1 Introduction
Polyamine ligands form stable complexes with 3d metal ions. Stability increases with ligand's denticity due to the chelate effect [1]. In the case of copper(II), the highest binding affinity is observed with linear tetramines, which fulfil the stereochemical penchant of the metal of being four-coordinated, according to a square planar geometry [2]. Among linear tetramines, the greatest coordinating tendencies are shown by ligand 1 (N,N′-bis(-2-aminoethyl)-propane-1,3-diamine, currently named 2.3.2-tet, in view of the number of carbon atoms connecting the four amine groups), which possesses the right length to encompass the corners of the square [3]. Indeed, the crystal structure of the [CuII(1)](ClO4)2 complex salt indicates co-planar tetramine coordination, with the two perchlorate ions at a large distance along z axis [4].
We consider here the coordinating tendencies of the linear tetramine 2 (1,3-propane-bis-[1-methyl-4-piperazine]), which possesses the same 2.3.2 carbon skeleton as 1, but in which each link between middle and terminal nitrogen atoms is reinforced by a further ethylenic chain. Due to the presence of a methyl substituent on each terminal nitrogen atom, ligand 2 possesses four tertiary amine groups, a feature that is expected to reduce its coordinating tendencies towards metal ions. The [CuII(2)](ClO4)2 complex salt was isolated as a crystalline solid and its molecular structure was determined. The binding affinity of the [CuII(2)]2+ cation towards some selected inorganic anions in an MeCN solution was investigated and compared to that of the precursor complex [CuII(1)]2+.
2 Results and discussion
2.1 Complex formation in solution
Binding of CuII by the reinforced tetramine 2 cannot be investigated in water through conventional pH-metric techniques (which typically involve the titration with standard base of a solution containing equimolar amounts of ligand and metal salt, plus excess of strong acid). In fact, precipitation of copper(II) hydroxide precedes complexation, indicating very low stability of the [CuII(2)]2+ complex species. The low stability has to be ascribed to two distinct factors: (i) the presence of four tertiary amine nitrogen atoms; (ii) the presence of two piperazine rings in the ligand framework. In fact, in the uncomplexed ligand, the two piperazine subunits probably exist in the more stable chair conformation. When coordinating the metal ion, piperazine has to change to the less stable boat conformation, as shown by the crystallographic study (vide infra): the enthalpy of such a conformational change, in the gas phase, corresponds to 5.34 kcal mol–1 [5]. It has been shown that the aqueous CuII complex with the 3.2.3 tetramine containing a middle piperazine ring has a formation constant 9 log units lower than that of the corresponding complex with the plain 3.2.3 tetramine [6]. It is therefore reasonable that complexation by 2 is especially disfavoured and cannot compete with the hydroxide formation and precipitation. However, the [CuII(2)]2+ complex forms in boiling n-butanol (i.e. in strict absence of water), from which can be precipitated as perchlorate salt. The [CuII(2)](ClO4)2 salt dissolves in MeCN as a stable species, but, when put in water, it decomposes with formation of the bluish precipitate of Cu(OH)2.
2.2 The molecular structure of the [CuII(2)]2+ complex
On slow diffusion of diethyl ether in an acetonitrile solution of [CuII(2)](ClO4)2, dark blue crystals suitable for X-ray diffraction studies were obtained. The structure of the metal complex is shown as an ORTEP plot in Fig. 1. Selected bond lengths and angles are listed in Table 1.
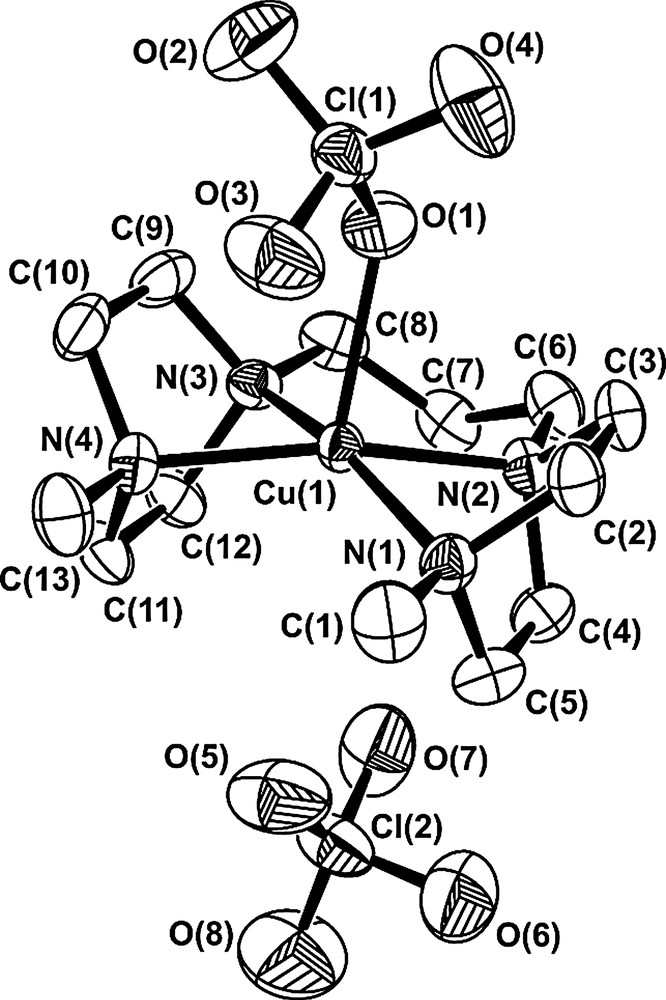
An ORTEP view of [CuII(2)(ClO4)]ClO4 (thermal ellipsoids are drawn at the 50% probability level, hydrogen atoms are omitted for clarity): the CuII centre shows a square-pyramidal coordination, both piperazine rings are in the boat conformation, and the [CuII(2)(ClO4)]1+ moiety exhibits CS symmetry (see text).
Selected bond lengths (Å) and angles (°) for [CuII(2)(ClO4)]ClO4
Cu(1)–N(1) | 2.051(4) |
Cu(1)–N(2) | 2.030(4) |
Cu(1)–N(3) | 2.026(4) |
Cu(1)–N(4) | 2.064(4) |
Cu(1)–O(1) | 2.355(4) |
N(1)–Cu(1)–N(2) | 73.83(15) |
N(1)–Cu(1)–N(3) | 163.12(15) |
N(1)–Cu(1)–N(4) | 110.34(15) |
N(2)–Cu(1)–N(3) | 97.71(15) |
N(2)–Cu(1)–N(4) | 163.81(14) |
N(3)–Cu(1)–N(4) | 73.93(15) |
O(1)–Cu(1)–N(1) | 99.17(15) |
O(1)–Cu(1)–N(2) | 94.69(14) |
O(1)–Cu(1)–N(3) | 96.03(14) |
O(1)–Cu(1)–N(4) | 99.91(14) |
The CuII centre shows a square-pyramidal coordination geometry, with the oxygen atom of a perchlorate group occupying the axial position: the metal centre lies above the N(1)–N(2)–N(3)–N(4) mean plane at a distance of 0.271(2) Å. The deviations of the four nitrogen atoms from the basal plane range between 0.005(2) and 0.006(2) Å. The Cu–N bond distances show values typically observed in copper(II) polyamine complexes: the distances to N(1) and N(4) atoms (bearing the terminal methyl groups) are only slightly longer than those observed to the middle nitrogen atoms N(2) and N(3), which are connected through a –CH2CH2CH2– chain. On the other hand, the axial Cu–O distance is especially short: this value is among the smallest observed for CuII complexes containing coordinated perchlorate anions and unequivocally characterises CuIIN4O square-pyramidal geometries [7]. It has to be noted that in the parent CuII(1)(ClO4)2 complex salt, the copper centre shows an octahedrally distorted coordination geometry. In particular, the metal is co-planar with the basal nitrogen atoms (the deviation from the N4 best plane being 0.03 Å) and the two axial Cu–O distances are 2.667 and 2.527 Å, i.e. in the range expected for octahedrally elongated copper(II) complexes. Thus, steric effects related to the presence of the piperazine rings seem to prevent the achievement of the most favoured co-planar coordination by the four amine nitrogen atoms in the [CuII(2)(ClO4)]ClO4 complex and raise the copper centre above the N4 plane. It has to be noted that in the corresponding perchlorate complex salt with the N,N′,N″,N″′-tetramethyl-cyclam derivative (Me4cyclam), in spite of the presence of four tertiary amine nitrogen atoms, the CuII ion is co-planarly chelated by the macrocycle, according to an octahedrally elongated geometry: in particular, the axial Cu–O distances are both 2.72 Å [8]. In the [CuII(2)(ClO4)]+ complex, the two piperazine rings show very similar boat conformations, the puckering parameters being [9]: q2 = 0.870(4) Å, q3 = –0.013(5), Ф = –3.3(3)°, QT = 0.870(4) Å, θ = 90.8(3)°, for the ring containing N(1) and N(2) atoms; q2 = 0.872(5) Å, q3 = –0.006(5), Ф = 3.0(4)°, QT = 0.872(5) Å, θ = 90.4(3)° for the other ring. The deviations from the C(2)–C(3)–C(4)–C(5) best planes are 0.025(3) for all the four C atoms, 0.739(6) Å for N(1), and 0.765(6) Å for N(2); those respect of the C(9)–C(10)–C(11)–C(12) best plane are 0.022(3) for the four C atoms, 0.760(6) Å for N(3), and 0.747(6) for N(4). The N–N vertex–vertex distances are 2.451(6) Å [N(1)–N(2)] and 2.460(6) Å [N(3)–N(4)].
The presence of two piperazine rings with boat conformation strongly reduces the N(1)–Cu(1)–N(2) and the N(3)–Cu(1)–N(4) bond angles (73.83° and 73.93°, respectively). Note that in the CuII(1)(ClO4)2 complex, corresponding angles are 85.30° and 85.24°. The two piperazine boats also force the C(1), C(6), C(8), and C(13) atoms to lie in the N(1)–N(2)–N(3)–N(4) mean-plane (the deviations respect of the amine best plane are in the range 0.028(8)–0.051(7) Å). In this way, the [CuII(2)(ClO4)1]+ moiety shows CS symmetry because it results characterised by a non-crystallographic mirror plane normal to the N4 best plane and passing through the Cu(1), C(7), O(1), Cl(1), and O(3) atoms. This atomic arrangement, in which the two terminal methyl groups remain in the amine best plane, produces (probably due to steric effects) an anomalous increase of the N(1)–Cu(1)–N(4) bond angle with respect to the value observed in the CuII(1)(ClO4)2 complex (95.47°).
The hexa-atomic chelate ring adopts a chair conformation: N(2)–N(3)–C(6)–C(8) atoms are very co-planar [the deviations from the best plane are in the range 0.001(2)–0.002(3) Å], Cu(1) is placed at 0.309(6) Å above the best plane and C(7) 0.739(7) Å below.
2.3 Interaction with inorganic anions in MeCN solution
On dissolving the [CuII(2)(ClO4)]ClO4 complex salt in MeCN, a blue solution is obtained, whose absorption spectrum shows a band centred at 552 nm (ɛ = 430 M–1 cm–1). It is hypothesised that the spectrum pertains to the [CuII(2)(MeCN)]2+ chromophore, in which a solvent molecule has replaced the perchlorate ion in the axial position of the square pyramid, observed in the solid complex salt. In fact, ClO4– is a poorly coordinating anion, which cannot compete with the MeCN ligand, present in overwhelming amount in an acetonitrile solution. Moreover, addition even of a large excess of Bu4NClO4 to the solution did not induce any modification of the spectrum, thus confirming lack of metal complex–anion interaction. On the other hand, on addition of Bu4NCl, the solution changed its colour from violet to blue, while significant variations were observed in the absorption spectrum.
Fig. 2 displays the family of spectra obtained on titrating an MeCN solution 1.0 × 10–3M in [CuII(2)(ClO4)]ClO4 with a standard MeCN solution of Bu4NCl. In particular, on chloride addition, the intensity of the band at 552 nm decreases, while a new band forms and develops at 676 nm. A sharp isosbestic point, at 610 nm, indicates that two species are present at the equilibrium. Moreover, a plot of the absorbance at 676 nm vs. the added equivalent. of chloride shows a saturation profile, a plateau being reached after the addition of 1 equiv (limiting absorbance value: 380 M–1 cm–1). All the above evidences indicate that the following equilibrium is established in solution:(1)

Spectra recorded in the course of the titration of an MeCN solution of [CuII(2)(ClO4)]ClO4 with a standard solution of Bu4NCl. The inset shows the change of the absorbance at 680 nm on addition of chloride equivalents.
Data pertinent to the equilibrium: [CuII(2)(MeCN)]2+ + X– ⇌ [CuII(2)(X)]+ + MeCN, in an MeCN solution, at 25 °C. λmax and ɛ (limiting molar absorbance) refer to the [CuII(2)(X)]+ chromophore
X- | logK | λ (nm) | ɛ (m–1 cm–1) |
Cl– | 5.87 ± 0.07 | 676 | 380 |
Br– | 5.48 ± 0.03 | 670 | 400 |
I– | 5.18 ± 0.09 | 665 | 330 |
NCS– | 4.33 ± 0.03 | 638 | 390 |
NO3– | 0.97 ± 0.05 | 572 | 350 |
The observed sequence of anion affinity (Cl– > Br– > I– > NCS– > NO3–) does not parallel the sequence of the solvation terms. In fact, the smallest chloride ion, in view of the highest value of the charge/radius ration, should be highly solvated and the very endothermic desolvation term should disfavour its binding to CuII. On the contrary, binding affinity decreases with the decreasing anion charge density, q/r and solvation, Thus, binding affinity has to be rather related to another energy term, for instance the intensity of the metal–ligand (CuII–X) interaction. In particular, it is observed that anion binding to the [CuII(2)(MeCN)]2+ complex induces a different red-shift of the d–d absorption band: the magnitude of the shift decreases along the series: Cl– > Br– > I– > NCS– > NO3–, thus paralleling the logK sequence.
In particular, logK and Δν are linearly correlated, as shown in the plot in Fig. 3 (Δν is the difference of νmax values of the [CuII(2)(MeCN)]2+ and [CuII(2)(X)]+ complexes). The broad bands observed for the two square-pyramidal complexes [CuII(2)(MeCN)]2+ and [CuII(2)(X)]+ are the envelope of three transitions: z2 → xz, yz; z2 → x2 – y2; z2 → xy [11,12]. In particular, one could assume that the maximum of the observed band coincides with the energy of the middle transition z2 → x2 – y2, which is related to the energy of the axial CuII–X interaction. Linear correlation indicates that log K strongly depends upon the energy of the metal–anion coordinative bond, while the observed sequence characterises the donor tendencies of X– as an apical ligand in a square-pyramidal complex.

Plot of logK values for the anion binding equilibrium to [CuII(2)]2+ (filled triangles) and to to [CuII(1)]2+ (open triangles) vs. Δν. Δν = νmax([CuII(L)(X)]+) – νmax([CuII(L)]2+), L = 1, 2.
For comparative purposes, we wanted to investigate the anion binding tendencies of the corresponding copper(II) complex with the parent tetramine 2.3.2-tet, 1. Thus a red–violet MeCN solution of the [CuII(1)](ClO4)2 complex salt was titrated with the same tetrabutylammonium salts as [CuII(2)(ClO4)]ClO4. On chloride addition the colour of the solution turned blue. Fig. 4 shows the family of spectra taken during the titration. It is seen that the band of the [CuII(1)]2+ complex, centred at 525 nm (ɛ = 100 M–1 cm–1), decreases, while a new band forms and develops at 584 nm, to reach a limiting value of molar absorbance ɛ = 140 M–1 cm–1 after the addition of 1 equiv. of chloride. The titration profile is shown in the inset.

Spectra recorded in the course of the titration of an MeCN solution of [CuII(1)](ClO4)2 with a standard solution of Bu4NCl. The inset shows the change of the absorbance at 548 nm on addition of chloride equivalents.
Also in the present case, it is suggested the occurrence of an association equilibrium, as represented below:(2)
Similar behaviour was observed on titration with bromide, iodide and thiocyanate salts. The association constants, calculated through non-linear least-squares fitting of the spectrophotometric titration profiles, are reported in Table 3, together with λmax and ε values of the [CuII(1)(X)]+ complex.
Data pertinent to the equilibrium: [CuII(1)]2+ + X– ⇌ [CuII(2)(X)]+, in an MeCN solution, at 25 °C. λmax and ɛ (limiting molar absorbance) refer to the [CuII(1)(X)]+ chromophore
X– | logK | λ (nm) | ɛ (m–1 cm–1) |
Cl– | 5.08 ± 0.02 | 584 | 140 |
Br– | 4.28 ± 0.04 | 564 | 130 |
I– | 3.41 ± 0.01 | 548 | 81 |
NCS– | 3.33 ± 0.01 | 570 | 99 |
On the other hand, even addition of a large excess of [Bu4N]NO3 did not cause any spectral modification, indicating no anion interaction with the copper(II) tetramine complex. In Fig. 3, log K values are plotted vs. corresponding Δν values (open triangles). It is seen that halide ions fit quite well a straight line parallel to that observed in the case of the reinforced tetramine complexes, while NCS– does not correlate. We could tentatively assume that the ‘bad' behaviour of the ambidentate thiocyanate ligand is related to its binding mode, which may differ when moving from complex [CuII(2)(NCS)]+ to [CuII(1)(NCS)]+.
Comparison of data in Fig. 3 suggests some considerations: (i) the energy of the axial coordinative interaction is the most important term determining the selective affinity of [CuII(2)]2+ and [CuII(1)]2+ complexes towards anions; (ii) axial binding seems stronger in the [CuII(2)]2+ complex, which is pre-organised to five-coordination, as shown by the larger values of Δν. However, the [CuII(1)]2+ complex is able to discriminate halide anions much better than the [CuII(2)]2+ complex: this results by the significant difference of Δν values, which reflects the varying intensity of the axial interaction; (iii) the ‘straight line' of complex [CuII(1)(X)]+ (for halides) lies ca. 2 log units above the straight line of the [CuII(2)(X)]+ complex: the corresponding energy (ca. 3 kcal mol–1) should reflect the steric repulsive effects which arise on anion coordination to the reinforced tetramine complex and which do not operate in the case of the complex with the plain tetramine 1.
3 Conclusions
There exists a current interest on anion recognition and sensing, in view of the relevant role anions play in environmental sciences and biomedicine [13]. In most cases, recognition is based on electrostatic interactions, which are not especially strong and are adirectional. Coordinatively unsaturated metal complex can behave as more efficient receptors for anions, due to the higher intensity of the metal–ligand interactions and to their defined directionality. A convincing example in this sense has been shown by this investigation. In particular, we have demonstrated that a copper(II) tetramine complex can be a good receptor for inorganic anions, which give five-coordinated species. Anion binding tendencies can be enhanced by preorienting the metal centre to a square-pyramidal geometry. This goal has been achieved through the used of a sterically hindered tetramine like 2.
4 Experimental section
4.1 1,1′-(1,3-Propandiyl)bis-(4-methyl-piperazine) (2)
To a stirred solution of 1-methylpiperazine (5.00 g, 49.89 mmol) in acetonitrile (40 ml), K2CO3 (6.9 g, 49.9 mmol) and KI (in catalytic amount) were added. The solution was stirred at 60 °C and a solution of 1,3-dibromopropane (5.14 g, 25.84 mmol) in acetonitrile (10 ml) was added. The resulting mixture was stirred at 60 °C for 24 h and then the solvent was removed under vacum. The crude product was purified by liquid chromatography (Al2O3, CH2Cl2/MeOH/NH3 50:9:1, Rf = 0.25); yield 81%. MS (ESI): m/z 241.4 [M+H]+.
4.2 [Cu(2)](ClO4)2
A solution of CuII(ClO4)2·6H2O (2.5 mmol, 0.926 g) in butanol was added to a solution of the ligand 2 (2.5 mmol, 0.601 g) in butanol. The mixture was refluxed for 2 h, and a violet precipitate formed. The solid was filtered, washed with cold methanol and dried (94% yield). Diffusion of diethyl ether into the mother liquor gave crystals suitable for X-ray crystallography. Elemental analysis: Calculated for C13H28N4Cl2CuO8: C, 31.05%, H, 5.61%, N, 11.14%. Found: C, 31.90%, H, 5.58%, N, 11.21%.
4.3 Crystal-structure analysis
An irregular dark-blue fragment of ~ 0.75 × 0.60 × 0.50 mm3 was used for single-crystal X-ray diffraction study. Intensity data were collected at ambient temperature on a Enraf-Nonius four-circle diffractometer, working with graphite-monochromatised MoKα X-radiation (λ 0.71073 Å), in the 2θ range 4–52°. Crystal data for C13H28Cl2CuN4O8 are: M = 502.84, monoclinic P21/c (no. 14), a = 8.767 (6) Å, b = 15.798(2) Å, c = 15.179(6) Å, β = 109.68(3)°, V = 1979.5(16) Å3, Z = 4, ρcalc = 1.687 g cm–3, μ = 1.42 mm–1.
Data reduction (including intensity integration, background, Lorentz and polarisation corrections) was performed using the WinGX package [14]. Absorption effects were evaluated with the psi-scan method [15] and absorption correction was applied to the data (0.394 and 0.489 min. and max. transmission factors).
Crystal structure was solved by direct methods (SIR 97) [16] and refined by full-matrix least-square procedures on F2 using all reflections (SHELXL 97) [17]. All non-hydrogen atoms were refined with anisotropic temperature factors; hydrogens were placed at calculated positions using the appropriate AFIX instructions and refined using a riding model. Refinement of 253 parameters converged to final R values of 0.0521 for 3172 intense reflections [IO > 2 σ(IO)] and 0.0660 for all 3867 data. The weighted final wR2 values were 0.1398 for intense reflections and 0.1498 for all data (GOF = 1.062).
5 Supplementary material available
Crystal structure details have been deposited at the Cambridge Crystallographic Data Centre, 12 Union Road, Cambridge CB2 1EZ, UK, and allocated the deposition number CCDC 237872. Data can be obtained by contacting the CCDC.
Acknowledgements
The financial support of the Italian Ministry of University and Research (PRIN 2003 ‘Dispositivi Supramolecolari'; FIRB–Contract RBNE019H9K_002) is gratefully acknowledged.