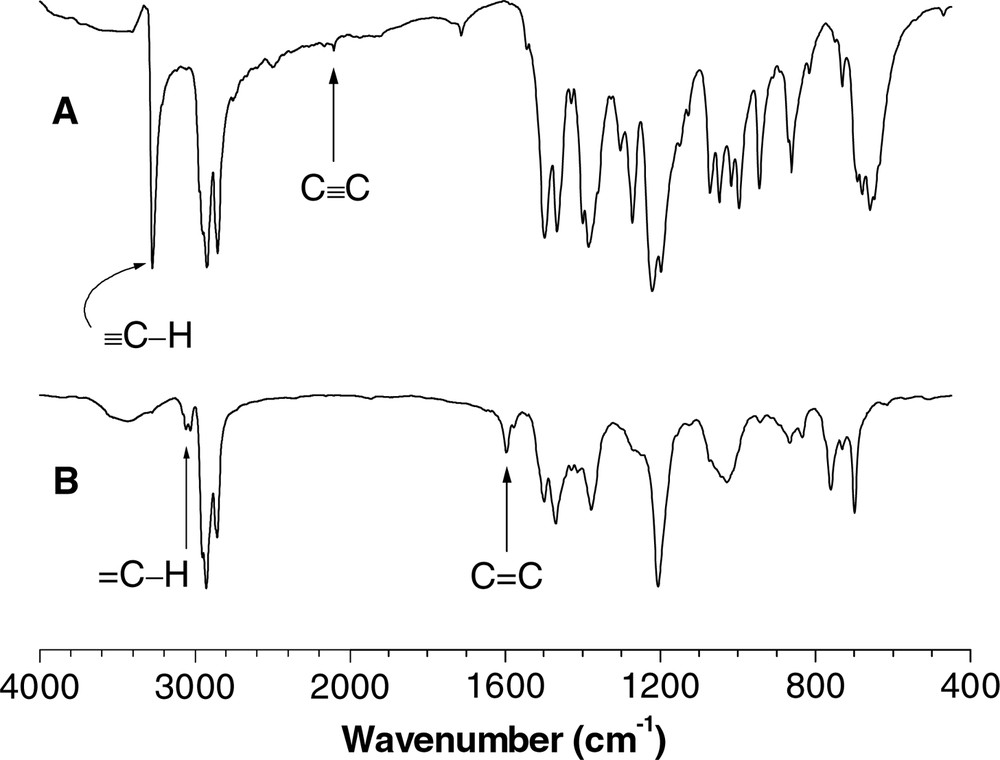
IR spectra of (A) diyne 16 and (B) co-HPA P16/V.
Fig. 5 shows the 1H NMR spectra of diyne 18 and its copolymer P18/II. The absorptions of the acetylenic protons in diyne 18 and monoyne II resonate at δ 3.5 and 1.9, respectively. These peaks are, however, absent in the spectrum of co-HPA P18/II. The polycyclotrimerization converts the triple bonds to aromatic rings, giving a strong aromatic absorption peak at δ 8–6.5 (Fig. 5B). The peak is broad, suggesting that the HPA possesses an irregular stereostructure. Accompanying the polycyclotrimerization, the propargyl protons of monoyne II are transformed to the benzyl ones, whose absorption is observed at δ 2.55. The absorption peaks at δ 2–0.5 are unambiguously due to the resonance of the aliphatic protons of the pentyl group originally from the monoyne II.
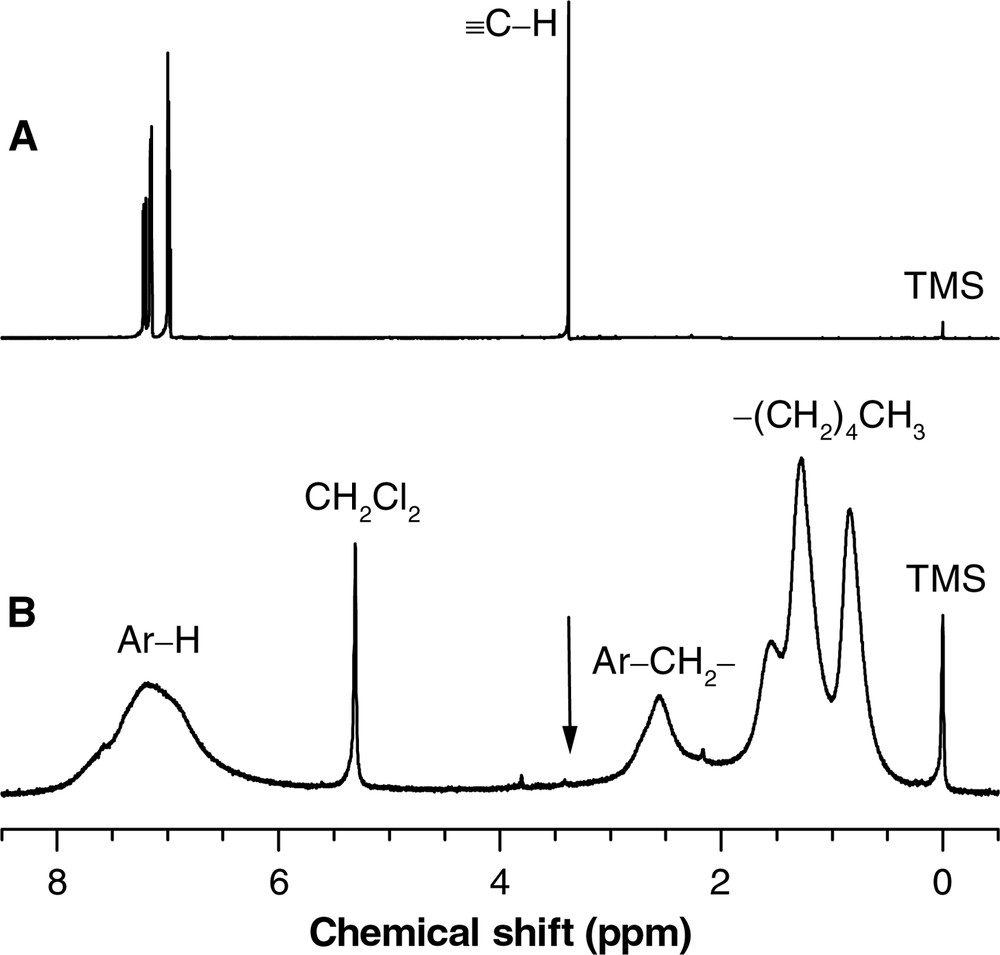
1H NMR spectra of (A) diyne 18 in chloroform-d and (B) HPA P18/II in dichloromethane-d2.
An example of the 13C NMR spectrum of co-HPA P12/V, along with that of one of its monomers (12), is shown in Fig. 6. The acetylenic carbons of 12 absorb at δ 83.7 and 79.8 (Fig. 6A), while those of V resonate at δ 80.7 and 70.5. However, no acetylenic absorption peak is observed in the spectrum of P12/V (Fig. 6B). On the other hand, many peaks are seen in the spectral region of aromatic carbon absorptions. These resonance peaks are the sum of the absorptions of aromatic carbons of the ‘old’ phenyl ring of monomer 12 and the ‘new’ ones of co-HPA P12/V formed by alkyne polycyclotrimerization.
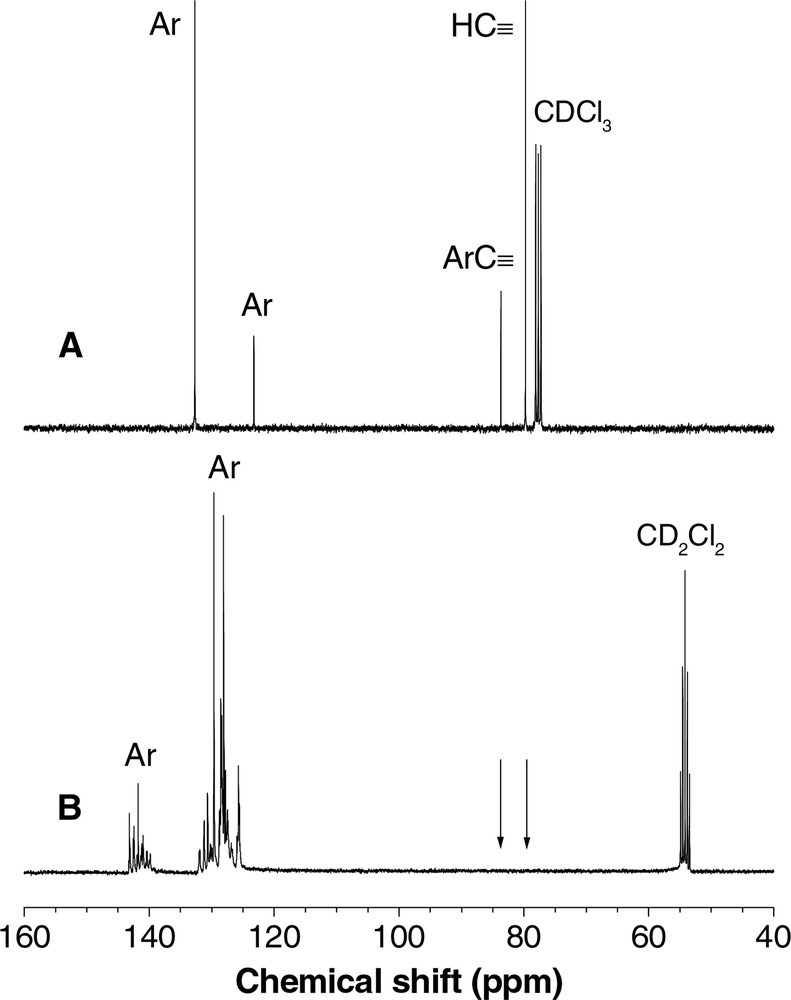
13C NMR spectra of (A) diyne 12 in chloroform-d and (B) HPA P12/V in dichloromethane-d2.
4 Properties
The thermal stabilities of the HPAs are evaluated by thermogravimetric analysis (TGA). Fig. 7 shows a few examples of their TGA thermograms and Table 3 summarizes their thermal analysis data. The HPAs are thermally very stable: for instance, P7–Co loses merely 5% of its weight at a temperature as high as 595 °C. All the polymers, except for P1/II and P18/II, graphitize in > 50% yields upon pyrolysis at 800 °C, with P19/I carbonized in a yield as high as 86 wt % (Table 3, No. 11). The thermal stabilities of the HPAs are similar to that of poly(p-phenylene) (PPP) but different from those of polyacetylenes such as poly(1-hexyne) (PH) and poly(phenylacetylene) (PPA; a linear polymer of monoyne V): PPP is stable up to ~550 °C [3–7], whereas PPA and PH start to decompose at temperatures as low as 220 and 150 °C, respectively [57]. PPP is made of thermally stable aromatic rings, but PPA and PH consist of labile polyene chains. The excellent thermal stabilities of the HPAs verify that they are composed of aromatic rings instead of polyene chains.
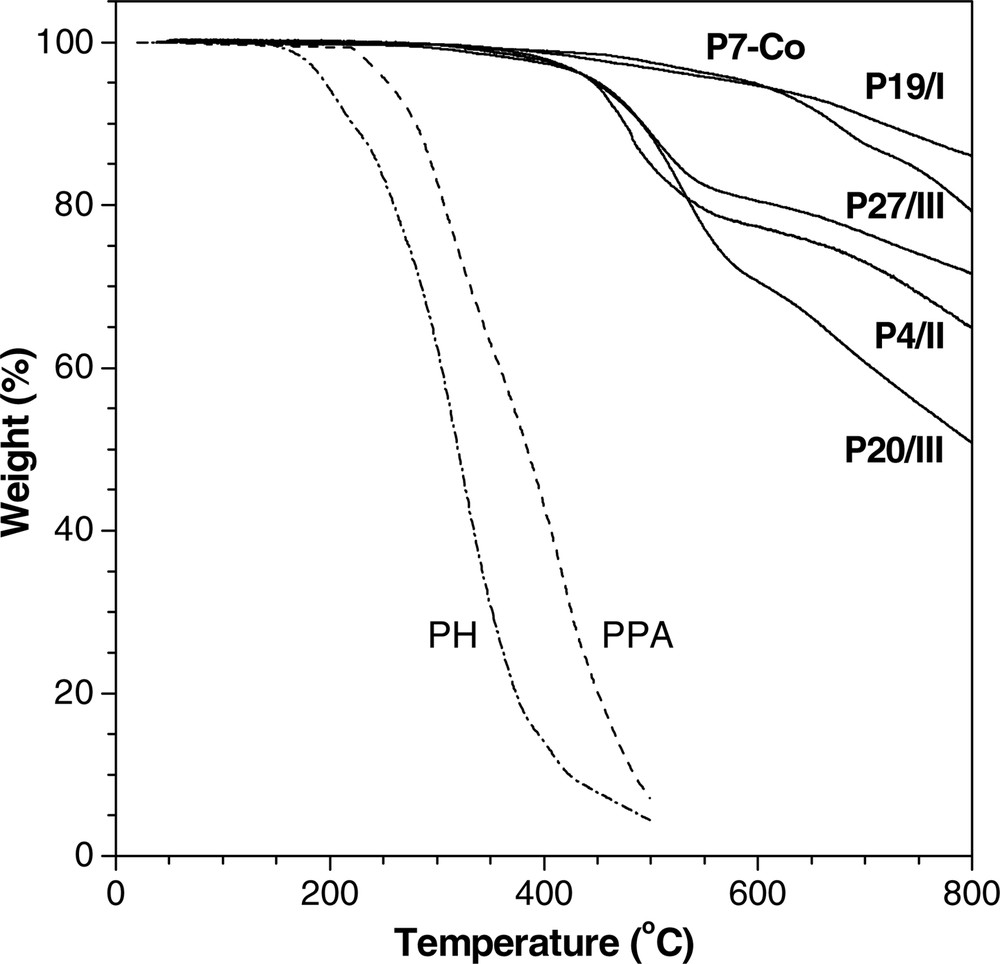
TGA thermograms of hyperbranched polyarylenes; the data for linear poly(1–hexyne) (PH) and poly(phenylacetylene) (PPA) are shown for comparison.
Thermal and optical properties of hyperbranched polyarylenes
No. | HPA | Tda (°C) | Wrb (wt %) | λemc (nm) | ΦFd (%) | T (%) | FLe (mJ/cm2 ) | Ft,m / F i,mf |
1 | P1/II | 414 | 16 | 402 | 90 | 72 | 2300 | 0.63 |
2 | P2a/I | 487 | 58 | 400 | 7 | 84 | 1400 | 0.36 |
3 | P2b/I | 404 | 83 | 398 | 10 | 83 | 635 | 0.36 |
4 | P2b/IV | 474 | 64 | 399 | 20 | 83 | 577 | 0.35 |
5 | P3/II | 477 | 70 | 398 | 21 | 49 | 1034 | 0.19 |
6 | P4/II | 463 | 65 | 396 | 28 | 46 | 1050 | 0.17 |
7 | P7–Ta | 564 | 72 | 397 | 1 | 84 | 2500 | 0.40 |
8 | P7–Co | 595 | 79 | 402 | 1 | 85 | 1500 | 0.43 |
9 | P12/II | 452 | 71g | 400 | 94 | 59 | 1016 | 0.15 |
10 | P18/II | 440 | 0 | 486 | 14 | 48 | 802 | 0.13 |
11 | P19/I | 585 | 86 | 398 | 49 | 58 | 343 | 0.21 |
12 | P19/II | 463 | 66 | 400 | 74 | 69 | 1265 | 0.15 |
13 | P19/IV | 412 | 50 | 397 | 9 | 92 | 900 | 0.52 |
14 | P20/III | 449 | 51 | 399 | 46 | 64 | 260 | 0.11 |
15 | P24/III | 458 | 53 | 399 | 15 | 44 | 155 | 0.08 |
16 | P26/I | 467 | 75 | 400 | 31 | 66 | 126 | 0.11 |
17 | P26/II | 451 | 70 | 400 | 86 | 48 | 1000 | 0.15 |
18 | P26/III | 459 | 65 | 400 | 98 | 65 | 509 | 0.11 |
Careful examination of the TGA data in Table 3 reveals that the co-HPAs with shorter alkyl chains generally have better thermal stabilities. Thus, P19/I, a co-HPA of 4,4′–biphenylyldiyne (19) with 1-heptyne (I; a monoyne with a short alkyl chain), is highly resistant to pyrolysis (Td = 585 °C, Wr = 86%), while its congener P19/II, a co-HPA of 19 with 1-octyne (II; a monoyne with a longer alkyl chain), shows lower Td and Wr (Table 3, Nos. 11 and 12). P19/IV contains even longer alkyl chains and hence shows even lower Td and Wr. Similar general trend is observed in the P26/x series with x = I (1-heptyne), II (1-octyne), and III (1-dodecyne) (Table 3, Nos. 16–18), although all the HPAs are thermally very stable, compared to ‘common’ organic polymers.
The HPAs luminesce when photoexcited, examples of their photoluminescence (PL) spectra being given in Fig. 8. The HPA carrying thienyl chromophores (P18/II) emits a blue-green light of 486 nm, while that with biphenyl ones (P19/I) emits a deep-blue light of 398 nm. More efficient PL is observed in P26/III, a co-HPA containing fluorenyl chromophores. The emission intensities of co-HPAs P19/I and P26/III are obviously higher than that of poly(1-phenyl-1-octyne), a well-known luminescent disubstituted polyacetylene [58,59]. The PL efficiencies of the HPAs change with their molecular structures, with P1/II, P12/II, P19/II, P26/II, and P26/III exhibiting fluorescence quantum yields (ΦF) as high as > 70% (Table 3). The emission of P26/III is especially efficient, with a ΦF value (98%) well approaching unity.
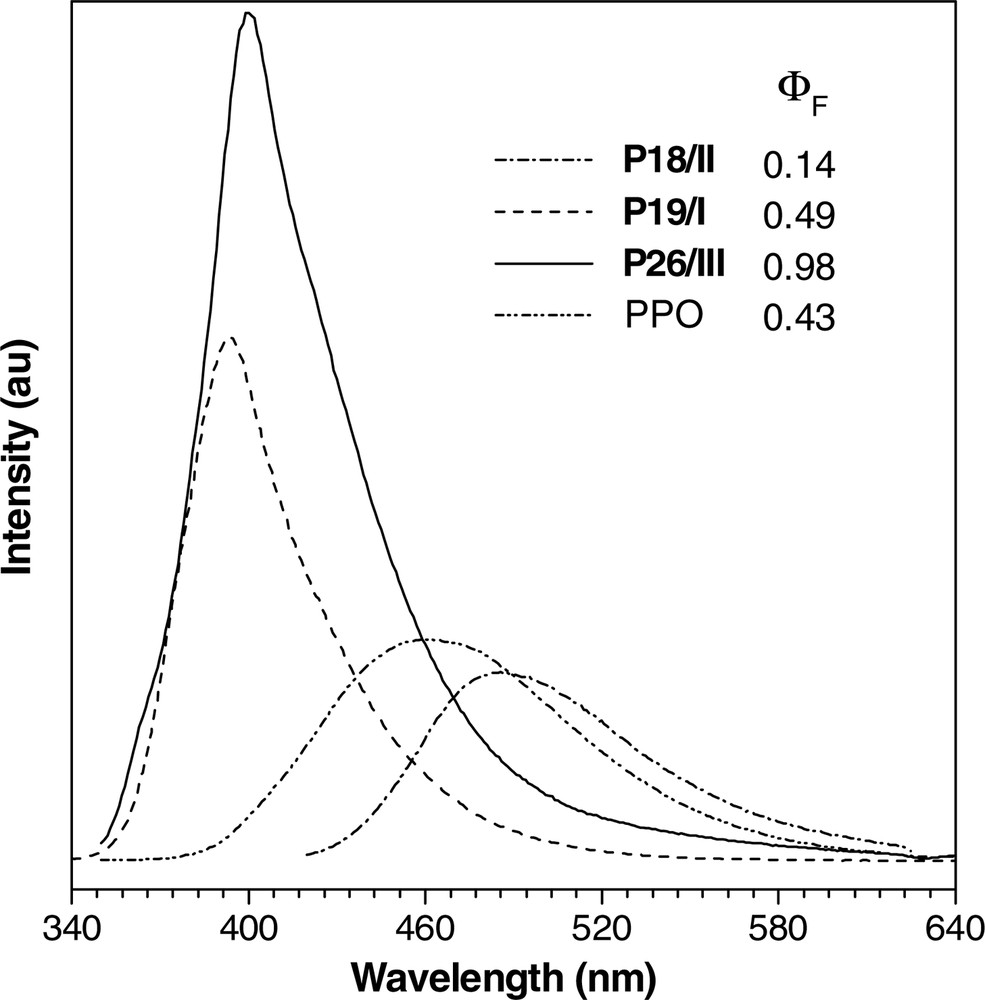
Photoluminescence spectra of dichloromethane solutions of P18/II, P19/I, and P26/III and a THF solution of poly(1-phenyl-1-octyne) (PPO). Concentration: 2 mM; excitation wavelengths (nm): 345 (P19/I and P26/III), 355 (PPO), and 412 (P18/II).
Molecules of fused aromatic rings may limit optical power [60–62]. The HPAs are aromatic macromolecules and are hence good optical limiters. As can be seen from the examples given in Fig. 9, the HPAs effectively attenuate the power of intense 532 nm laser pulses. The transmitted fluence of P20/III initially increases with incident fluence in a linear fashion. It commences to deviate from linearity at an incident fluence of ~260 mJ cm–2 and reaches a saturation plateau at 140 mJ cm–2. Its optical limiting threshold (FL) and signal suppression (Ft,m/Fi,m; Table 3, No. 14) are superior to those of C60, a well-known optical limiter [63]. Compared to P20/III, P24/III is a better optical limiter but P26/II is poorer one. This indicates that the optical limiting performance of an HPA is sensitive to a change in its molecular structure, offering the opportunity to tune its optical limiting properties through molecular engineering endeavor.
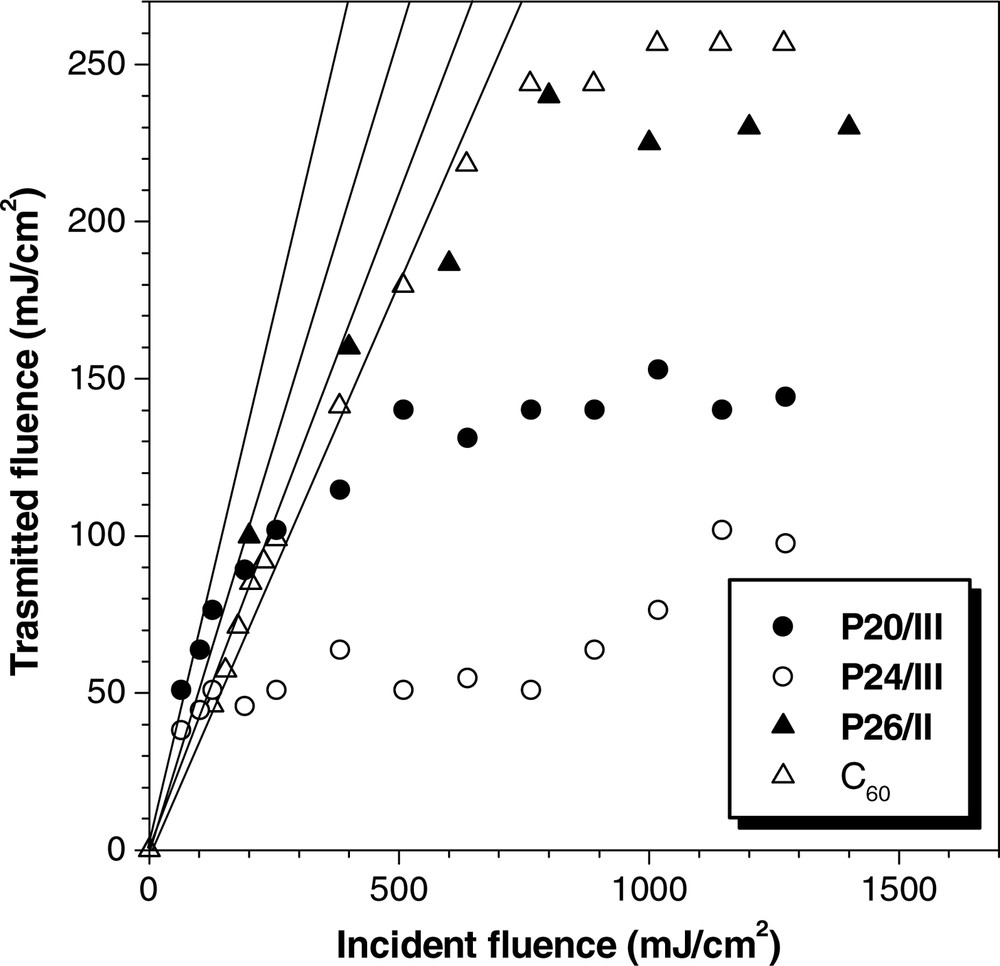
Optical limiting responses to 8-ns, 532-nm optical pulses, of dichloromethane solutions of hyperbranched polyarylenes P20/III, P24/III, and P26/II; concentration: 0.86 mg ml–1. Data for a toluene solution C60 (0.16 mg ml–1) is shown for comparison.
5 Conclusion
New organic and organometallic HPAs are successfully synthesized in high isolation yields by one-pot, transition metal-catalyzed homopolycyclotrimerizations of functional aromatic diynes and their copolycyclotrimerizations with monoynes. All the homo- and co-HPAs are completely soluble in common organic solvents such as THF, dichloromethane, chloroform, and toluene. Thanks to their conjugated molecular structures, the HPAs are efficient light emitters. The aromatic polymers are both thermally and optically stable, losing little of their weights when heated to high temperatures and showing no signs of photodegradation when shot by intense optical pulses. These remarkable attributes make the HPAs promising materials for high-tech applications.
Acknowledgements
We thank the financial support of the Research Grants Council (Project Nos. HKUST 6085/02P and 6121/01P) and the University Grants Committee of Hong Kong (Project No. AoE/P–10/01-1-A).