1 Introduction
The paraxylene market has enjoyed high growth rates over the years and it is expected that this trend will continue for the future. Xylenes are produced from catalytic reforming of naphtha. Isomerization combined with separation allows to produce high purity paraxylene in agreement with the market demand. For this reason, the separation of paraxylene is a key operation which requires high purity, high yield, and high capacity. Adsorption is widely used to perform the separation, because of its high efficiency. The adsorbents which are used for selective adsorption of paraxylene are X or Y zeolites exchanged with adequate cations. Liquid phase Simulated Counter Current adsorption is mainly used as it is the most efficient process [1].
The primary requirement for an economic separation process is an adsorbent with sufficiently high selectivity, high capacity, as well as high mass transfer rates. These separation properties are strongly dependent upon the zeolite parameters as depicted by numerous studies.
- - The first parameter which can influence the adsorption behavior is the compensating cation. For example, NaY is selective for metaxylene and KY is selective for paraxylene. This is clearly illustrated by the values given in the patents [2]. At the same time, diffusion processes are influenced by the cations as shown in recent papers [3,4].
- - The Si/Al ratio is also important as demonstrated by Seko et al. [5]. According to these results, paraxylene selectivity is increasing with increasing Si/Al ratio in the range 1.2–3.
Therefore, it clearly appears that the cation size, number and positions strongly influence the adsorption and diffusion properties.
In addition, Cottier et al. [6] demonstrated that loading is a key parameter which can strongly influence the adsorption. For example, the selectivity for paraxylene on KY is only obtained at high loading of the zeolite (over two molecules per supercage). The structural work performed by Mellot et al. [7–9], Descours [10] and Pichon et al. [11,12] provided a good insight into this phenomenon. Using neutron powder diffraction combined with the Rietveld method, they located xylene molecules in the zeolite cavities as a function of loading. They demonstrated that in BaX zeolite, the first molecules are adsorbed close to the site II cation (Ba) and that an increase of the loading leads to a large rearrangement and to a minimization of the interactions between the aromatic ring and the cations. This is associated with increasing interactions between the methyl groups and the framework oxygens. In this respect, paraxylene selectivity is only obtained because of a favorable packing in the supercage.
The last key parameter is the preadsorption of small polar molecules. It is well established now that preadsorbed water can also modify the adsorption of xylenes in BaX zeolite. This is illustrated by the work of Furlan et al. [13] who put into evidence a maximum in the selectivity as a function of the water content. It was demonstrated by Pichon et al. [11,12] that the water molecules are strongly interacting with the cations and consequently weakening the interactions between the aromatic ring and the cation. It has also been shown by pulsed-field gradient NMR (PFG NMR) that low quantities of water have a large effect on the self-diffusion of unsaturated hydrocarbons [14].
As the presence of water is important for the adsorption selectivity, it is worth considering the dynamics of this preadsorbed molecule and its influence on the zeolite itself. The diffusion of water in 4A and 5A zeolites has recently been studied by quasi-elastic neutron scattering (QENS) and PFG NMR techniques [15]. The diffusion of water in BaX zeolite has not been yet studied by these microscopic techniques.
We report here QENS results obtained for water adsorbed at different loadings in BaX. The behavior of water in this zeolite is quite different compared to NaCaA zeolites.
2 Experimental
The BaX zeolite was prepared by ion exchange in successive batches, starting from the NaX form. The final degree of exchange was 95%. It was checked that no dealumination or loss of crystallinity occurred during the exchange reactions. The zeolite was activated by heating to 575 K under flowing oxygen. After cooling, the zeolite was pumped to 10–4 Pa, while heating up to 625 K. Several samples were prepared by adsorbing known amounts of water onto the activated zeolite, they correspond to five, 10, and 15 molecules of water per supercage (or 4.15%, 8.3% and 12.5% of the dried weight of the zeolite, respectively). The samples were transferred, inside a glovebox, into aluminum containers of annular geometry. All samples were equilibrated at 375 K during 10 h. A cell containing the dehydrated zeolite was also prepared. Its signal was subtracted from the spectra recorded with the cells containing adsorbed water.
The neutron experiment was performed on the backscattering spectrometer IN10 at the Institut Laue-Langevin, Grenoble. The containers were placed in a cryofurnace. The incident neutron energy was 2.08 meV (6.27 Å), using a Si(111) monochromator. Quasi-elastic measurements were performed by applying a Doppler shift to the incident neutrons through a movement of the monochromator. Spectra were recorded at different scattering angles, corresponding to neutron momentum transfer values, Q, ranging from 0.15 to 1.45 Å–1. The resolution function could not be fitted with a simple analytical function so that numerical convolution was employed (the full width at half-maximum of the energy resolution is of the order of 1 μeV). The energy transfer was analyzed in a window of ±11 μeV. The scattered intensity is dominated by the hydrogen atoms motions, because of the large incoherent cross section of hydrogen.
X-ray diffraction patterns were recorded using a Bruker D5005 diffractometer (Cu Kα radiation).
3 QENS results
QENS spectra obtained for BaX loaded with 15 water molecules per supercage are shown in Fig. 1. The temperature of the cryofurnace was set at 450 K, the sample being at 440 K. The spectrum which was the first measured is Fig. 1(a). While the sample was kept at 440 K, new spectra were measured as a function of time, each run lasting 4 h (the memory was erased after each run). From the sequence of spectra shown in Fig. 1, it appears that the elastic intensity increases while time increases. Since this observation was unexpected, the sample was removed out of the cryofurnace after measuring the spectrum shown in Fig. 1(e). It was checked that the weight of the sample had not changed. The sample was inserted again in the cryofurnace, which was set initially at 500 K. While the temperature of the sample was rising, the detectors and the electronics of the spectrometer were tested. In order to check that all this procedure had not modified the counting rates, it was decided to measure the sample again at 440 K. The regulation temperature was reset at 450 K, but during a short time interval the sample temperature had gone above 450 K. A new run was started after the sample temperature had reached equilibrium at 440 K, it corresponds to Fig. 2(a). This spectrum is identical to the one reported in Fig. 1(e). Spectra shown in Fig. 2(a), (b) were obtained after 2 h, and 2(c) after 11 h (the signal is constant). The sample was then heated at 484 K and measured during 12 h, the signal was purely elastic and no variation of intensity was observed during that time. The sample was then heated at 520 K, and during a measuring time of 14 h, no evolution of the spectrum was observed, the intensity being strictly elastic. The X-ray diffraction pattern of this sample, after the QENS experiment, is shown in Fig. 3(c).
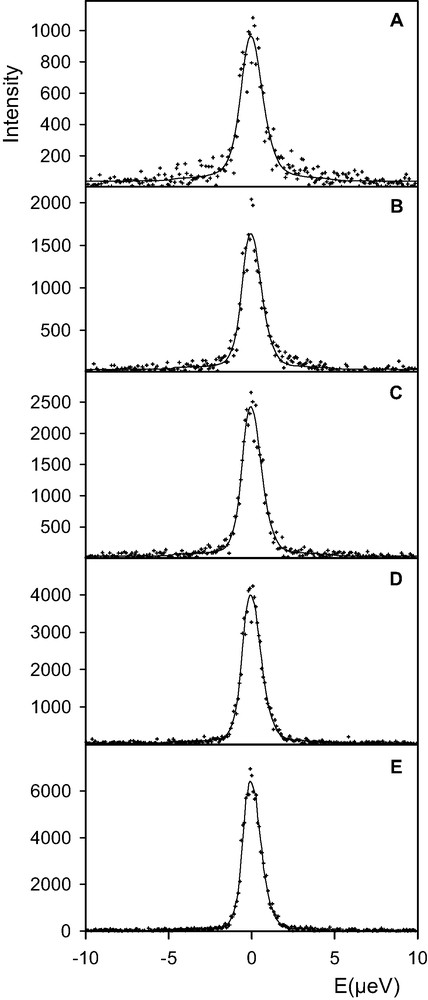
Comparison between experimental (+) and calculated (solid lines) QENS spectra obtained for a concentration of 15 water molecules per supercage of BaX (Q = 0.15 Å–1). The temperature of the sample was 440 ± 1 K. Spectra were recorded from (a) to (e) (duration of one run: 4 h).
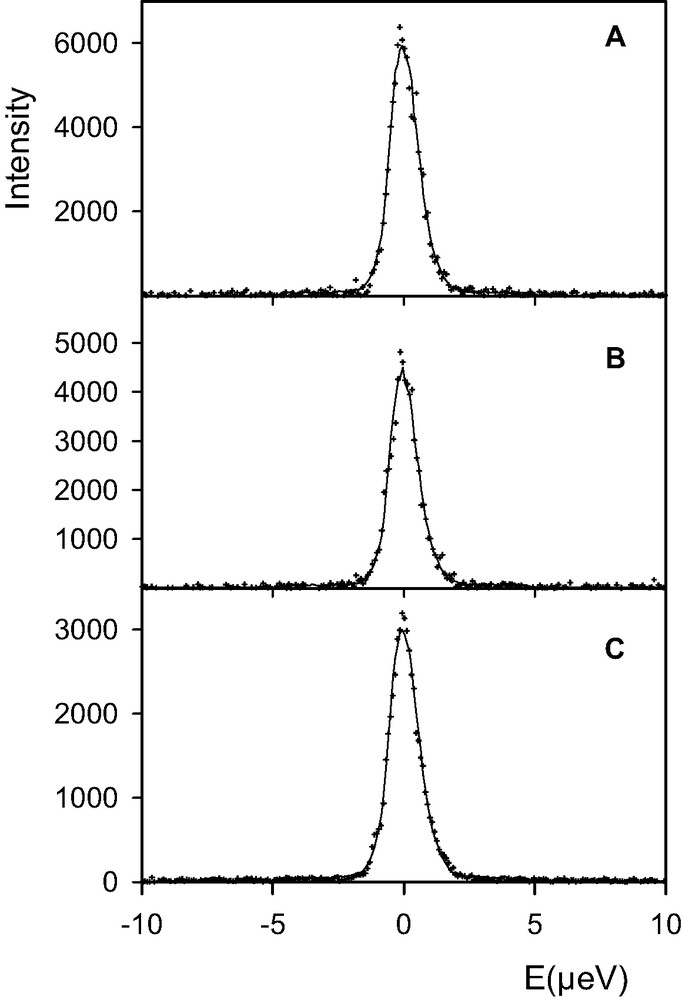
Comparison between experimental (+) and calculated (solid lines) QENS spectra obtained for a concentration of 15 water molecules per supercage of BaX (Q = 0.15 Å–1). The temperature of the sample went briefly above 450 K, and then equilibrated at 440 K. Spectra were recorded from (a) to (c) (duration of the first two runs: 2 h, of the last one: 11 h).
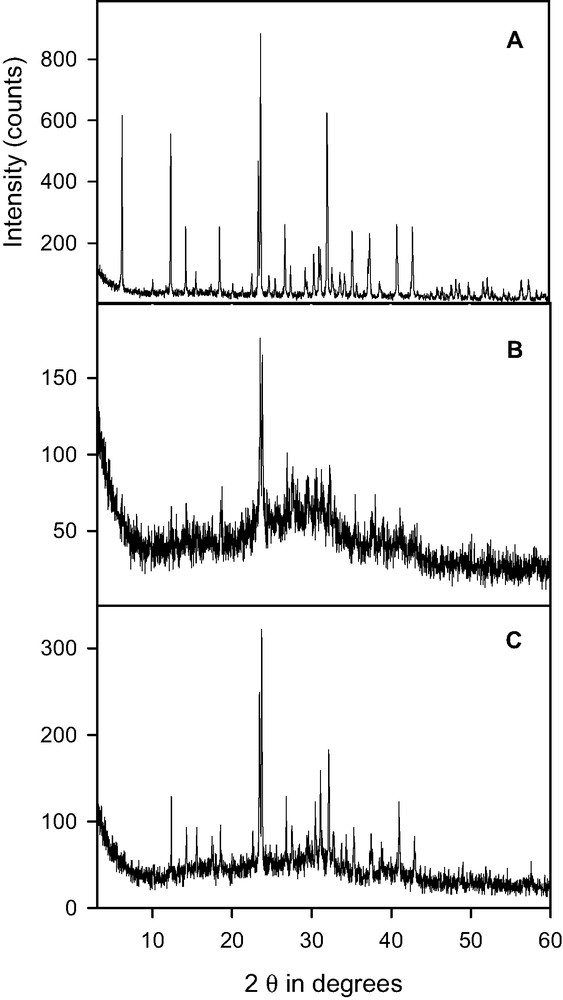
X-ray diffraction patterns of the different samples after the QENS experiments. The number of water molecules per supercage is (a) 5; (b) 10; (c) 15.
The results obtained for the zeolite loaded with 10 water molecules per supercage are different. The QENS spectrum recorded for a sample temperature of 440 K is shown in Fig. 4(a). No evolution of the signal was noted during a total run time of 18 h. The sample was then heated at 484 K, Fig. 4(b), again the intensity did not vary during a recording time of 12 h. On the other hand, after heating the sample at 520 K, the measured intensity was found to increase with time, Fig. 4(c–e). The X-ray diffraction pattern of this sample, recorded after the QENS experiment, is shown in Fig. 3(b).
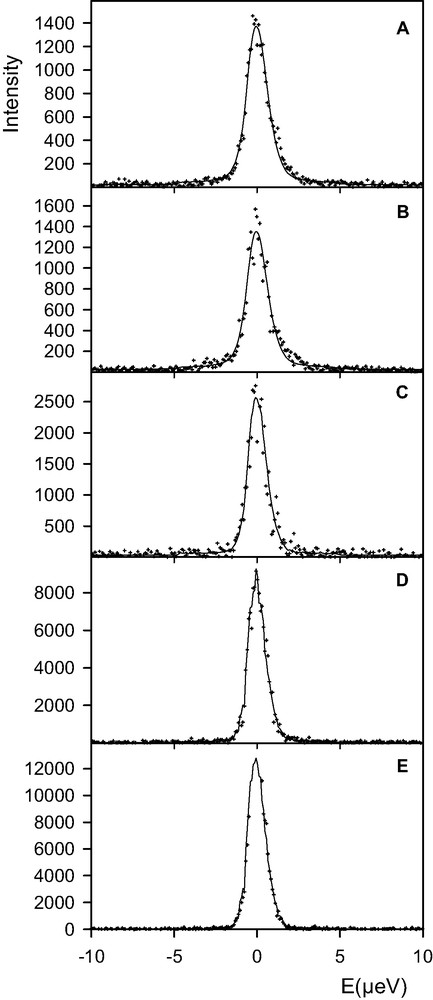
Comparison between experimental (+) and calculated (solid lines) QENS spectra obtained for a concentration of 10 water molecules per supercage of BaX (Q = 0.15 Å–1). Temperature of the sample: (a) 440 K (run of 18 h); (b) 484 K (run of 12 h); (c), (d), and (e): 520 K (runs of 2 h).
No variation of intensity could be observed at the different temperatures for the last sample, loaded with five water molecules per supercage. The QENS spectra are shown in Fig. 5(a–c). The X-ray diffraction pattern recorded after the QENS experiment is reported in Fig. 3(a).
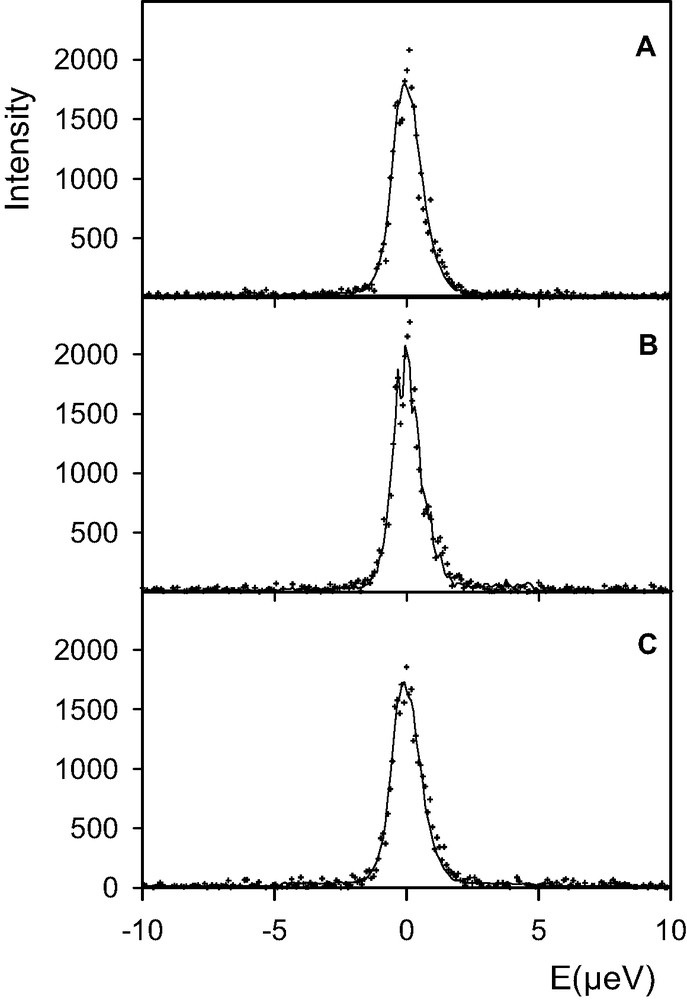
Comparison between experimental (+) and calculated (solid lines) QENS spectra obtained for a concentration of five water molecules per supercage of BaX (Q = 0.15 Å–1). Temperature of the sample: (a) 440 K; (b) 484 K; (c) 520 K (runs of 20 h each).
4 Discussion
The QENS spectra shown in the different Figures correspond to a momentum transfer value, Q, of 0.15 Å–1 [16]. The spectra recorded at 0.27 Å–1 confirmed the observed trends and those measured at higher Q values were polluted by Bragg scattering because of the low Q resolution of a backscattering spectrometer [17]. The spectra obtained at the lowest Q value are easier to interpret. The reason is that any broadening of the instrumental resolution can only be due to the long-range diffusion of water. The length scale probed at this Q value is 42 Å, which is much larger than the cage-to-cage distance. Contribution to the quasi-elastic profile from rotational motions appears at larger Q values. The diffusion coefficient of water can be estimated from the broadening at the lowest Q value. As an example, the spectrum in Fig. 1(a) shows a broadening of the elastic peak, whereas in Fig. 1(e) the profile corresponds to the instrumental resolution. When the spectrum is strictly elastic, like in Fig. 1(e), this means that no diffusion can be measured on the time scale of the experiment, ≈10 ns.
The sample corresponding to the highest loading, 15 water molecules per supercage, shows a continuous evolution of intensity at 440 K, during 20 h (Fig. 1). After 4 h, the diffusion coefficient of water is 1.3 × 10–10 m2 s–1 (Fig. 1a). The value of this diffusion coefficient decreases with time, and after 20 h, the intensity is only elastic, indicating a diffusion coefficient lower than 10–12 m2 s–1. Simultaneously, the total intensity increases. This indicates that after sample preparation: water adsorption and heating at 375 K, the sample was not in thermal equilibrium. When the sample temperature is raised to 440 K, there are still mobile molecules, Fig. 1(a). The increase of intensity is due to the slowing down of the dynamics of water molecules. The molecules that were too mobile to be observed at the beginning of the measurement, Fig. 1(a), progressively enter the energy window of the spectrometer and are detected, Fig. 1(b–e). This shows that a relatively high temperature, 440 K, is needed to get equilibrium at this water loading, in BaX zeolite. It should be noted that this was not the case in NaCaA zeolites, which were equilibrated at 373 K only, and where variations in intensity were not detected as a function of time [15]. Remember that in QENS, one observes the hydrogen atoms, so that changes of the dynamics of water, as indicated in Fig. 1, may also be accompanied by a redistribution of the Ba cations on the different sites.
The sample with 15 water molecules per supercage was briefly heated above 450 K, before it stabilized at 440 K. The QENS spectra, Fig. 2, show a decrease of intensity with time. To understand this behavior, new samples were prepared in the same conditions as for the QENS experiment. The same quantity of zeolite was loaded with 15 water molecules per supercage and heated in a neutron container. X-ray diffraction profiles obtained after heating at 443 and 453 K are shown in Fig. 6(a), (b), respectively. It appears that the crystallinity of the sample is affected by the thermal treatment at 453 K. Therefore, the evolution of the spectra shown in Fig. 2 reflect changes in the framework in addition to cation migration and water dynamics.
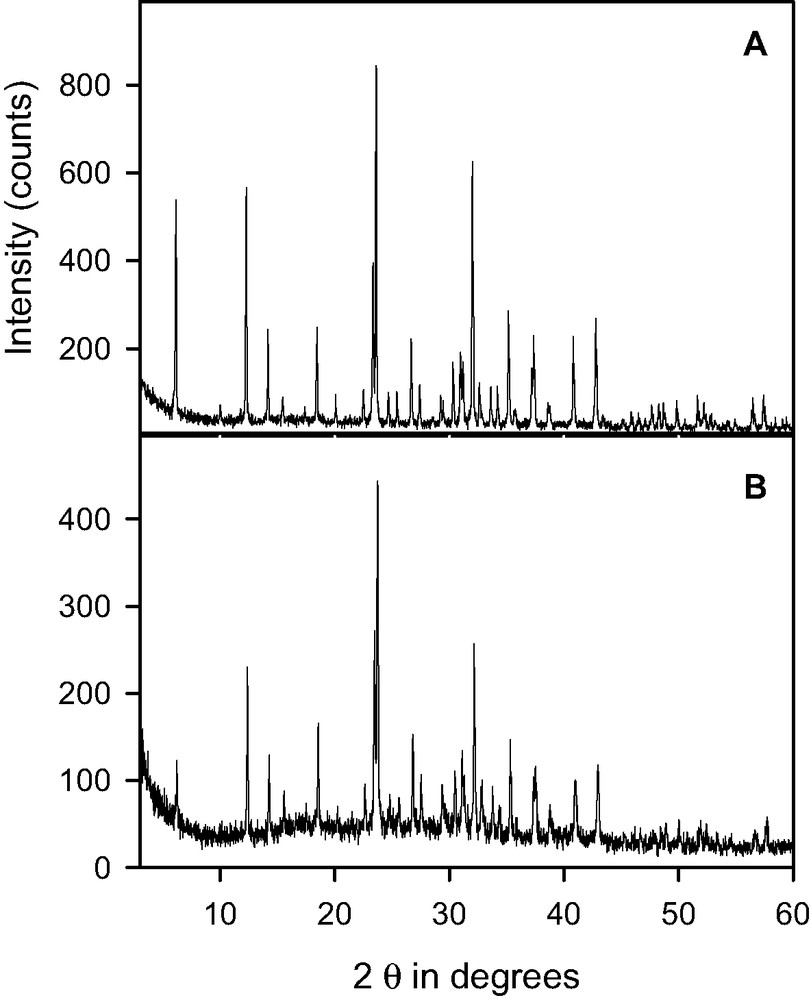
X-ray diffraction patterns of BaX zeolite loaded with 15 water molecules per supercage and heated at (a) 443 K, (b) 453 K (the data are recorded at room temperature).
For a lower loading, 10 water molecules per supercage, the QENS results indicate that a higher temperature, 520 K, is needed to reach equilibrium (Fig. 4). At the end of the measurements at 520 K, Fig. 4(e), the intensity is purely elastic so that the diffusivity of water is less than 10–12 m2 s–1.
For the lowest loading, five water molecules per supercage, the QENS intensity varies neither with time or temperature (Fig. 5). A comparison between the spectra shown in Figs. 5(c) and 4(e) indicates that the main proportion of water is not ‘seen’ at the lowest concentration, even at 520 K (Fig. 5). This indicates that there are still highly mobile molecules, whereas at a larger loading the molecules have reached their equilibrium positions, they are firmly bound and have a very low diffusivity. The X-ray diffraction pattern of the zeolite loaded with five water molecules per supercage, Fig. 3(a), shows that this sample has not been damaged during the whole QENS measurements. On the other hand, the samples containing a larger quantity of water have lost crystallinity after heating at the same temperature of 520 K.
In NaCaA zeolites, the diffusion coefficient of water was found to increase with increasing loading [15]. However, the diffusivities derived from QENS were always larger than 10–10 m2 s–1, at 520 K. In BaX, the diffusion coefficient of water is lower than 10–12 m2 s–1, when water molecules have reached their equilibrium positions. This indicates that in the separation process of xylenes using BaX, the water molecules are not more mobile than the xylenes molecules [4]. The proximity of the water molecules near the SII Ba cations are expected to weaken interactions of the aromatic ring of xylene molecules with barium atoms. This is in agreement with the neutron diffraction studies. The separation process itself must be influenced by these results. The dynamics of water molecules together with its interactions with the cations are likely to modify the adsorption and diffusion rates of the xylene molecules. Consequently, the amount of preadsorbed water has to be accurately controlled in order to monitor the adsorbent performances.
5 Conclusions
The adsorption of water in BaX zeolite is much more complicated than in A-type zeolites. Regarding the adsorption, one finds that a much higher temperature is necessary for water to access high energy sites. This temperature depends on the water loading: the lower the water content, the higher the temperature. It appears that the diffusion coefficient of water increases when the loading increases, adsorbed molecules being more mobile at saturation. This may explain the hysteresis loops observed in thermogravimetric measurements [18]. The very low diffusivity of water in BaX also explains partially the very low adsorption and desorption rates observed by Moïse et al. [18]. However, to account for the evolution of the QENS spectra with time, one cannot easily settle between water or cation motions.
The diffusion of water could only be measured when the samples were not in equilibrium. In order to determine the very low diffusivity of water in equilibrated samples, one would have to use the neutron spin echo technique [4].