1 Introduction
The chemical modification of wood with anhydride reagents has been the subject of research for many decades [1–4]. Acetylation has been the most widely used and successful chemical modification and is a single site reaction that replaces a hydroxyl group with an acetyl group. Acetyl groups are more hydrophobic than hydroxyl groups; therefore, replacing some of the hydroxyl groups with acetyl groups reduces the hydrophilic property of the cell-wall polymers [5]. The acetyl group is also larger than the hydroxyl group; therefore, the material undergoes permanent expansion [6]. In general, the reaction leads to an increased content of acetyl groups in the wood material, to approximately 20 wt% compared with 1–2 wt% for unmodified wood. The introduction of new acetyl groups in wood polymers results in a certain degree of bulking of the wood cell walls. This, in combination with the decreased ability to attract water molecules, leads to highly improved dimensional stability of acetylated wood material [7]. On the other hand, chemical modification of whole wood suffers from difficulties in achieving effective penetration of reagent to the bulk of the material, and the disadvantages of subsequent removal of unreacted chemical and by-products. Due to these problems, much research has concentrated upon the modification of wood fibre or chips, but the economics of such a process is in some doubt [1]. In addition, with decreasing wood resources, work has increased regarding the use of non-wood products such as agricultural residues, like raw materials for industrial use.
Wheat straw is one of the major agricultural by-products that are not used as industrial raw material on a significant scale except for only a minor portion that is reserved as animal feed, household fuel or as raw materials for paper industry. Interestingly, straw is similar in chemical composition to wood. The predominant constituents of wheat straw are cellulose (38–40%), hemicelluloses (35–38%), and lignin (14–17%) [8]. The former two components are hydrophilic and the latter is hydrophobic. However, straw is sparingly insoluble in water and in organic solvents only partly because of the hydrogen bonds between polysaccharides and adhesion of lignin to the polysaccharides. However, it would be expected that wheat straw would react with acetic anhydride in a similar manner to wood and that the properties of acetylated wheat straw would be much the same as those of acetylated wood. Acetylated wheat straw could, therefore, represent an abundant, inexpensive, and renewable lignocellulosic biomass as a novel material for environmentally friendly industrial utilization. An example of this is potential usage as a natural sorbent in oil spill cleanup, since acetylation increases the hydrophobic nature of the straw and gives a lowered wettability, but a higher oil absorptivity of the material.
Most of the procedures used to modify wood developed over the years have complicated reaction schemes through the use of an organic co-solvent. Use of a solvent, which reduces the reaction rate because of dilution of modifiers, would require complicated separation procedures to recover the chemicals after the reaction. Organic solvents are often harmful to humans and the environment. Therefore, it is the best to eliminate organic solvents in the reaction system [9]. On the other hand, the addition of catalysts has been proved to accelerate the rate reaction of acetic anhydride with wood, because the reaction is acid or base catalysed [10]. Pyridine-catalysed acetylation is a standard method for the determination of hydroxyl compounds and other acylable substances. The mechanism involves a nucleophilic catalyst with the intermediate formation of the acylpyridium ion [11]. Although pyridine is an effective catalyst in such acylations, it is toxic, has an unpleasant odour and is not suitable for use in large-scale reactions [12]. Hofle et al. [13] reported that a tertiary amine, 4-dimethylaminopyridine (DMAP), is much superior to pyridine as a catalyst for some synthetic acylations, such as typical acylation reactions for primary and secondary alcohols, and is an effective catalyst of analytical acylation by acetic anhydride, having a specific catalytic activity about 104 times greater than that of pyridine. As far as the authors are aware, there has been no report of its use as a catalyst for the acetylation of straw using acetic anhydride as oil sorbents. The present article describes the application of DMAP as a possible straw acetylation catalyst. Results were compared with pyridine and other two tertiary amine catalysts, which have not previously been studied with respect to straw acetylation reactions, N-methyl pyrrolidine (MPI), and N-methyl pyrrolidinone (MPO). FT–IR and solid-state 13C–NMR spectroscopy were performed to investigate the reaction. Thermogravimetric analysis (TGA) and differential scanning calorimetry (DSC) were carried out to study the thermal behaviour of acetylated wheat straw and to compare it with the control. The absorptivity of oil was tested with machine oil.
2 Materials and methods
2.1 Materials and reagents
Wheat straw was obtained from the experimental farm of ‘The North-Western Sciences and Technology University of Agriculture and Forestry’ (Yangling, China). It was dried in sunlight and then cut into 1–2-cm-length small pieces. The cut straw was further dried in an oven at 60 °C for 16 h before use. Acetic anhydride, 4-dimethylamino pyridine (DMAP), pyridine, N-methyl pyrrolidine (MPI), and N-methyl pyrrolidinone (MPO) were purchased from Aldrich-Sigma Company (Beijing).
2.2 Acetylation of wheat straw
In order to determine the relative rates of reaction, the following procedure was adopted. A quantity of straw (approximately 10 g) was placed in a 500-ml round bottom flask containing 200 ml acetic anhydride and either without catalyst or with a catalyst (either pyridine, DMAP, MPI, or MPO) at a concentration of 1, 2, 3, 4, 5, 6, and 7%, based on dried straw used. The flask was then placed in an oil bath set at the required temperature (100 or 120 °C) using atmospheric pressure with a reflux condenser fitted. Following the reaction for the required time (40, 60, 90, 120, 180, or 360 min), the flask was removed from the oil bath, and the hot reagent was decanted off. The straws were then thoroughly washed with ethanol and acetone to remove the un-reacted acetic anhydride and acetic acid by-product. Samples were then dried in an oven at 60 °C for 16 h prior to re-weighing. The oven-dry straws were weighted to determine the weight gains on the basis of initial oven-dry measurements. To reduce errors and confirm the results, each experiment was repeated in triplicate under the same conditions. Weight percent gain (WPG) of the straws due to acetylation was calculated as the average of these three replicates according to:
2.3 Spectroscopic and thermal characterization
The FT–IR spectra of the raw and acetylated straws were recorded on a Nicolet-510 FT–IR spectrophotometer, using KBr pellets containing 1% finely ground samples. Solid-state Cross-Polarisation Magic-Angle Spinning Carbon-13 Nuclear Magnetic Resonance (CP/MAS 13C–NMR) spectra were obtained using a Bruker MSI-300 spectrometer at 74.5 MHz. About 250 mg of sample were packed into zirconium rotors for MAS at approximately 4 kHz. The CP contact time was 5 s, acquisition time 0.1, and total acquisition time 2 h.
Thermal analysis of the unmodified and acetylated straw was performed using thermogravimetric analysis (TGA) and differential scanning calorimetry (DSC) on a simultaneous thermal analyser (Netzsch STA-409). All the measurements were made under nitrogen flow (160 ml min–1) keeping a constant heating rate at 10 °C min–1 and using an alumina crucible with a pinhole. Each sample was heated from room temperature to 600 °C.
2.4 Oil absorptivity
Machine oil (20 g) was suspended in water in a beaker. The acetylated straw (0.5 g) was added and mixed for 1 min at room temperature. The oil was found to be absorbed immediately by the acetylated straw. The straw was then picked out and weighed to determine the oil absorptivity.
3 Results and discussion
3.1 Influence of catalysts, and reaction temperature and time upon the rate of acetylation
Weight-percent gain from acetylation of wheat straw as a function of different catalysts, and reaction temperature and time are given in Table 1. It appears that, at 120 °C, a 40-min increase of DMAP concentration to 6% resulted in an increment of WPG from 13.2 (control sample 1) to 19.6% (sample 7). A further increase in DMAP to 7% did not result in any additional WPG. This is probably due to the limited rate of diffusion of the reagent into the straw matrix at a relatively high concentration of DMAP. Similar results have been reported in the studies of the chloroacetic anhydride modification of wood by Rowell and co-workers [14]. The authors showed that the highest levels of reaction (at low WPG) were observed in the S2 layer of the cell wall, but at high WPG the greatest substitution occurs in the middle lamella, indicating that the reaction kinetics of acetic anhydride with wood or straw is diffusion limited. Hill and Jones [15] also stated that as the reaction proceeds the bulk reactions assume increasing importance, and the reaction rate will therefore be expected to display diffusion-dominated process. In addition, the reaction rate of acetic anhydride with wheat straw may be also controlled by the type of hydroxyl groups present in the straw. These groups can be distinguished as being phenolic, benzylic, or alcoholic on the lignin regions, and alcoholic in the carbohydrate. The alcoholic hydroxyl groups occur as either primary or secondary, and the phenolic hydroxyl groups are attached to an aromatic ring that has various substituents attached [1]. Therefore, each of these groups will exhibit a different reactivity towards acetic anhydride. For example, based on the investigation of acetyl distribution in acetylated whole wood and reactivity of isolated wood cell-wall components to acetic anhydride, Rowell et al. [14] stated that the order of reactivity was found to be lignin > hemicelluloses >> holocellulose. Cellulose did not react with acetic anhydride in the absence of a catalyst.
The effect of different catalysts on the extent of acetylation at 100 or 120 °C for various reaction times
Catalytic acetylation conditions | Acetylated wheat straw | ||||
Temperature (°C) | Reaction time (min) | Catalyst (% dried straw) | Sample No. | WPGa | OAb (g oil g–1 straw) |
120 | 40 | DMAPc, 0% | 1 | 13.2 | 19.1 |
120 | 40 | DMAP, 1% | 2 | 16.0 | 23.3 |
120 | 40 | DMAP, 2% | 3 | 17.6 | 25.1 |
120 | 40 | DMAP, 3% | 4 | 17.8 | 25.5 |
120 | 40 | DMAP, 4% | 5 | 18.4 | 27.1 |
120 | 40 | DMAP, 5% | 6 | 18.8 | 27.2 |
120 | 40 | DMAP, 6% | 7 | 19.6 | 28.6 |
120 | 40 | DMAP, 7% | 8 | 19.6 | 28.8 |
100 | 40 | DMAP, 2% | 9 | 13.8 | 19.6 |
100 | 40 | Pyridine, 2% | 10 | 8.4 | 12.4 |
100 | 40 | MPId, 2% | 11 | 10.2 | 15.4 |
100 | 40 | MPOe, 2% | 12 | 10.0 | 14.8 |
100 | 40 | DMAP, 6 | 13 | 15.4 | 23.1 |
100 | 40 | Pyridine, 6% | 14 | 10.4 | 15.8 |
100 | 40 | MPI, 6% | 15 | 10.6 | 15.9 |
100 | 40 | MPO, 6% | 16 | 9.0 | 13.6 |
100 | 40 | Without catalyst | 17 | 7.8 | 12.0 |
120 | 60 | DMAP, 2% | 18 | 17.8 | 25.6 |
120 | 90 | DMAP, 2% | 19 | 18.0 | 26.0 |
120 | 120 | DMAP, 2% | 20 | 18.2 | 26.4 |
120 | 180 | DMAP, 2% | 21 | 18.2 | 26.6 |
120 | 360 | DMAP, 2% | 22 | 18.4 | 27.5 |
a WPG represents the weight percent gain of the product based on the starting dried wheat straw.
b Abbreviation for oil absorptivity (g oil g–1 acetylated straw).
c Abbreviation for 4-dimethylamino pyridine.
d Abbreviation for N-methyl pyrrolidine.
e,f Abbreviation for N-methyl pyrrolidinone.
Since the reaction between straw and acetic anhydride is acid or base catalysed, as mentioned above, the four tertiary amine catalysts used all accelerate the reaction, as shown by an increase in WPG (samples 9–12) as compared to the control. In comparison, pyridine had little effect upon the rate of reaction as shown by a WPG value of 8.4% obtained at 100 °C for 40 min (sample 10) when 2% pyridine was used, with the data points falling very close to those for neat acetic anhydride (7.8%, sample 17). DMAP showed the fastest rate of reaction (WPG 13.8%, sample 9). MPI and MPO exhibited similar effect on the rate of acetylation (WPG 10.2%, 10.0%, samples 11, 12), and both accelerated faster than pyridine. However, at a relatively high concentration of 6%, both pyridine and MPI catalysed the reaction at a faster rate than MPO (samples 14–16). This strongly supports the results obtained by Hill et al. [12] in the studies of potential catalysts for the acetylation of wood. The initial step in the mechanism for the reaction of acetic anhydride with a hydroxyl group involves an attack of the acyl carbon centre of the acetic anhydride molecule by a nucleophile such as DMAP; a subsequent loss of acetic acid generates the ester. Therefore, the rate of the reaction will depend on the nucleophilicity of the catalysts [16].
The results in Table 1 also showed that the WPG increased with an increment in reaction temperature and time. Clearly, a relatively higher value of WPG (13.2%, sample 1) occurred at 120 °C for 40 min without catalyst, whereas it dropped to only 7.8% at 100 °C for the same reaction time in the absence of catalyst. Similarly, at 100 °C with 2% DMAP as a catalyst, the WPG was 13.8% (sample 9), whilst it rose to 17.6% (sample 3) when the reaction temperature increased to 120°C. The reason for this increment of acetylation by rising temperature was probably due to the favourable effect of temperature on compatibility of the reaction ingredients, swellability of the straw, diffusion of the esterifying agent, and mobility of the reactant molecules [17]. On the other hand, a slight increment of acetylation rate (WPG from 17.8 to 18.4%) by prolonging the reaction time (from 40 to 360 min, samples 18–22) at 120 °C with 2% DMAP as a catalyst is a direct consequence of the favourable effect of time on diffusion and adsorption of the reactants between the acetic anhydride and straw fibres. This indicated that the reaction rate increased significantly with the increment of reaction temperature, and the reaction rate increased slightly with an increment in reaction time at 120 °C with 2% DMAP as a catalyst. Overall, at higher temperature or at low temperature such as 100 °C with a catalyst, acetic anhydride was able to swell the straw, making reactive chemical sites more accessible, and therefore increasing the reaction rate. All acetylated straws were found to be brighter and lighter in colour. This is attributed to the process having removed some extractives, imparting a lighter coloration.
3.2 FT–IR spectra
The reaction between wheat straw and acetic anhydride was monitored by observing in the FT–IR spectra a reduction in the OH stretching band and the appearance of ester carbonyl absorbances. As illustrated in Fig. 1 , the four major changes that are observed on acetylation in the FT–IR spectrum of acetylated wheat straw sample 2 (spectrum 2), prepared at 120 °C for 40 min with 1% DMAP as a catalyst, compared to that of the unmodified straw sample (spectrum 1), are: (1) a reduction in the hydroxyl (O–H) stretching band at 3449 cm–1; (2) an increase in the carbonyl (C=O) stretching absorbance at 1752 cm–1; (3) an increment in carbon–hydrogen bending (C–H) at 1381 cm–1 in the acetyl group; and (4) an enhancement in carbon–oxygen (C–O) stretching at 1242 cm–1 in an –O–(C=O)–CH3 group. A strong band at 1056 cm–1 is assigned to C–O stretching in cellulose, hemicelluloses, and lignin [18,19]. The lack of peaks in region 1840–1760 cm–1 and at 1700 cm–1 in spectrum 2 indicated that the product is free of the unreacted acetic anhydride and of the by-product, acetic acid.

FT–IR spectra of unmodified wheat straw (spectrum 1) and acetylated wheat straw sample 2 (spectrum 2) prepared at 120 °C for 40 min with 1% DMAP as a catalyst.
Fig. 2 displays the FT–IR spectra of acetylated wheat straw samples prepared at 120 °C for 40 min without catalyst (spectrum 1, sample 1) and with DMAP as a catalyst at concentrations of 3% (% dried unmodified straw) (spectrum 2, sample 4) and 6% (spectrum 3, sample 7). The similar spectral profiles indicate the similar structures of the acetylated wheat straws. However, on a close comparison of the spectra, some small differences are observed. The signals for three ester bands at 1759, 1374, and 1248 cm–1 increased slightly from spectrum 1 to 2, and to 3, which corresponds to an increment of WPG from 13.2% (sample 1) to 17.8% (sample 4), and to 19.6% (sample 7). In contrast, the absorbance for the unexchanged hydroxyl band at 3449 cm–1 diminished from spectrum a to b, and to c as the increment of WPG went from sample 1 to 4, and to 7.
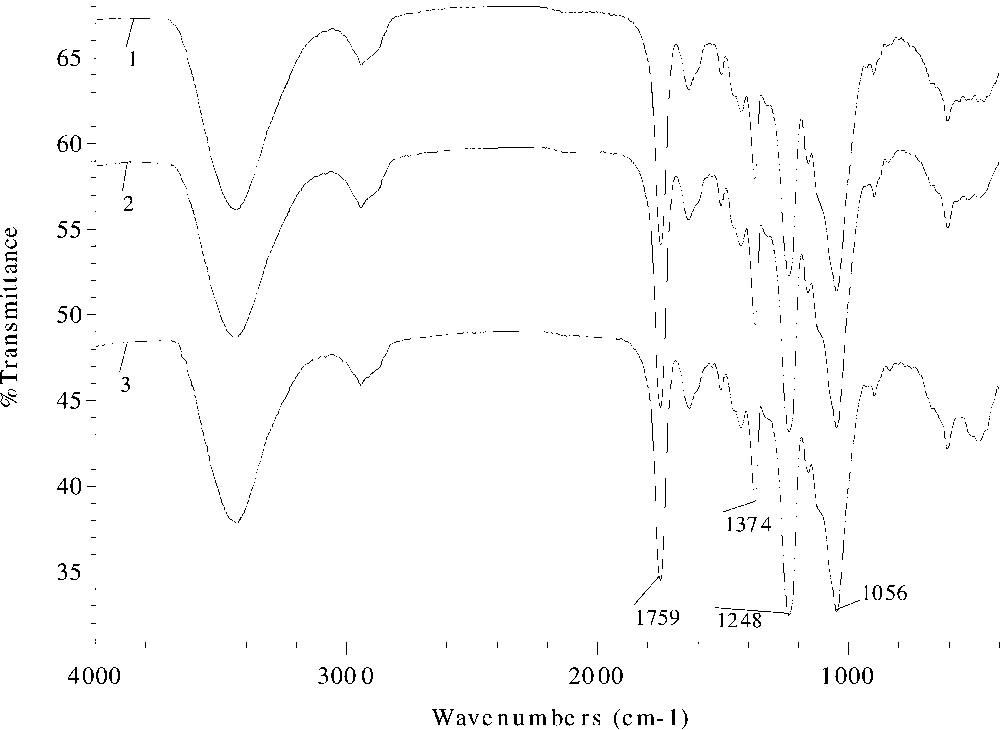
FT–IR spectra of acetylated wheat straw samples prepared at 120 °C for 40 min without catalyst (spectrum 1, sample 1) and with DMAP as a catalyst at the concentration of 3% (% dried unmodified straw) (spectrum 2, sample 4) and 6% (spectrum 3, sample 7).
The effects of different catalysts and reaction duration on the absorption intensity in the FT–IR spectra are given in Figs. 3 and 4 , respectively. Evidently, the ester band intensity at 1746 or 1752, 1381, and 1242 cm–1 increased from spectrum 2 (sample 10) to spectra 3 and 4 (samples 11, 12), and to spectrum 1 (sample 9), proportionally to the increase in the values of WPG. Similar increasing trend of the peak intensity with reaction time was observed in samples 18–20 and 22 in Fig. 4, thereby providing evidence for successful acetylation.

FT–IR spectra of acetylated wheat straw samples prepared at 100 °C for 40 min with 2% DMAP (spectrum 1, sample 9), 2% pyridine (spectrum 2, sample 10), 2% MPI (spectrum 3, sample 11), and 2% MPO (spectrum 4, sample 12) as catalysts.

FT–IR spectra of acetylated wheat straw samples prepared at 120°C with 2% DMAP as a catalyst for 60 (spectrum 1, sample 18), 90 (spectrum 2, sample 19), 120 (spectrum 3, sample 20), and 360 min (spectrum 4, sample 22).
3.3 CP–MAS 13C–NMR spectra
In order to determine whether a chemical reaction was taking place between the straw and acetic anhydride, samples were also subjected to analysis by CP–MAS 13C–NMR spectroscopy. Fig. 5 shows the spectra of un-reacted wheat straw (a) and acetylated straw sample 7 prepared with 6% DMAP as a catalyst at 120°C for 40 min. Evidently, both spectra were dominant and very similar in the carbohydrate region (60–110 ppm), namely C-1 (106.2 ppm), C-4 (90.0 ppm, crystalline, and 85.1 ppm, amorphous), C-2, C-3 and C-5 (75.6 ppm), and C-6 of cellulose and C-5 of xylan (65.2 ppm). A decrease in the intensity of the C-2 band in the spectrum of acetylated straw revealed that acetylation at C-2 might have occurred. This is important because it infers that in the straw itself reaction of acetic anhydride on straw carbohydrates occur. A broad signal at 58.4 ppm and a sharp peak at 34.7 ppm arise from methoxyl (OCH3) and methylene (CH2) groups in lignin, respectively [20]. Occurrence of a very small decrease in the intensity of a band at 58.4 ppm in acetylated straw sample (spectrum b) implied that de-methylation of the methoxy groups does take place under the reaction condition given. More importantly, in Fig. 5b, the methyl band of the acetyl group at 21.4 ppm and the carboxylic group at 171.1 ppm clearly verified the presence of acetyl groups in modified wheat straw. A slight increase in lignin band at 153 ppm (C-3/C-5 in syringyl units) and an enhancement of peak at 116 ppm (C-5 in guaiacyl units and C-3/C-5 of 4-hydroxyphenyl units) demonstrated that new substituents have been introduced in the aromatic ring of lignin [21].

CP–MAS 13C–NMR spectra of un-reacted wheat straw (a) and acetylated straw sample 7 prepared with 6% DMAP as a catalyst at 120 °C for 40 min (b).
3.4 Thermal analysis
The thermal decomposing pattern of native wheat straw and acetylated straw samples 1 and 7 (acetylated at 120 °C for 40 min without and with 6% DMAP as a catalyst) gave additional evidence to the relatively higher stability of the acetylated wheat straw (Fig. 6 ). As illustrated in Fig. 6, the TGA curves of native wheat straw and acetylated straw samples 1 and 7 started to decompose at 213 (Fig. 6a), 240 (Fig. 6b), and 254 °C (Fig. 6c), respectively. At 10% weight loss, decomposition of the native wheat straw and of the two acetylated straw samples 1 and 7 occurred at 253, 286, and 293°C, respectively. This first decomposition step is due to hemicelluloses. Similarly, at 50% weight loss, the decomposition temperature was observed at 300 °C for native wheat straw, 335 °C for acetylated straw sample 1, and 340 °C for acetylated straw sample 7, which is mainly attributed to decomposition of cellulose. This increasing trend of decomposition temperature indicated that the thermal stability of the acetylated straw is higher than that of the untreated wheat straw, and the thermal stability increased with an increment of WPG.

Thermograms of native wheat straw (a) and acetylated straw samples 1 prepared at 120 °C for 40 min without catalyst (b) and 7 prepared at 120 °C for 40 min with 6% DMAP as a catalyst (c).
The DSC thermograms of native straw and the corresponding two acetylated straw samples in an N2 atmosphere gave a small broad peak below 100 °C due to moisture desorption [22]. The decomposition was mainly governed by the exothermic peak at 307 °C for unmodified wheat straw. For acetylated straw samples 1 and 7, the exothermic peak was shifted to a higher value of 340 and 348 °C, respectively, which indicated again that the thermal stability of acetylated wheat straw increased.
3.5 Oil absorptivity
In recent years, there has been a worldwide pollution problem from the devastating results of oil spills being washed up on coastlines and the subsequent need to effectively remove them. In addition, there have been tremendous increases of accidental and intentional oil discharges during production, transpiration, and refining, which cause immense environmental damage. One of the most economical and efficient means for the removal of spilled oil from either land or sea is the use of sorbents [23]. Synthetic sorbents such as polypropylene and polyurethane are the most commonly used commercial sorbents in oil-spill cleanup, due to their oleophilic and hydrophobic characteristics [24]. These synthetic sorbents, however, are often either non-biodegradable or only very slowly biodegradable, which is environmentally undesirable and incineration is very expensive. Natural sorbents such as acetylated wheat straw, which have the characteristics of low cost, high capacity, quick uptake, and easy desorption, can offer distinct advantages in such regard. They are like biodegradable sorbents and, as such, preferable to minimize subsequent environmental problems.
The oil absorption of the acetylated wheat straws was examined using machine oil. The oil was suspended in water and treated with acetylated-straw samples. The machine oil in water was immediately absorbed by the acetylated straw, which does not absorb water. The capacity of oil absorbance of the samples was 12.0–28.8 g g–1 of acetylated straw at room temperature (Table 1), which increased with the increment of WPG. In comparison, the oil sorption capacities of the modified straws were much greater than polypropylene fibres or polypropylene web (~10 g of light crude oil per gram of the synthetic fibre at room temperature) [25]. That is, the oil absorption capacity was approximately 1.2–2.9 times higher than that of polypropylene web or fibres. Therefore, the acetylated wheat straws can be used as potential sorbents to substitute non-biodegradable synthetic materials in oil-spill cleanup. Furthermore, based on the results obtained in our laboratory, the acetylated straw sorbents can be recycled several times (maximum 11 times) for oil spill cleanup by a simple squeezing operation, which was sufficient to remove most of the oil sorbed on the modified straws.
In conclusion, the consequences of all the above are that in wheat straw acetylation occurs on both the lignin and carbohydrate components. It was found that the reaction temperature significantly affects the level of acetylation with or without catalyst. Of the four tertiary amine catalysts used, DMAP was observed to produce a large acceleration in the rate of reaction of acetic anhydride with wheat straw, and the rate of reaction increased with an increment of its concentration between 1 and 6%. MPI and MPO exhibited at a faster rate to accelerate the acetylation than pyridine at 100 °C for 40 min when 2% catalyst was used, while when the concentration of the catalyst rose to 6%, at the same given reaction conditions, MPI and pyridine were more accelerating than MPO. The maximum WPG found was 19.6% for the acetic anhydride-6% DMAP system at 120 °C for 40 min, whereas using only acetic anhydride WPG was 13.2% at the same reaction condition. The thermal stability of acetylated wheat straw was found to be higher than that of the unmodified straw. Replacing some of the hydroxyl groups with acetic anhydride substantially increased the hydrophobic property of the cell-wall polymers, as shown by having 1.2–2.9 times higher oil absorption capacity than that of non-biodegradable synthetic sorbents such as polypropylene fibres. These results suggested that a total or partial substitution of commercial synthetic oil sorbents by acetylated wheat straw sorbents could be beneficial in the oil spill cleanup operation by improving the efficiency of oil sorption and by the incorporation of other advantages such as biodegradability.
Acknowledgements
The authors are grateful to the National Natural Science Foundation of China for its financial support of this research (Nos. 30271061 and 30025036) and the Guangdong Natural Science Foundation (Nos. 013034 and 36567).