1 Introduction
The solvating [1] and ligating [2–6] properties peculiar to dimethylsulphoxide (DMSO), (CH3)2SO, have been exploited in very diverse ways but one of the simple attractions of its use has been the ease with which its usually readily crystallised coordination complexes may be prepared for subsequent application as a source of anhydrous metal ions. The uranyl ion complex [UO2(DMSO)5](ClO4)2 [7], for example, has been a useful reagent in the preparation of uranyl ion complexes with calixarenes [8,9], some of which are sensitive to water [10]. Though the [UO2(DMSO)5]2+ cation could be said to be a stereotypical (pentagonal bipyramidal) complex of U(VI), given the poor anion-solvating ability of DMSO [1] and the ready observation of perchlorato complex formation by some very heavy metals [11], the question arises as to why DMSO is able to exclude perchlorate (in [UO2(DMSO)5](ClO4)2) from the primary coordination sphere of the uranyl entity, a question relevant to a considerable body of recent work [12] concerned with the relation between solution and solid state structures of solvated metal cations and rendered significant by the recent observation of new hydrates of uranyl perchlorate in which perchlorate coordination does occur [13]. In attempting to understand this by structural studies of DMSO solvates of a range of relatively simple uranyl compounds, we have exposed a number of subtleties in the coordination chemistry of uranyl ion open to influence by DMSO and report these results herein. Our structure determination for [UO2(DMSO)2(NO3)2] was conducted in ignorance of the recently published structure (293 K) [14] for this complex but we include the results of our more precise low temperature (153 K) determination herein for the purposes of comparisons with the other structures reported1.
2 Experimental
2.1 Synthesis
2.1.1 [UO2(DMSO)5](CF3SO3)2
Excess CF3SO3H (0.5 ml) was added to a slurry of [UO2(O2CCH3)2(OH2)2] (‘uranyl acetate dihydrate’, 0.42 g) in water (20 ml) and the solution formed evaporated to dryness on a steam bath. The glassy residue was dissolved in ethanol (20 ml) and DMSO (2 ml) added, causing the yellow colour of the solution to intensify markedly. Within minutes, long, yellow needles of the product (as used for the structure determination) commenced to precipitate. After 24 h, these were collected by filtration and washed with ethanol and ether. Yield: 0.75 g. Analysis: calcd for C12H30F6O13S7U: C, 15.03; H, 3.15; S, 23.41; found: C, 15.1; H, 3.1; S, 23.3%.
2.1.2 [UO2(DMSO)2(O2NO)2]
[UO2(OH2)2(O2NO)2]·4 H2O (uranyl nitrate hexahydrate, 0.50 g) was dissolved in DMSO (2 ml) by gentle warming and ethanol (20 ml) then added to give initially a clear solution that soon began to deposit a crystalline, pale yellow precipitate, which was collected after 15 min and washed with diethyl ether (0.48 g). This material was recrystallised from DMSO (1 ml) by the addition of ethanol (20 ml) before crystals as used for the structure determination were obtained by vapour diffusion of ethanol into its solution in DMSO. Analysis: calcd for C4H12N2O10S2U, C, 8.73; H. 2.20; N. 5.09; S, 11.65; found: C, 8.8; H, 2.2; N, 5.0; S, 11.7%.
2.1.3 [UO2(DMSO)3(pic)2]
Freshly-precipitated ‘uranyl hydroxide’, prepared by mixing aqueous uranyl nitrate (1.04 g in 20 ml) with a twofold molar quantity of aqueous sodium hydroxide (0.16 g in 10 ml), was collected by filtration, washed with water, then dissolved on the filter by slurrying with a slight excess (1.10 g) of picric acid (Hpic) and water (5 ml). The solution formed was filtered, evaporated to dryness under vacuum and the residue extracted (3 × 10 ml) with dichloromethane to remove any excess acid present. The yellow solid remaining was dissolved in DMSO and the complex rapidly precipitated once (yield 1.20 g) by the addition of ethanol to ensure displacement of water before recrystallisation was conducted from a hot (90 °C) mixture of DMSO (5 ml) and ethanol (100 ml) by allowing the solution to cool slowly to room temperature. The crystals initially deposited were in the form of extremely fine needles but, on extended standing (~1 yr) of the mixture, some crystals of a size and morphology useful for structure determination were apparent. Analysis: calcd for C18H22N6O19S3U, C, 22.51; H, 2.31; N, 8.75; S, 10.01; found: C, 22.6; H, 2.4; N, 8.4; S, 9.8%.
2.1.4 [(UO2)4(μ3-O)2(DMSO)4(O2CCH3)4]
A saturated solution of [UO2(O2CCH3)2(OH2)2] in DMSO at 100 °C was filtered and allowed stand at room temperature for several weeks as clusters of yellow crystals suitable for structure determination slowly deposited. Analysis: calcd for C16H36O22S4U4, C, 11.57; H, 2.18; S, 7.72; found: C, 11.9; H, 2.1; S, 7.8%.
2.2 Structure determinations
Full spheres of CCD area-detector data were measured at ca 153 K (Bruker AXS instrument, ω scans; monochromatic Mo Kα radiation, λ = 0.71073 Å) yielding Ntotal reflections, reducing to N unique (Rint quoted) after ‘empirical’/multiscan absorption correction (proprietary software), No with F > 4σ(F) being considered ‘observed’ and used in the full matrix least-squares refinement (anisotropic displacement parameter forms for the non-hydrogen atoms; (x, y, z, Uiso)H constrained at estimated values). Conventional residuals R, Rw (weights: (σ2(F) + 0.0004 F2)–1) are quoted on |F| at convergence. Neutral atom complex scattering factors were employed within the context of the Xtal 3.7 program system [15]. Pertinent results are given in Tables 1–6 and in the figures, the latter showing 50% probability amplitude displacement ellipsoids for the non-hydrogen atoms, hydrogen atoms having arbitrary radii of 0.1 Å. In [UO2(DMSO)5](CF3SO3)2, the sulphur and oxygen atoms of DMSO 5 were modelled as disordered over two sets of sites, seemingly in concert with disorder in the axial OUO array, the second component of the latter being slightly displaced (U…U′ 0.402(2) Å) and tilted (the angle between the two OUO component lines being 12.3°), and with the SO3 component of anion 2, all components being held at occupancies 0.5 after trial refinement. While these features raise questions of superlattice, suspect space group, twinning, etc., undetected after systematic re-examination (apart from certain diffuse scattering manifestations), we note that the remainder of the structure, containing the majority of the DMSO groups and the other anion, all notoriously prone to disorder, refines smoothly and unproblematically. Friedel data were retained distinct, xabs refining to 0.332(6).
Crystal and refinement data
Compound | 1 | 2 | 3 | 4 |
Chemical formula | C12H30F6O13S7U | C4H12N2O10S2U | C18H22N6O19S3U | C16H36O22S4U4 |
Formula mass | 958.8 | 550.3 | 960.6 | 1660.8 |
Crystal system | monoclinic | monoclinic | triclinic | monoclinic |
Space group | C2 (#5) | C2/c (#15) | P (#2) | C2/c (#15) |
a (Å) | 24.106(2) | 17.731(2) | 7.7051(8) | 18.686(3) |
b (Å) | 6.3745(7) | 5.8756(6) | 12.219(1) | 10.653(2) |
c (Å) | 23.482(2) | 15.332(1) | 16.573(2) | 18.776(3) |
α (°) | 90 | 90 | 78.295(2) | 90 |
β (°) | 119.695(2) | 116.797(2) | 79.891(2) | 96.539(4) |
γ (°) | 90 | 90 | 87.687(2) | 90 |
V (Å3) | 3134 | 1397 | 1504 | 3713 |
Z | 4 | 4 | 2 | 4 |
Dcalc (g cm–3) | 2.031 | 2.616 | 2.121 | 2.970 |
μ (Mo Kα) (mm–1) | 5.7 | 12.0 | 5.7 | 17.7 |
Crystal size (mm) | 0.18 × 0.12 × 0.09 | 0.37 × 0.11 × 0.05 | 0.40 × 0.37 × 0.24 | 0.12 × 0.11 × 0.08 |
Tmin/max | 0.74 | 0.45 | 0.33 | 0.67 |
2θmax (°) | 75 | 75 | 75 | 65 |
Nt | 31 615 | 13 930 | 29 591 | 26 147 |
N (Rint) | 8333 (0.029) | 3608 (0.047) | 15 354 (0.044) | 6554 (0.058) |
No | 6891 | 2660 | 13 311 | 5367 |
R | 0.041 | 0.036 | 0.041 | 0.030 |
Rw | 0.060 | 0.063 | 0.051 | 0.044 |
The uranium environments. [UO2(DMSO–O)5](F3CSO3)2, 1. The r values (Å) are the U–O distances; other entries in the matrix are the angles (degrees) subtended at the uranium by the relevant atoms at the head of the row and column. The upper entry is the environment for the array containing UO2 and O(5), the lower for the disordered (UO2′) and O(5′) component
Atom | r | O(12/12′) | O(1) | O(2) | O(3) | O(4) | O(5/5′) |
O(11) | 1.75(2) | 178.0(8) | 82.8(7) | 92.2(7) | 90.6(6) | 81.7(8) | 90.7(7) |
O(11′) | 1.77(2) | 178.6(8) | 95.8(5) | 92.9(6) | 89.6(4) | 92.6(6) | 89.1(5) |
O(12) | 1.78(2) | 99.0(7) | 87.5(6) | 87.4(5) | 97.3(7) | 90.8(6) | |
O(12′) | 1.77(2) | 84.9(5) | 88.4(6) | 90.5(4) | 86.2(6) | 90.0(5) | |
O(1) | 2.416(7) | 69.0(3) | 137.0(3) | 147.4(3) | 76.4(4) | ||
2.384(7) | 72.1(3) | 144.7(3) | 142.7(3) | 71.7(4) | |||
O(2) | 2.477(8) | 68.9(2) | 139.9(2) | 144.6(4) | |||
2.327(8) | 72.8(2) | 143.8(2) | 140.1(4) | ||||
O(3) | 2.452(5) | 71.6(2) | 146.4(4) | ||||
2.370(5) | 71.5(2) | 143.4(4) | |||||
O(4) | 2.322(8) | 75.3(4) | |||||
2.417(8) | 72.1(4) | ||||||
O(5) | 2.322(13) | ||||||
O(5′) | 2.377(11) |
The uranium environments. [UO2(DMSO-O)2(O2NO)2], 2. Presentation as in Table 2. Primed atoms are inversion related
Atom | r | O(01) | O(02) | O(1) | O(11′) | O(01′) | O(02′) | O(1′) |
O(11) | 1.771(4) | 91.5(2) | 87.6(2) | 88.7(2) | 180(-) | 88.5(2) | (92.4(2)) | 91.3(2) |
O(01) | 2.524(4) | 50.3(1) | 65.2(1) | 88.5(2) | 180(-) | 129.7(1) | 114.8(1) | |
O(02) | 2.534(5) | 115.2(1) | (92.4(2)) | (129.7(1)) | 180(–) | 64.8(1) | ||
O(1) | 2.355(3) | (91.3(2)) | (114.8(1)) | (64.8(1)) | 180(–) |
The uranium environments. [UO2(DMSO-O)3](picrate-O)2, 3. Presentation as in Table 2
Atom | r | O(2) | O(10) | O(20) | O(30) | O(11) | O(21) |
O(1) | 1.774(5) | 177.0(1) | 87.3(1) | 93.9(1) | 91.1(1) | 96.5(1) | 91.8(1) |
O(2) | 1.771(2) | 90.8(1) | 83.3(1) | 91.9(1) | 85.1(1) | 88.5(1) | |
O(10) | 2.356(3) | 72.82(9) | 140.24(9) | 70.20(9) | 146.18(9) | ||
O(20) | 2.419(2) | 146.83(10) | 140.93(9) | 73.52(9) | |||
O(3) | 2.376(3) | 70.55(10) | 73.56(9) | ||||
O(11) | 2.386(3) | 143.25(9) | |||||
O(21) | 2.357(3) |
Selected geometries, [(UO2)4(μ3-O)2(DMSO)4(CH3CO2)4], 4. Primed atoms are inversion related. The uranium environments; presentation as in Table 2
(i) U(1) | |||||||
Atom | r | O(12) | O(0) | O(0′) | O(1) | O(101) | O(201) |
O(11) | 1.785(4) | 176.1(2) | 88.5(2) | 94.3(2) | 90.7(2) | 88.6(2) | 91.2(2) |
O(12) | 1.784(4) | 93.7(2) | 89.5(2) | 85.8(2) | 91.9(2) | 85.9(2) | |
O(0) | 2.249(4) | 71.7(1) | 151.9(2) | 138.9(1) | 81.9(2) | ||
O(0′) | 2.306(4) | 136.3(2) | 67.7(1) | 152.9(1) | |||
O(1) | 2.481(5) | 69.1(2) | 70.0(2) | ||||
O(101) | 2.467(5) | 139.1(2) | |||||
O(201) | 2.405(4) | ||||||
(ii) U(2) | |||||||
Atom | r | O(22) | O(0) | O(2) | O(202) | O(101′) | O(102′) |
O(21) | 1.785(4) | 175.5(2) | 94.8(2) | 88.6(2) | 89.8(2) | 89.3(2) | 91.1(2) |
O(22) | 1.784(4) | 89.7(2) | 87.1(2) | 90.3(2) | 92.5(2) | 86.8(2) | |
O(0) | 2.216(4) | 165.6(2) | 88.1(2) | 68.5(2) | 119.5(2) | ||
O(2) | 2.390(4) | 77.9(2) | 125.6(2) | 74.3(2) | |||
O(202) | 2.328(5) | 156.4(2) | 152.2(2) | ||||
O(101′) | 2.494(4) | 51.4(2) | |||||
O(102′) | 2.527(5) |
Further geometries, [(UO2)4(μ3-O)2(DMSO)4(CH3CO2)4], 4. Primed atoms are inversion related
Atoms | Parameter | Atoms | Parameter |
Distances (Å) | |||
U(1)···U(2) | 4.0967(6) | U(1)···U(1′) | 3.6917(6) |
U(1)···U(2′) | 3.8534(6) | O(0)···O(0′) | 2.670(6) |
C(101)–O(101) | 1.290(8) | O(101)···O(0′) | 2.661(6) |
C(101)–O(102) | 1.240(8) | C(201)–O(201) | 1.273(8) |
C(201)–O202) | 1.273(8) | ||
Angles (degrees) | |||
U(1)–O(1)–S(1) | 130.3(3) | U(2)–O(2)–S(2) | 121.9(2) |
U(1)–O(0)–U(1′) | 108.3(2) | U(1)–O(101)–U(2′) | 101.9(2) |
U(1)–O(0)–U(2) | 113.1(2) | U(1)–O(101)–C(101 | 162.7(4) |
U(2)–O(0)–U(1′) | 116.9(2) | C(101)–O(101)–U(2′) | 94.7(4) |
U(1)–O(201)–C(201) | 119.0(6) | C(101–O(102)–U(2′) | 94.5(4) |
U(2)–O(202)–C(201) | 126.7(4) | O(201)–C(201)–O(202) | 121.8(5) |
O(101)–C(101)–C(102) | 119.6(6) |
The crystallographic data, in the form of .cif files, have been deposited with the CCDC, deposition numbers 225235–225238. These data may be obtained free of charge via www.ccdc.cam.ac.uk/conts/retrieving.html or from the Cambridge Crystallographic Data Centre, 12 Union Road, Cambridge CB2 1EZ, UK; fax.: (+44) 1223-336-033 or deposit@ccdc.cam.ac.uk.
3 Results and discussion
The trifluoromethanesulphonate, [CF3SO3]–, ‘triflate’, anion is generally considered to be a weakly coordinating ligand [16], though one of greater basicity than perchlorate, [ClO4]– [17], so that it was of interest to determine if the DMSO solvate of uranyl triflate would differ significantly in structure from that of uranyl perchlorate [7]. The hydrated triflate is known to contain [UO2(OH2)5]2+ [18], as do two of the hydrated perchlorates [13], but consideration of its structure is complicated by the presence of a strongly H-bonding crown ether as part of the lattice array. In other circumstances, coordination of triflate to uranium is certainly well-established [19]. In fact, structural characterisation of the DMSO solvate of uranyl trifluoromethanesulphonate (Fig. 1) shows it to be a penta-solvate, with all solvent molecules coordinated through their oxygen atoms to the UO2 moiety, [UO2(DMSO-O)5](F3CSO3)2, the immediate environment of the U(VI) centre being very closely similar to that in the perchlorate counterpart, [UO2(DMSO-O)5](ClO4)2 [7]. In both cases, the array of seven oxygen atoms about the central metal is pentagonal bipyramidal, the uranyl oxygen atoms axial and those of DMSO equatorial. Regrettably, the present more modern determination offers enhancement in precision of only limited credibility over that of the perchlorate in respect of the uranium environment, difficulties in the determination, ascribed to disorder as above, degrading the precision in that respect. (It is of interest to note that both structures presented difficulty in solution by virtue of the uranium disposition, that of the perchlorate being located at (1/2, 0.046, 1/4) in a Cc cell, that of the present divided over (0.499, 1/2, 0.76; 0.499, 0.44, 0.77)). In the cation of the perchlorate, U–O(uranyl) are 1.70(3), 1.81(4), U–O(DMSO) 2.33(4)–2.43(2) (< > 2.38(4) Å), in the present (triflate), (< >) U–O(uranyl) are 1.77(1) and U–O(DMSO) 2.39(5) Å. About U, U′ the O(1-4) component defines a good plane (χ2 11) with deviations of U,U′,O(5),O(5′) being –0.231(3), 0.142(3), –0.59(2), 0.29(2) Å. (Significant anisotropy in the ellipsoids of O(1,5), possibly encompassing unresolved disorder, is noted.) In both compounds, as found generally for O-bound complexes of DMSO [1,2,20], the geometry of bound DMSO differs from that of the ‘free’ molecule, with the bond angle changes (Tables 2–4) indicating an approach towards a more nearly tetrahedral array about S. If this is interpreted as meaning that the charge distribution in the ligand is close to that defined by the octet form of DMSO, where S has a formal positive charge and O a negative, then some tendency of the S of bound DMSO to act as a site for interaction with negative moieties, including the oxygen atom of another DMSO (as in the dipole–dipole interactions of the pure solvent), might be anticipated. Indeed, evidence that the S of O-coordinated DMSO is involved in at least two weak interactions, in addition to its three ‘obvious’ bonding interactions, can be found in a wide variety of crystal structures [21], including that of the tetrafluoroborate of [UO2(DMSO-O)5]2+ [22], as well as those of both the perchlorate and triflate. Disorder in the last two structures leads to considerable uncertainty in some interionic contacts but in all three salts there is at least one intramolecular S···O (of adjacent DMSO or uranyl-O) in the range 2.9–3.2 Å for all S. As well, in the tetrafluoroborate there are S···F(BF3–) contacts ~3.3 Å and in the perchlorate, S···O(ClO3) approaches as short as 3.16(1) Å, while in the triflate, there are S···F(F2CSO3) approaches as short as 3.54(1) Å and S···O(O2SCF3) approaches as short as 2.93(3) Å. In addition, some C atoms of DMSO methyl groups lie within 3.34(1) Å of perchlorate-O and within 2.93(5) and 3.27(2) Å of triflate-O and -F, respectively, indicating more than one specific mechanism for association between cation and anion in these complexes. Interestingly, one of the uranyl-O atoms in the perchlorate lies at 3.7 ± 0.1 Å of all five DMSO-S atoms (while the other is at 4.1±0.1 Å) and in the triflate one uranyl-O lies at 3.6 ± 0.3 from four DMSO-S and at 4.45(2) Å from the fifth, which, however, is at 3.45(2) Å from the other uranyl-O. This is perhaps a factor favouring both O-coordination to U and the binding of DMSO in preference to the anions. There is evidence in very different contexts [23,24] for the unrecognised importance of uranyl-O basicity in determining the properties of U(VI) compounds and it may be noted also that uranyl-O in [UO2(OH2)5]2+ lie within H-bonding distances of the water ligands. In this particular case, however, it has recently been shown [13] that the presence or absence of perchlorate in the primary coordination sphere of U(VI) in various hydrated uranyl perchlorates seems to depend largely on the composition of the solution from which the crystals deposit, so that if there is no marked preference for water over what is conventionally regarded as a very weak ligand [17], a special interaction with uranyl-O cannot be considered dominant and perhaps angular factors minimise any H-bonding interaction of coordinated water and uranyl-O.
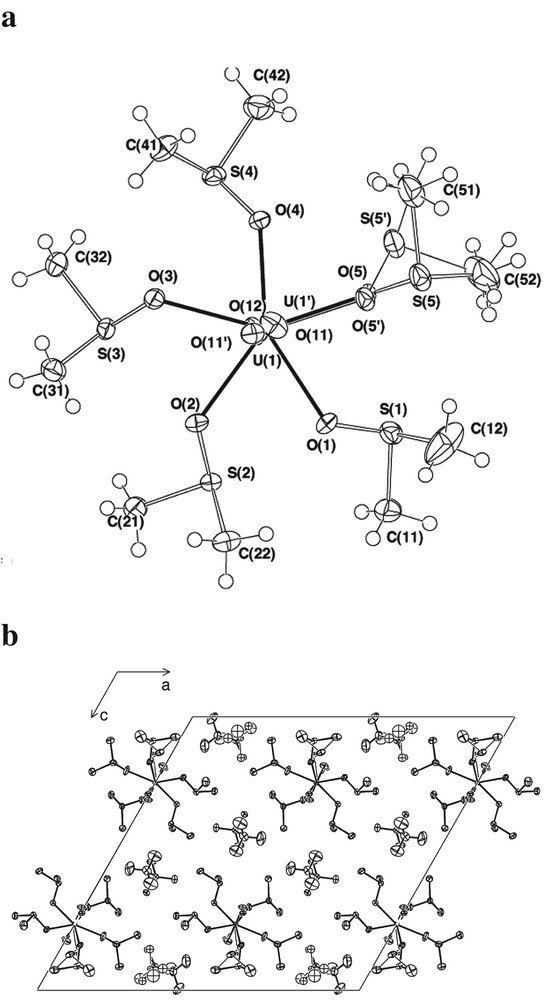
(a) The pentagonal bipyramidal [UO2(DMSO)5]2+ cation projected down the UO2 axis, showing the disordered components of the UO2 array and DMSO 5. (b) Unit-cell contents projected down b, showing the positioning of the counteranions and the regions of order and disorder in the cell.
Although the 2,4,6-trinitrophenoxide (picrate = pic–) anion has sometimes been described as a ‘weak’ ligand [25], there is substantial evidence to the contrary [26] and the present studies show that, in a crystalline complex, it is at least comparable to nitrate in its ability to bind to U(VI) in the presence of DMSO. Thus, in the DMSO solvates of both uranyl nitrate and picrate (Fig. 2), the two anions are coordinated, so that the lattices can be considered to be made up solely of neutral molecules. In the case of the nitrate, the anion is bound as a symmetrical chelate, and in the picrate as a unidentate, bound through the (formally) phenoxide oxygen atom. The nitrate crystallises with one half of the formula unit [UO2(DMSO-O)2(O2NO)2] comprising the asymmetric unit of the structure. The uranium is disposed on a crystallographic inversion centre, and with a coordination number of eight, cf. seven in the triflate and picrate, by virtue of the small bite of the nitrate, though if, as commonly appears justified [27], symmetrically chelating nitrate is considered to occupy a single ‘normal’ coordination site, the U atom can be considered to have octahedral six-coordination. The uranium lies 0.066(9) Å out of the plane of the nitrate, which has a dihedral angle of 4.5(2)° to the O6 equatorial plane that contains (necessarily) the uranium atom. The DMSO-S atom here is only 3.333(4) Å from uranyl-O and even closer (3.251(4) Å) to one of the chelating nitrate donor oxygen atoms. Such intramolecular contacts are accompanied by intermolecular contacts also involving the nitrate units. Thus, there are pairwise interactions between [UO2(DMSO-O)2(O2NO)2] entities that involve a uranyl oxygen atom being only 3.137(6) Å from the nitrate-N of an adjacent molecule, a DMSO methyl-C approach to within 3.34(1) Å of a coordinated nitrate-O and another within 3.243(8) Å of the uncoordinated O-atom of a nitrate ligand. Again, DMSO appears to have a specific role in maintaining the complete lattice array as well as in simply satisfying the coordination requirements of U(VI).
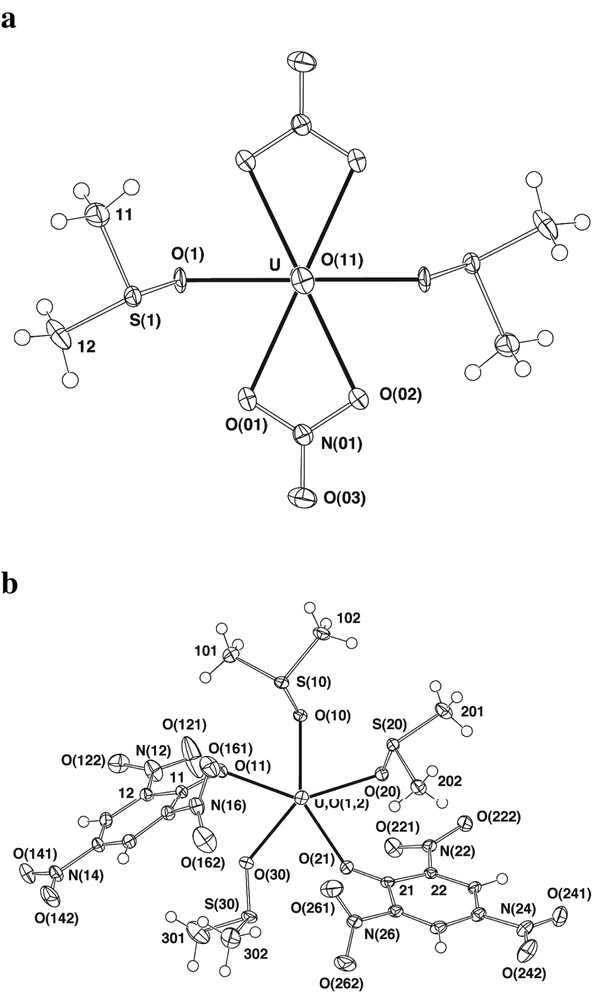
The ‘molecular’ units, projected down their O–U–O axes, found within the lattices of: (a) 2, [UO2(DMSO)2(O2NO)2] and (b) 3, [UO2(DMSO)3(pic)2].
Although a ligand of remarkable versatility [26], picrate anion is formally a member of the class of aryloxides and, structurally, uranium(VI) complexes of aryloxide ligands are somewhat better characterised in the particular case of calixarenes [9,28,29] than they are for simple, unidentate aryloxides [30], though this is a situation under redress [23]. A common feature of the currently known chemistry in both domains appears to be the frequent detection of complexes involving binding of the aryloxide donors to oligonuclear uranium clusters which can be regarded as arising from uranyl (UO22+) entities linked, most commonly, via bridging by way of equatorial donor atoms. There are, however, instances where the uranyl oxo (axial) atoms act as the bridge [9,23,28,29,31]. This can be seen as an indication of significant basicity at these sites [23,24], perhaps interpretable also as an indication of the weight to be given to the representation U+–O–, rather than U=O, of the uranyl-U–oxygen bond. The evidence discussed above for close interatomic approaches involving uranyl-O in the DMSO solvates of both uranyl triflate and nitrate is indicative that this apparent basicity is not some peculiar facet of U(VI)/O/U(VI) interactions, so that it is unsurprising to find further evidence for it in the structure of the DMSO solvate of uranyl picrate.
Structural studies of metal picrates are extensive [26,32] and a significant feature of the lattice array in all, as for picrates generally, is the occurrence of picrate ‘stacking’. Although this stacking involves various forms of projection of one picrate unit upon another, in a global sense it can be understood in terms of optimising the approaches, typically to within ~3.5 Å, of centres of relatively positive charge in one picrate to centres of relatively negative charge on another [33,34]. More specifically, it is often seen to involve the close approach of a nitro-group oxygen atom of one picrate to the nitro-group nitrogen atom of another. This suggests that the interaction may be at least partly related to the balance between three- and four-coordination of N(V) when bound to oxygen, a balance which usually lies in favour of three-coordination, though one which can be displaced, at least in the solid state, as seen in the structural characterisation of ‘orthonitrate’, [NO4]3– [35]. Thus, the question arises (though this was really not foreseen prior to the present structure determination) as to whether the basicity of uranyl-O and the acidity of picrate-N might lead to a specific interaction when these two entities are combined.
The structure solution obtained for [UO2(pic)2(DMSO)3] shows it to have several features which might have been anticipated from prior knowledge of uranyl and picrate compounds generally. A full formula unit (Fig. 2), devoid of crystallographic symmetry comprises the asymmetric unit of the structure and contains uranium in a pentagonal bipyramidal environment with an essentially linear OUO (uranyl) unit of ‘normal’ dimensions (U–O 1.773(1) Å; O–U–O 177.0(1)°) and, in the ‘equatorial’ plane, three O-bound dimethylsulphoxide and two phenoxide-O-bound unidentate picrate ligands. For the O5 plane, χ2 = 7944, deviations δO(11,21,10,20,30) being –0.155(3), 0.030(3), 0.171(3), –0.116(3), 0.100(4) with δU 0.094(1) Å, the C6 aromatic planes 1,2 having dihedral angles of 69.97(9), 57.29(9)° to it, with the associated nitro planes (2,4,6) at angles of 20.9(2), 4.4(2), 43.2(2); 27.4(1), 3.3(1), 59.1(2)° to their parent C6 planes, i.e. those para are essentially coplanar, the ortho being twisted. U–O(n1)–C(n1) (n = 1, 2) are 132.2(2), 141.9(2)°, while U–O(n0)–S(n0) (n = 1–3) are 125.2(1), 115.3(1), 137.1(2)°, there being no consistent correlation with the associated U–O distance. As might be expected, the picrate aromatic planes overlap their inversion images in the lattice in an obligate parallel disposition.
The symmetry-inequivalent picrate ligands 1 and 2 are bound to 1,3 positions (i.e., separated by one DMSO ligand on one side and two on the other), with U–O(phenoxide) distances (2.356(3), 2.386(3) Å) considerably longer than in other either octahedral or pentagonal-bipyramidal uranyl aryloxides [9,28,29] perhaps in reflection of the relatively weak donor ability of nitrophenoxide-O. The U–O(DMSO) distances (2.357(3), 2.375(3), 2.419(2) Å) are comparable to those in [UO2(DMSO)5](ClO4)2 [7]. Throughout the lattice, ligands 1 and 2 form independent parallel arrays of centrosymmetrically related proximal pairs, representative of two variants on picrate stacking. Although the C6 entities are essentially planar, the oxygen atoms of the 2,6 nitro groups are significantly displaced (to different extents) from this plane (see above), at first sight perhaps unsurprisingly, because they are not involved in chelation to uranium.
In fact, although nitro-group rotation out of the aromatic plane is commonly seen in picrates, in the present case the degree of rotation of one on each of the two picrate ligands 1,2 is extreme (> 40°). This appears to be a concomitant of other unusual aspects of the structure. For picrate 1, there is a close approach (3.129(3) Å) of one of the uranyl-oxygen atoms to the N atom of the (rotated) 2-nitro-group of picrate 1 and a close approach (3.109(4) Å) of one of the same nitro-group oxygen atoms to an unsubstituted carbon atom of the same picrate ligand in an inversion-related complex unit. This ‘intermolecular’ C···O contact is reciprocated, and projection of one ring upon the other shows that not only do the aromatic rings lie closely parallel but that they overlap significantly (Fig. 3), consistent with other contributions to this stacking, though the shortest atom-atom distances are nearer 3.5 Å. Within the complex unit, there is also a close approach (3.046(3) Å) involving a DMSO-O and the nitro-group N(22) of picrate 2 and a moderately close approach (3.890(3) Å) of a DMSO-S atom to the N(26) atom of the other nitro group of this picrate. While binding of the phenoxide-O to uranium must necessarily bring the 2,6 substituents into close proximity with the rest of the complex, the specific forms of the closest approaches indicate that attractive forces may compensate for repulsions (‘steric effects’) that have been identified as particularly important in uranyl aryloxides [28]. Like picrate 1, picrate 2 is involved in an apparent stacking array through the lattice, again as essentially a sum of pairwise ‘intermolecular’ interactions. Here, however, projection perpendicular to the ring planes shows essentially no overlap of picrate entities but solely a quite short approach (2.993(4) Å) of a 2-nitro-group oxygen atom (less twisted from the plane than in picrate 1) of picrate 2 to C(3) of its image.
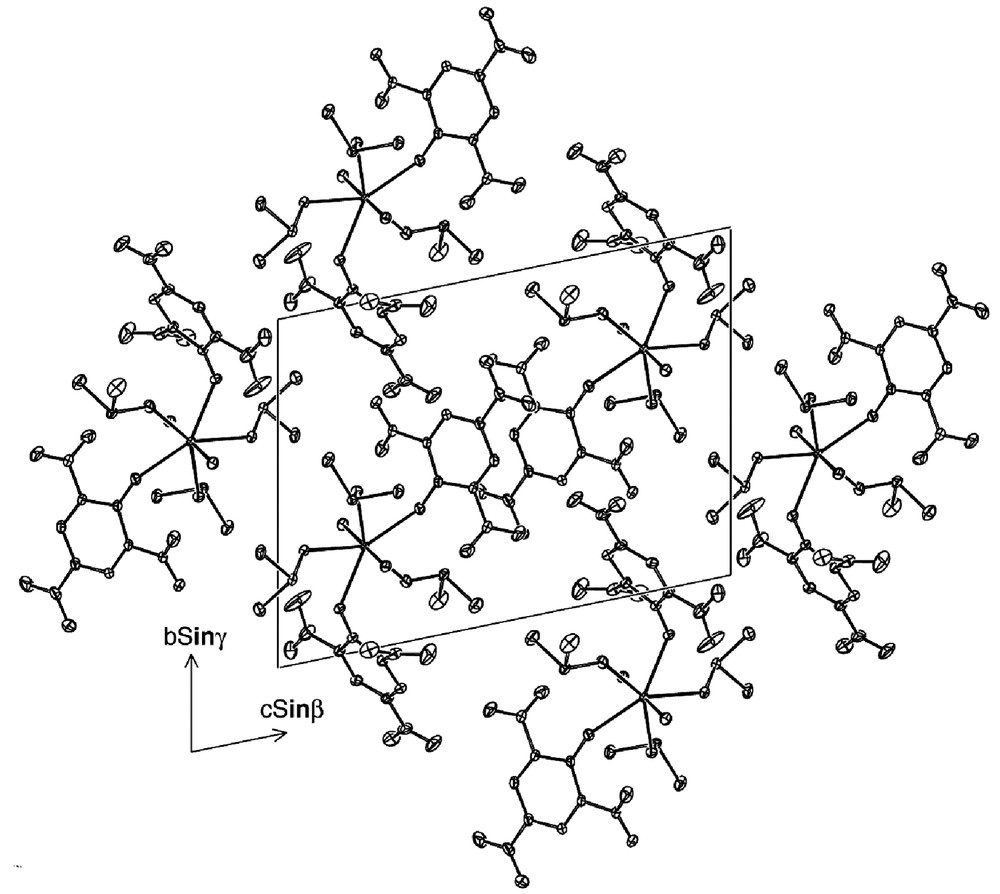
Unit-cell contents of 3, projected down a, showing the packing of inversion-related picrate groups.
Uranyl picrate is only the second actinide metal picrate to be structurally characterised [36]. It provides yet another variation on the theme of the balance between stacking interactions and coordinate bonding in metal picrates [26], both the degree of twisting of nitro groups from the aromatic plane and the proximity of intermolecular nitro-O/aromatic-C being unusually pronounced. Clearly, the term ‘stacking’ is highly ambiguous in regard to the specific centres which may be involved even when just a single species, like picrate, is considered but, in the case of uranyl picrate, it is of a form compatible with phenoxide-O coordination of both anions of the stoichiometric unit. Coordination accompanied by stacking for all picrate units is common with metal(II) cations but not for known lanthanide(III) species and is not found for Th(IV) [36]. The seemingly unique involvement of picrate with uranyl oxygen in the present uranyl picrate solvate has a parallel with some recent independent work [24] demonstrating the binding of alkali metal ions to uranyl oxygen in homo-oxacalixarene compounds.
Although, in aqueous media, acetate is rather ineffective at deprotonating water, one of the well-known characteristics of dipolar, aprotic solvents (such as DMSO) is their capacity to enormously enhance the basicity of anions (as a consequence of their inefficient solvation of such species) [1]. Thus, assuming that the dissolution of a labile species such as [UO2(O2CCH3)2(OH2)2] is likely to result in a proton redistribution favouring the formation of CH3CO2H and OH–, with coordination to U(VI) favouring further conversion of the latter to (bound) oxide, the deposition of an oligomeric, oxo-bridged species, [(UO2)4-(μ3-O)2(DMSO)4(CH3CO2)4] (Fig. 4), from a solution of uranyl acetate dihydrate in DMSO can be understood. The DMSO entities, as in all complexes described herein, are coordinated as unidentate oxygen donors. The tetranuclear array is centrosymmetric, i.e. one half of that unit comprises the asymmetric unit of the structure. The total symmetry of the cluster approaches 2/m, the major departure being the disposition of the peripheral S(CH3)2 components of the DMSO.
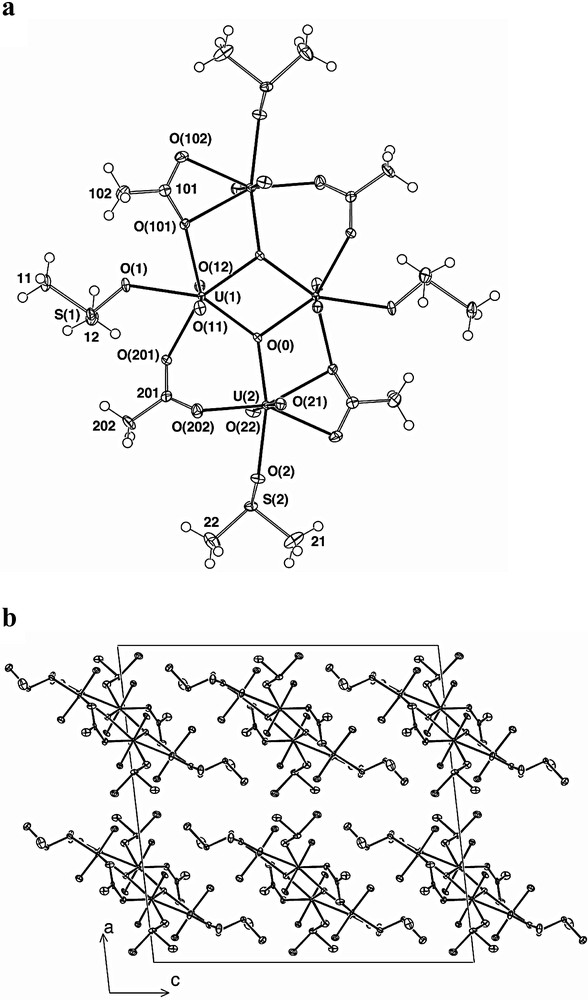
(a) A projection of the centrosymmetric [(UO2)4(μ3-O)2(DMSO)4(CH3CO2)4] molecule, normal to its ‘plane’. (b) Unit-cell contents projected down b, showing the packing of the molecules quasi-parallel to that axis.
The two independent uranyl components both have the usual UO2 quasi-linear axis, and about both there are five oxygen atoms, one from a DMSO ligand, in their equatorial planes. The remaining four donors are made up of one (U(2)) or two (U(1)) μ3-oxo donors and carboxylate oxygen atoms. The μ3-oxo donors link the four uranium atoms into a quasi-planar array, reinforced by bridging by both carboxylates, one of which also chelates a uranium. The core of the array is a necessarily planar (and familiar [37]) U2(μ(3)-O)2 four-membered ring, the two uraniums being U(1) and its image, the oxygens (O(0)) likewise. The array is extended by the incorporation of U(2) into further four-membered rings via carboxylate O(101), the array of three rings in projection (Fig. 4a) having the aspect of a tetranuclear chair cluster. Such a form is precluded by the use of uranyl, rather than tetrahedral metal atoms; acetate 1 chelates U(2) (O(101) bridging, O(102) terminal), while acetate 2 via its two terminally bound oxygens O(201,202) bridges U(1) and U(2), the geometries of the two ligands being quite different, as might be expected. The result is a planar array of uranium atoms, linked by atoms and ligands which are closely associated with that plane as is seen in Fig. 4b. Oxygen atom deviations δ(O(0;1,2;101,102,201,202) from the U4 plane are -0.168(4), –0.031(5), 0.191(5); 0.550(5), 0.608(5), –0.531(5), 0.599(5) Å, the dihedral angles to the carboxylate planes being 23.2(2), 31.4(2)°. Tenuous interactions of inter- and intramolecular nature are seen as in the other complexes presently discussed, the S of one of the DMSO ligands of the asymmetric unit being but 3.155(5) Å (intramolecularly) from a uranyl-O, while that of the other DMSO ligand is remote (3.883(4) Å) from uranyl-O but close (2.867(5) Å) to one oxygen atom of a chelating acetate unit. Intermolecular approaches to DMSO methyl include those to uranyl-O (3.247(8) Å) and to bridging (but non-chelating) acetate-O (3.205(8) Å), and (chelating) acetate methyl-C approaches DMSO-O at 3.30 Å. The shortest U···U separation in the tetranuclear unit is 3.6917(6) Å between the U(1) pair and may indicate a very weak metal-metal interaction. Other U···U separations are 3.8534(6), 4.0967(6) and 7.0451(8) Å.
In all the present compounds except the nitrate, uranium can be regarded as 7-coordinate. Bond lengths to the equatorial DMSO ligands are unsurprisingly similar, though in all instances (as in [UO2(DMSO)5](ClO4)2) there is a significant range of U–O distances, with the extremes varying from 2.481(5) for one of the ligands in the tetranuclear acetate to 2.322(8) for one in the triflate. To what extent these may reflect secondary interactions of the DMSO ligands described above is difficult to say but, in the tetranuclear acetate, for example, the shorter U–O(DMSO) distance is that associated with the ligand where there is a short intramolecular approach of acetate oxygen to S. In the nitrate, where the U–O(DMSO) separation is shorter than the mean for any of the presently studied species, a stronger interaction and thus bond-length shortening might be anticipated because of the relatively long and weak U–O(nitrate) bonds (or because of the lower coordination number, if nitrate is regarded as occupying a single coordination site) but it is accompanied by quite short intramolecular S…O approaches. In the picrate, it has been noted above that the U–O(phenoxide) bonds are long compared to those for unsubstituted aryloxides but it is also true that they are similar in length to those to DMSO-O, perhaps again because of cooperative interactions between bound DMSO and picrate. In terms of the primary coordination sphere about U, the picrate is very similar to [UO2(OClO3)2(OH2)3] [13] where, despite expectations based on the supposedly poor coordinating ability of perchlorate, the <U–O> is essentially identical for both water and perchlorate. Here, it is possible that intramolecular H-bonding assists coordination. While this implies that DMSO may not be unique in its capacity to undergo interactions other than ‘simple’ coordination, it does appear to be a molecule that is remarkable, given its simplicity, for the variety of weak interactions in which it may be involved. That these are prominent in uranyl ion complexes may be a consequence of the crowding of at least five donor atoms in the equatorial plane of such species.
4 Conclusions
The significance of studying dimethylsulphoxide in particular as a ligand should not be exaggerated and its value in the present case lies largely in the ease with which crystalline materials suitable for X-ray diffraction measurements can be obtained. Structures of the present and many related complexes of DMSO [2–4,12,21,38] do have a general value, however, in defining some of the consequences of the action of attractive, as distinct from repulsive or ‘steric’ forces between ligands on the form of their complexes, especially as there is no reason to expect that such effects should be limited to DMSO [39]. Further, evidence that solution and solid-state structures of solvento complexes are commonly identical [12] means that these attractions may be determinants of solution properties as well as those of solids. In the case of uranyl ion as the coordination site for DMSO, the uranyl-O atoms provide a special site for interaction with the S-centre of O-bound DMSO and this appears to be of sufficient strength to compete with other weak intermolecular forces in controlling the bound ligand conformations. From broader considerations of DMSO complex structures [21], it has been estimated that the energy of a DMSO/DMSO O···S interaction may be ~4 kJ mol–1 and while this is small, when more than two DMSO ligands are present, the total energy could readily attain a value (~10 kJ mol–1) sufficient to change a rate or an equilibrium constant by a factor of 102. Thus, O-coordination of DMSO, as observed for U(VI), is no simple process, what is formally a Lewis acid/Lewis base interaction of metal and O-donor atom being the cause of a variety of other possible acid/base processes.
1 Very similar structures have also been recently reported for the analogous complexes of diphenyl- and dibenzyl-sulphoxide [14b].