1 Introduction
The advantage of homogenous catalysis is the high molecular definition of the active sites allowing an excellent control on the selectivity of the catalytic reactions. However, separation and recovery from the reaction mixture are drawbacks in comparison to heterogeneous catalysis. Fixation of the catalyst on a solid support usually leads to a loss of selectivity. Nevertheless, nature shows us that prearrangement of some functions near the active site of metalloproteines favours selectivity [1]. In order to have controlled porosity and relatively large pores to allow active site definition and substrate access, mesostructured porous silica can be used. The so-called MCM-41 (hexagonal symmetry) exhibit extremely high surface area and well defined pore size as well as high thermal stability. Since their discovery in 1992 [2,3] intensive efforts have been devoted to the development of synthesis and characterisation techniques, in order to obtain materials suitable for various chemical, biochemical and physical applications [4,5]. These materials can easily be modified and functionalised by incorporation of organic groups in the silica structure either by grafting silane moieties on the pre-formed silica by reaction with surface silanol and siloxane groups [6], or through the use of substituted trialcoxysilanes in the sol-gel synthesis, which co-condensate with the silica source [7].
When dual functionalisation is at stake, one has to deal not only with each function distribution but also with the vicinity of each function one to another. Different approaches are described in the literature to obtain a material possessing a surface with site-isolated groups and controlled distance between the grafted functions. Wulff et al. [8,9] demonstrated that it is possible to graft two amine groups at a specific distance one to each other by connecting them via a spacer which is removed by hydrolysis under mild conditions after grafting. Sasaki et al. used a similar procedure to position three amines using different types of tripod templates on a silica-gel surface [10,11]. This approach was extended by Shin et al. to position two or three amines (dipod or tripod, respectively) on mesostructured porous silica (SBA-15), creating cavities of about 1.0 nm [12,13]. A similar approach was developed by Katz and Davis [14] who anchored three amine groups a specific distance from each other using in this case a direct synthesis of imprinted silica. In all of these cases the distance between the two or three functions in each site is controlled but a low loading of the function (<1 molecule nm–2) is required to obtain site isolation. In order to have higher loading a molecular patterning technique can be used as described by McKittrick and Jones [15]: a voluminous trithylimine patterning agent is used to spatially position amine sites a minimum distance from each other. However, in both strategies a statistical distribution of the sites is obtained. We have developed a new strategy which consists to take advantage of the presence of the positively charged surfactant in the as-made silica, the positioning of which is ruled by electrostatic repulsion. In fact, one of us has shown that it is possible to graft trimethylsilyl (TMS) groups while maintaining most of the surfactant inside the channels [16]. Then, the remaining surfactant can be displaced by cation exchange or acid treatment prior introduction of the second function [17]. The surfactant molecules act in both cases as protecting groups homogeneously distributed onto the surface. This is a novel approach based on the use of a so-called ‘molecular stencil pattern’. The surfactant plays here both roles: (i) patterning from coulombic repulsion and, (ii) stencil from surface protection-deprotection.
Dual functionalisation of the surface is proposed here for the first time using grafting for the second step of the molecular patterning approach. In addition, it is tested whether more complex or sterically hindered functions could be introduced in the first step of this approach. The grafting has been performed starting with mesostructured silicas containing the surfactant and avoiding any calcination step, to work with the highest silanol surface density. The functions such as alkyldimethylchlorosilane or siloxane, R(CH3)2SiCl or [(CH3)3Si]2O, R = methyl or 3-chloropropyl, have been deliberately chosen with only one possible linkage to the surface to avoid their grafted oligomers. Then the only side reaction could be formation of molecular dimers, which should be removed by appropriate washing. The TMS function (Fig. 1d) is known to tune the hydrophobicity of the surface and, the chloropropyl group (Fig. 1b) allows further chemical derivatisation through nucleophilic substitution. The challenge here is to maintain as much as possible the first function on the surface when introducing the second one.
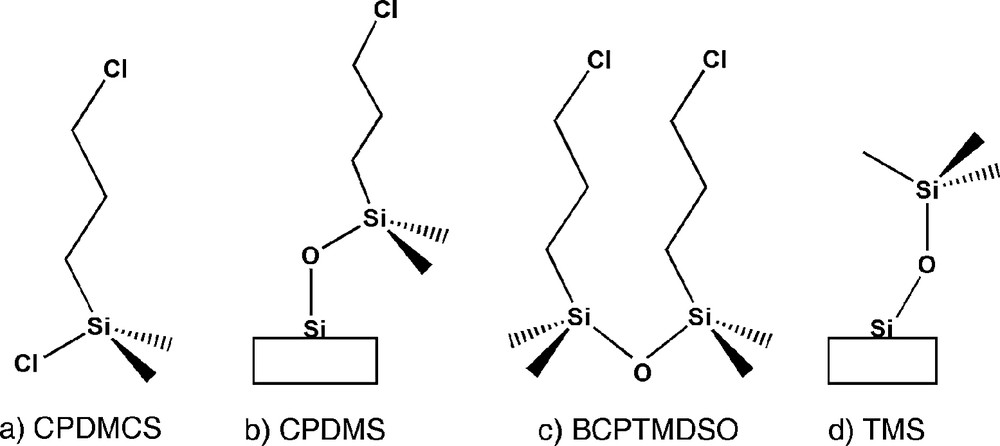
Species present in mesopores.
2 Experimental part
2.1 Instrumentations
Low-angle X-ray powder diffraction experiments have been carried out using a Bruker (Siemens) D5005 diffractometer using Cu Kα monochromatic radiation. Infrared spectra were recorded from KBr pellets using a Vector 22 Bruker spectrometer. Nitrogen adsorption–desorption isotherms at 77 K were determined with a volume device Micromeritics ASAP 2010M. 1H and 13C liquid NMR were recorded on a Bruker AC 200 MHz spectrometer. 13C and 29Si CP-MAS solid NMR measurements were collected on a Bruker DSXv400 spectrometer (for both cross-polarisation (CP) was used). For 29Si (79.49 MHz), a 4 μs (corresponding to θ = π/3) pulse was used with a repetition time of 4 s. For 13C (100.6 MHz), a 6 μs (θ = π/2) pulse was used with a repetition time of 3 s. The spinning rate of the rotor was about 5 kHz and the number of scans, between 2000 and 15,000, depends on the rate of grafting. For 29Si MAS solid NMR, a 3.5 μs (corresponding to θ = π/4) pulse was used with a repetition time of 60 s. The spinning rate of the rotor was about 10 kHz.
2.2 Materials
Hexadecyltrimethylammonium-p-toluene-sulfonate (CTATos) (> 99% Merck), chlorotrimethylsilane (CTMS) (98% Acros), hexamethyldisilazane (HMDSA) (98% Acros), hexamethyldisiloxane (HMDSO; 98% Acros), Ludox HS-40 (40% SiO2 Aldrich) and 3-chloropropyldimethylchlorosilane (CPDMCS; 97% ABCR) were used as received. Toluene (Merck), acetonitrile (SDS) and ethanol (VWR) were distilled and stored under nitrogen.
2.3 Synthesis of LUS-1
LUS-1 was prepared as follows [18]: Ludox (15.5 g, 0.26 mol) was added to sodium hydroxide (2 g, 5 × 10–2 mol) in deionised water (50 ml), then stirred at 313 K until clear (about 24 h). A second solution of hexadecyltrimethylammonium p-toluenesulfonate (CTATos) (2.5 g, 5.5 × 10–3 mol) in deionised water (90 ml) was stirred during 1 h at 333 K. The first solution was dropwise added to the second one, then stirred at 333 K during 2 h. The resulting sol-gel was heated in an autoclave at 403 K during 20 h. After filtration and washing with deionised water (approximately 1000 ml), the as-synthesised solid was dried at 353 K. Elemental analysis – LUS-1: C (33.1%), H (7.6%), N (2.0%), weight loss at 1273 K (49.7%). BET surface area of 940 m2 was obtained after solvent extraction (see below) and vacuum treatment at 423 K for 24 h.
2.4 Chloropropyl functionalisation: LUS-CP and LUS-PS-CP
As-synthesised LUS-1 (1.9 g) was dried at 353 K under nitrogen flow during 15 h, then placed in a round bottom two-neck flask where CPDMCS (5 ml, 3.0 × 10–2 mol) was added, pure or diluted either in toluene or in a toluene/acetonitrile mixture. The grafting reaction was run under different conditions provided in Table 1 (vide infra). The functionalised silicas (LUS-CPX) were washed and dried at 343 K. A similar procedure was adopted to functionalise a partially trimethylsilylated silica, LUS-PS (vide infra). Elemental analysis of the so-called LUS-CP1: C (28.7%), H (5.7%), N (1.4%), Cl (6.4%), weight loss at 1273 K (45.9%). Elemental analysis of LUS-CP2: C (20.7%), H (4.3%), N (0.4%), Cl (9.2%). Elemental analysis of LUS-CP3: C (32.2%), H (6.6%), N (1.4%), Cl (8.9%), weight loss at 1273 K (52.3%). Elemental analysis of LUS-CP4: C (15.3%), H (3.3%), N (< 0.1%), Cl (8.5%), weight loss at 1273 K (23.6%). Elemental analysis of LUS-PS-CP5: C (13.4%), H (3.1%), N (<0.1%), Cl (6.3%), weight loss at 1273 K (19.8%).
Grafting conditions of CPDMCS
Material | Solvent | T (K) | Reaction time (h) | Washing conditions | Drying T (K) |
LUS-CP1 | Toluene | 333 | 18 | Toluene + soxhlet (ether/CH2Cl2) | 343 |
LUS-CP2 | Toluene | 383 | 18 | Toluene + soxhlet (ether/CH2Cl2) | 343 |
LUS-CP3 | None | 393 | 2 | Toluene | 343 |
LUS-CP4 | None | 393 | 18 | Toluene | 343 |
LUS-PS-CP5 | Toluene/acetonitrile | 373 | 18 | Toluene/acetonitrile | 343 |
2.5 Trimethylsilylation: LUS-CP2-C, LUS-C and LUS-PS
Capping. The chloropropyl functionalised silica LUS-CP2 (1.5 g) was dried at 353 K under nitrogen flow during 15 h. In a round bottom two-neck flask, HMDSO (12.5 ml, 5.9 × 10–2 mol) and CTMS (7.5 ml, 5.9 × 10–2 mol) were added under nitrogen. The mixture was stirred 1 h at room temperature and then refluxed for 15 h. The obtained solid, LUS-CP2-C, was finally washed with ethanol (about 100 ml) and dried at 343 K. Elemental analysis of LUS-CP2-C: C (15.2%), H (3.4%), N (<0.1%), Cl (7.2%), weight loss at 1273 K (22.5%). This capping treatment was performed on as-made silica leading to a fully silylated sample, LUS-C. Elemental analysis of LUS-C: C (10.8%), H (2.9%), N (<0.1%), weight loss at 1273 K (11.0%).
Partial silylation. As-synthesised LUS-1 (0.5 g) was dried at 353 K under nitrogen flow during 3 h. Dry toluene (5 ml) and HMDSO (5 ml, 2.3 × 10–2 mol) were added to the solid under nitrogen. The mixture was stirred 1 h at room temperature and then refluxed for 16 h. The partially silylated solid, LUS-PS, was finally washed with toluene, acetone and ethanol, and dried under vacuum at room temperature leading to 0.4 g of product. Elemental analysis: C (27.9%), H (5.9%), N (1.5%), weight loss at 1273 K (41.9%).
3 Results
The synthesis in basic media using hexadecyltrimethylammonium-p-toluene-sulfonate (CTATos) templating agent produces mesostructured porous silica with a P6/m symmetry point group, which is MCM-41 structure like [2,3]. This solid, called LUS-1 [18], has been used as starting material to perform post-functionalisation, since it is highly mechanically and thermally stable [19]. The XRD patterns revealed that the hexagonal array of the channels of LUS-1 is maintained through the sequence of treatments undergone by the solids that are reported here (Fig. 2).

XRD diagram of a) LUS-1, b) LUS-CP2, c) LUS-CP2-C, d) LUS-PS-CP5.
First of all, the 3-chloropropyldimethylcholorosilane (CPDMCS) (Fig. 1a) was reacted with the as-made LUS-1 at different conditions as shown in Table 1 in order to optimise the grafting procedure. The amount of surfactant displaced was found to vary depending on the nature of the reaction mixture, reaction time and temperature, according to elemental analysis (Table 2) and 13C and 29Si NMR. Fig. 3 exhibits the 13C CP-MAS NMR spectra of as-made LUS-1 and the solids obtained upon different treatments with CPDMCS. The signature of the surfactant is the only signal present on the spectrum of LUS-1. When this solid was treated with CPDMCS new peaks at 47.2, 26.9, 15.4 and 0.2 ppm were observed and assigned to α, β, and γ carbons of the chloropropyl group and to the methyl groups bound to the silicon atoms, respectively. These resonances match well those of the molecular CPDMCS species in CDCl3 arising at 47.2, 26.6, 16.5 and 1.6 ppm. The intensity of the NMR signal due to the chloropropyl group compared to that of the surfactant increased with higher temperature (LUS-CP1 and LUS-CP2, Fig. 3b, c) and with longer reaction time (LUS-CP3 and LUS-CP4, Fig. 3d, e). The question remained whether these signals belonged to the unreacted CPDMCS or to its grafted analogue and, it will be discussed later. The 29Si CP-MAS NMR spectra of all the functionalised solids as well as the as-made LUS-1 are gathered on Fig. 4. Q2, Q3 and Q4 signals of silica are observed at –92, –100 and –109 ppm [20], respectively, on all spectra with various intensities depending on the treatment. Upon reaction with CPDMCS a new signal at around 15 ppm with a shoulder at about 20 ppm was observed, which is attributed to M type silicon species [SiC3O], where the silicon is bound to three carbon and one oxygen atoms. Conversely, the Q3 signal decreases, which is consistent with the reaction of silanols with the CPDMCS. Surprisingly, the variation of Q3 signal intensity was not always exactly matching the increase of M species. The CP conditions are known to affect the NMR signal intensity. Therefore, these conditions were kept all constant in the whole sequence of experiments. In addition, the magnetisation transfer during the CP experiment is different for each species. To overcome these difficulties, spectra were acquired with and without CP for one sample of the series to allow calibration. Therefore, the proportionality of the NMR signal and the concentration of each species was establish, allowing pseudo-quantitative analysis, used as such in the following. The problem of intensity concerning M and Q3 species is a matter of concern, discussed later.
Chemical analysis of grafted materials using CPDMCS
Elemental analysis | NMR | Coveragec | ||||
N/SiO2a | Cl/SiO2a | M/Qb | Sigrafted/SiO2a | % | ||
LUS-1 | 0.116 ± 0.001 | 0 | – | |||
LUS-CP1 | 0.118 ± 0.002 | 0.214 ± 0.004 | 0.06 ± 0.01 | < 26 ± 4 | ||
LUS-CP2 | 0.020 ± 0.006d | NAe | 0.19 ± 0.02 | ~0.19 ± 0.02 | ~83 ± 9 | |
LUS-CP3 | 0.146 ± 0.004 | 0.36 ± 0.01 | 0.15 ± 0.02 | < 65 ± 9 | ||
LUS-CP3-EXf | < 0.006 | 0.091 ± 0.001 | NAe | 0.091 ± 0.001 | 39 ± 1 | |
LUS-CP4 | < 0.007g [~0] | 0.226 ± 0.003 | 0.228 ± 0.007 | 0.229 ± 0.003 | 99 ± 1 |
a Molar ratio calculated considering SiO2 content obtained from weight loss at 1273 K and removing the contribution of the SiO2 formed for the grafted silane.
b M stands for a silicon atom bond to only one oxygen atom (a grafted species, for instance). Q stands for a silicon atom bond to four oxygen atoms.
c Assuming that 0.23 function per silicium atom in the silica wall corresponds to 100% coverage (vide infra results part).
d Calculated from 29Si and 13C NMR.
e Not available.
f After extraction, N/SiO2 is assumed to 0 to determine the other values.
g Maximal value due to the detection limit of elemental analyser (0.1% in nitrogen). The 13C NMR estimation of this value is in brackets.
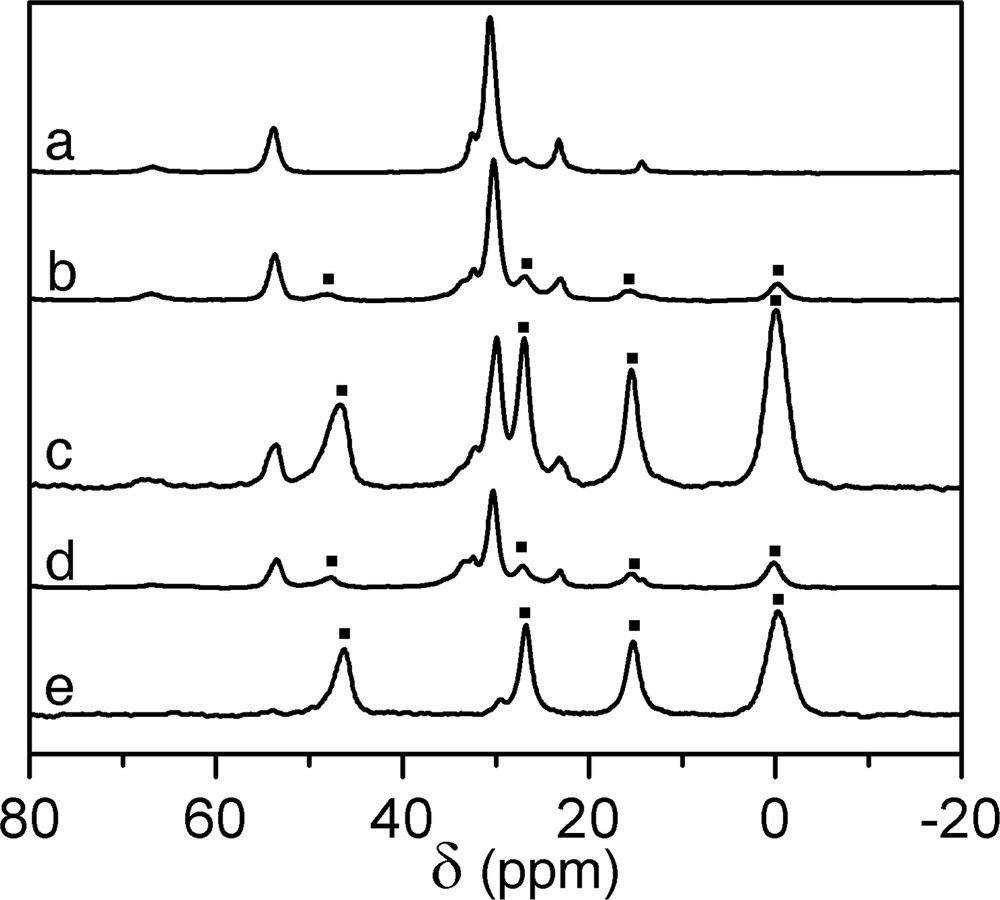
13C CP-MAS NMR spectra of (a) LUS-1, (b) LUS-CP1, (c) LUS-CP2, (d) LUS-CP3, (e) LUS-CP4. In spectrum (a), all the NMR resonances correspond to the surfactant. In the other spectra, the new resonances (■) at about 0, 15, 27, 47 ppm belong to the reacted CPDMCS.
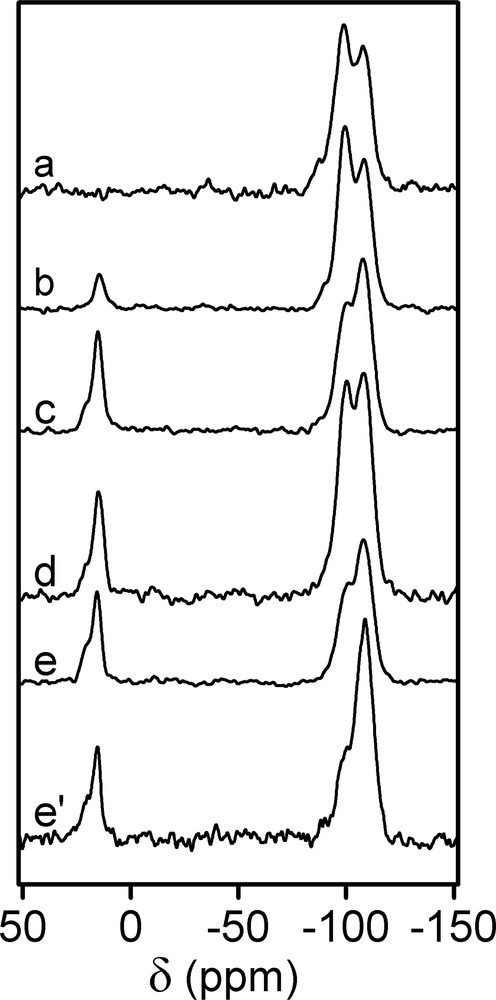
29Si CP-MAS NMR spectra of (a) LUS-1, (b) LUS-CP1, (c) LUS-CP2, (d) LUS-CP3, (e) LUS-CP4, and (e′) 29Si MAS NMR spectrum of LUS-CP4. Q2, Q3 and Q4 signals at about –88, –100 and –108 ppm, respectively, and M at ~15 ppm with a shoulder at about 20 ppm.
Nonetheless, one may conclude looking at spectrum e on Fig. 3, that the surfactant can be quantitatively displaced during the reaction of the solid with pure CPDMCS at 393 K during 18 h. This is consistent with the work of Antochshuk and Jaroniec [21–23], who made similar observations using pure n-octyldimethylchorosilane or pure 3-cyanopropyldimethylchlorosilane. It is further shown here that a similar quantitative displacement of the surfactant can be obtained with twelve times less chlorosilane, diluted in a mixture of toluene and acetonitrile (NMR spectra similar to that of LUS-CP4 and not shown here).
The first step of the dual functionalisation consisted in synthesising partially grafted silica maintaining, as much as possible, the templating surfactant. Indeed, a partially trimethylsilylated silica (ca. 40%, vide infra), LUS-PS was obtained using HMDSO. The 13C CP-MAS NMR effectively reveals the presence of both surfactant and TMS species, the former exhibiting resonances at the same chemical shift as given before, and the latter at 1.8 ppm (Fig. 5, top). In the second step, this solid was treated with CPDMCS in a mixture of toluene/acetonitrile (1:1, v/v), LUS-PS-CP5, as shown in Table 1. The 13C NMR spectrum (Fig. 5, bottom) is consistent with complete removal of the surfactant and the presence of chloropropyl groups characterised by resonances at the same positions as pointed out above. It is worth to notice that the signal at about 0 ppm is higher than that of the grafted LUS-CP4 (Fig. 3e). This suggests that this signal accounts for methyl groups of both TMS which remain on the surface and CPDMS groups present in the final solid (Fig. 1d and Fig. 1b, c, respectively). The 29Si NMR spectrum (Fig. 6) allows checking if the function was grafted. Indeed, a decrease of the Q3 signal concomitantly with an increase of the M signal was observed upon treatment with CPDMS. A new bifunctionalised solid was synthesised inversing the order of incorporation of the two previous functions. A partially chloropropylated sample still containing some of the templating agent (LUS-CP2) was treated with an equimolar mixture of HMDSO and CTMS. The templating agent was displaced by the TMS groups (LUS-CP2-C), as it is shown in the 13C NMR spectra (Fig. 7). Indeed, an increase of the 0 ppm signal relative to the other peaks was observed, which confirms the presence of TMS groups. However, the TMS/CPDMS ratio was smaller than in the previous case. Comparing 29Si NMR spectra of both LUS-CP2 and LUS-CP2-C (Fig. 8), it appears that the M signal drastically increases while the Q3 signal stays nearly constant. This suggests that the presence of the TMS function is not necessarily correlated to its grafting (see Section 4).

13C CP-MAS NMR of LUS-PS (top: all resonances belong to the surfactant except the signal (#) at 1.8 ppm assigned to TMS) and LUS-PS-CP5 (bottom: all resonances (■) correspond to CPDMS and TMS).
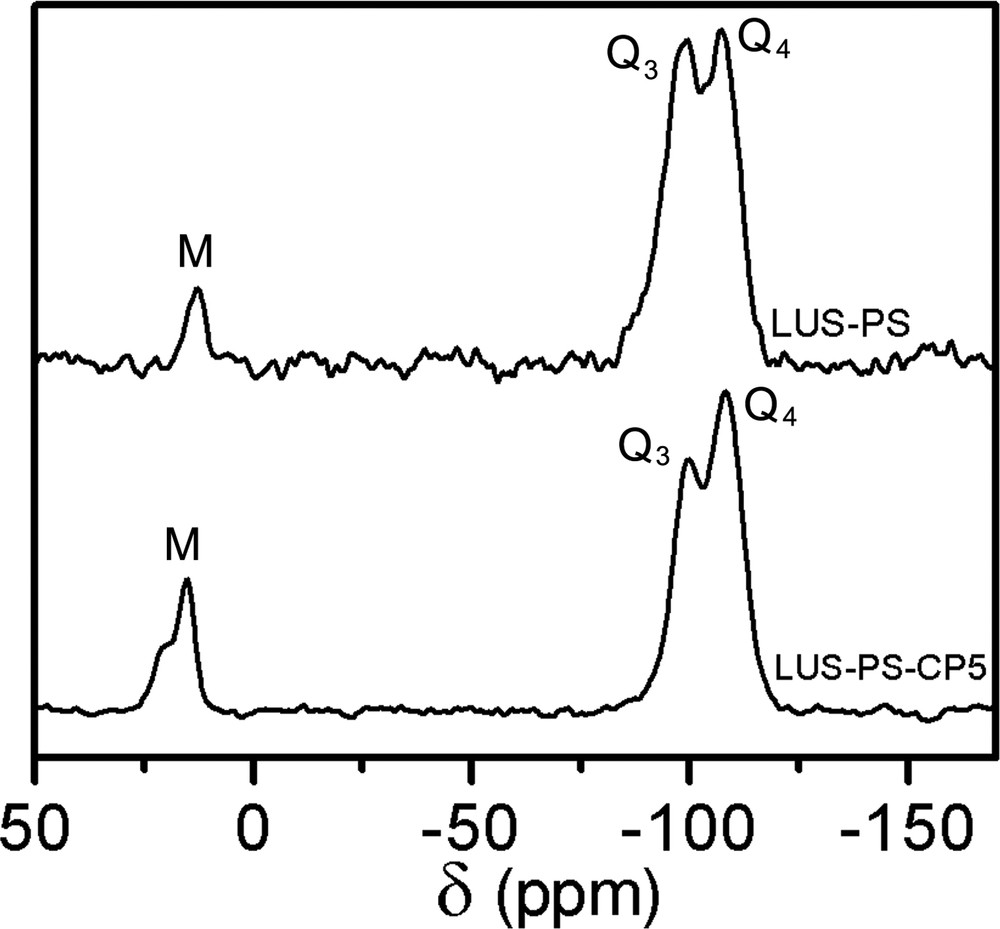
29Si CP-MAS NMR of LUS-PS (top) and LUS-PS-CP5 (bottom).

13C CP-MAS NMR of LUS-CP2 (top; surfactant and CPDMS are present) and LUS-CP2-C (bottom; all resonances correspond to CPDMS and TMS).
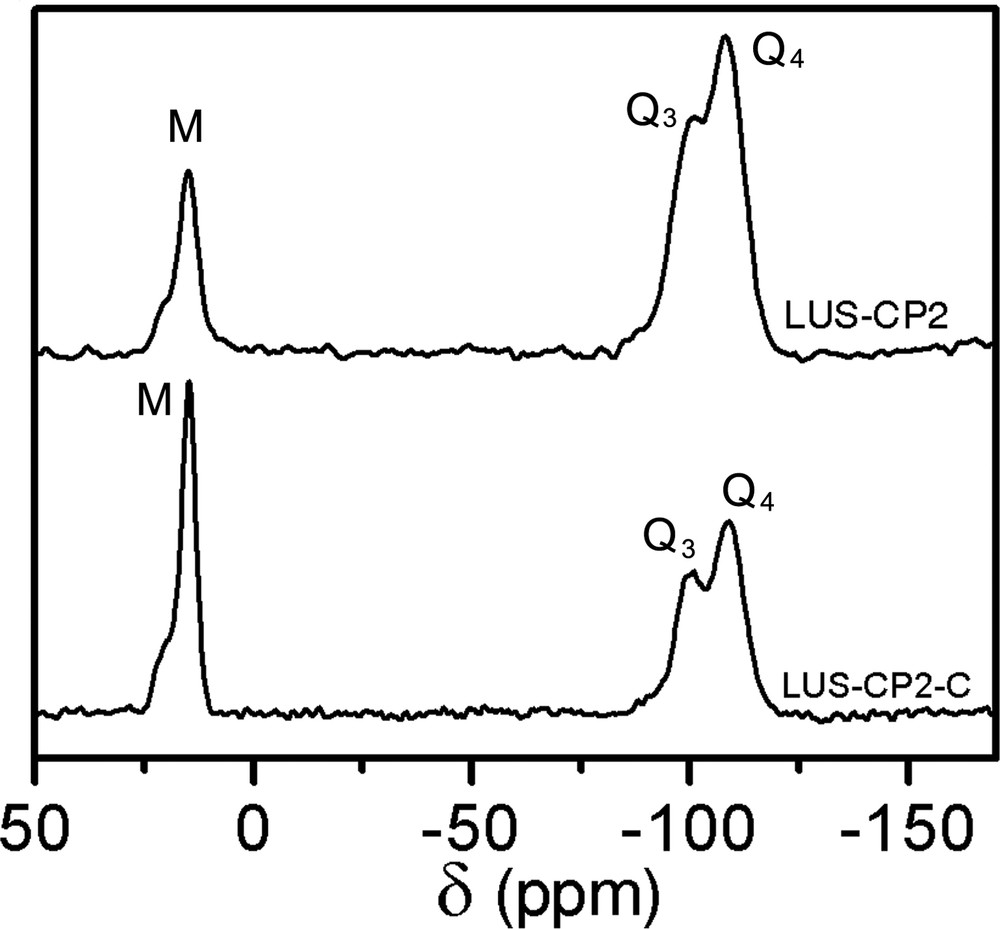
29Si CP-MAS NMR of LUS-CP2 (top) and LUS-CP2-C (bottom).
To further discuss the results the chemical analysis obtained from both elemental analysis and 29Si NMR spectra are gathered in Tables 2 and 3 for mono- and bifunctionalised silicas. The coverage for each function has been calculated from elemental analysis and is given in percentage relative to a totally silylated sample, LUS-C, with a 0.23 function/SiO2 ratio. This value corresponds to 2.45 grafted functions per nm2 assuming a specific surface of 940 m2 g–1 or 3.0 mmol g–1 of solid before weight loss at 1273 K. In the following, we have considered that this value corresponds to total coverage of the surface consistently with previous results obtained for complete trimethylsilylation of analogous mesoporous silica [24,25]. In some cases, solid state NMR has been used semi-quantitatively to complement or crosscheck chemical analysis. The surfactant and grafted CPDMS content can be obtained from N and Cl elemental analysis, respectively. For all chloropropylated samples, it has been assumed the formation of CTA+Cl– entities (vide infra). For samples containing traces of surfactant, the limit of detection of nitrogen was reached1 and an estimated value from 13C NMR has been used. If the second function, TMS, is present, the TMS/CPDMS ratio can be deduced from the Cl/C ratio. The different molar ratios are calculated considering SiO2 content obtained from weight loss at 1273 K and removing the contribution of SiO2 formed from the grafted silanes. The M/Q ratios calculated from 29Si NMR signals can be related to the content of grafted silanes vs. the bulk silica. Note that, for sample LUS-CP4 the M/Q ratio of 0.228 obtained from the 29Si MAS NMR nicely fits the chemical analysis (Cl/SiO2 ratio of 0.226). Besides, it appears, in Table 2, that there is a discrepancy between chlorine content and the number of chloropropyl species characterised from NMR as M species. This is discussed below.
4 Discussion
Both chemical analysis and solid state NMR data are discussed in the following in term of grafting efficiency, and dual functionalisation strategy. Indeed, it is important to understand how the grafted molecules lay down onto the surface analysing both the maximum coverage achievable for each function and checking their molecular integrity. The 13C NMR signal close to 2 ppm (LUS-PS) is consistent with partially grafted TMS species in presence of surfactant. According to Sindorf and Maciel [26], in calcined silica TMS resonance arises at 0 ppm. It has been previously observed that this downfield shift is related to the close vicinity of the cationic surfactant and reversibly disappears upon surfactant removal [16,24]. Concerning the CPDMS groups, chemical shifts (see above) reveal that the chloropropyldimethylsilyl (CPDMS) moieties are fully intact. When both functions are grafted together, there is no new resonances, however, as the surfactant was removed, the signal close to 0 ppm accounts for both TMS and dimethyl moieties of the CPDMS which preclude any definitive conclusion concerning the presence of TMS. Nonetheless, it is reasonable to think that it is also the case when they are grafted altogether on the surface since their molecular integrity is maintained when they are grafted alone. To the contrary, when the grafting is performed with chloropropyltriethoxysilane (CPTES), the TMS peak is clearly identified and not superimposed to the CPTES peaks (results not shown here). However, an additional resonance at 66 ppm appears on the spectra that can be explained assuming that some of the C–Cl bonds have been replaced by C–O bonds via nucleophilic substitution of chlorine probably due to the presence of residual water localised at the surfactant–silica interface [27]. This drawback disappears on fully dehydrated surfaces obtained after calcination [6,28]. In the literature, most of the studies report on grafting performed on calcined material. In addition, triethoxysilane or trimethoxysilane anchoring fragments are preferred and widely used because a large range of functions is available [6,7,11,12,15,28–32]. Furthermore, the advantage of such fragment is to allow high loading of specific functions, which are useful for example for immobilised chromatographic phases. For instance, functions density as high as 4.9 molecule nm–2 can be achieved in a MCM-41 [33]. Since the silanol density in the latter material is around 3–4 molecule nm–2 [25], such a high density of grafting implies that the ethoxysilanes oligomerise via siloxane bridge formation, Si–O–Si, leading therefore to a loss of control in the surface definition. Here, the use of dimethylchlorosilane anchoring fragment avoids oligomerisation and the maximum surface coverage should correspond to a molecular monolayer. Then, the coverage limitation should be related to the size of the molecule.
According to Sindorf and Maciel [34], a single TMS group covers 0.43 nm2, leading to the theoretical maximum coverage of 2.33 molecules nm–2 on a flat surface. Indeed, maximum TMS coverage of 2.6 molecules nm–2 in a MCM-41 material has been reported by Badiei [24] using CTMS as silylating agent. In addition, TMS packing as high as 2.45 and 2.7 molecules nm–2 were also reported in amorphous silica using HMDSA [35] and CTMS [36], respectively. For LUS-CP4, taking into account a surface area of 940 m2 g–1, the M/Q molar ratio of 0.228 (Table 2) corresponds to 2.43 CPDMS molecules nm–2 comparable to the maximum for TMS. Such high value can be explained assuming that the chloropropyl chain points upward perpendicularly to the surface. Unfortunately, rigorous comparison of function loading and coverage with literature data are not always straightforward, since it is not clearly stated whether the weight of the solid refers to the material as it is, including the grafted function or, obtained after weight loss at high temperature (i.e. the SiO2 weight only). Brunel et al. have grafted on calcined MCM-41 various functions using trialkoxysilanes as anchoring fragments, and loading varies from 0.6 to 1.3 molecule nm–2 [6,28–31] while using chlorosilanes loadings up to 1.9 molecule nm–2 were achieved [25,37]. Nonetheless such loadings are substantially lower than those obtained on the actual uncalcined mesostructured silica, characterised by a higher density of silanol groups. It is worth noticing that the non-functionalised extracted LUS presented a much higher BET constant of 230 than after calcination (138), consistently with much polar surface due to a higher density of silanol groups and therefore grafting capacity. Accordingly, previous studies on as-made mesoporous silica functionalised by displacement of the surfactant exhibit higher function loading than the related functionalised calcined samples [22,23,38]. In addition, Antochshuk et al. have demonstrated that alkyldimethylchlorosilane are more efficient than alkyltrialkoxysilane ones, both used as pure reactant (absence of solvent). For instance, the loading using n-octyldimethylchlorosilane on MCM-41 silica (2.4 molecules nm–2) is about 30% higher than when n-octyltriethoxysilane is used (1.8 molecules nm–2) [22].
In the present study, partial displacement of the surfactant and therefore partial coverage of grafted CPDMS was obtained after 2 h of reaction at 393 K in pure reactant (LUS-CP3) while full coverage is achieved after 18 h of reaction (LUS-CP4). In the latter case, the nice match in Cl loading and M species indicates an efficient removal of all HCl molecules evolving from the condensation of silanol groups with chlorosilane or eventually from the dimerisation of CPDMCS in presence of water residing at the surfactant–silica interface in the as-made mesostructured silicas (Fig. 1c). By contrast, when full coverage is not completed (LUS-CP3), there is a large excess of chlorine content in comparison to chloropropyl function estimated from the M/Q ratio. This indicates that part of the chloride evolving as HCl from the reacting Si–Cl moieties is retained in the solid. Since the {SiO–, CTA+} interface is rather basic at the beginning of the grafting process, the acidic HCl neutralises the silanolate SiO–. The surfactant is retained with chloride as ion pairs at the interface, {SiOH, CTA+, Cl–}, since it is not soluble in the reaction media and the washing solvents. This explains only part of the excess of chlorine. The other sources of chloride besides the grafted functions are more likely unreacted CPDMCS and HCl entrapped in the pores. The former species can be discarded since no SiCl species was detected on the 29Si NMR.
In order to evaluate how much of chlorine pertains to the grafted functions, a thorough extraction of the molecules not covalently linked to the surface is needed. This was performed by contacting the solid with ammonium acetate three times at 333 K for 20 min in ethanol, LUS-CP3-EX. Elemental analysis [C (11.4%), H (2.7%), N (<0.1%), Cl (4.0%), weight loss at 1273 K (20.1%)] confirms that most of the surfactant has been removed. Some chlorine is effectively retained, that can be assigned to grafted CPDMS groups (Cl/SiO2 ratio = 0.091) which is notably smaller than the M/Q ratio of 0.15 measured by NMR before extraction. The rational for such a high M/Q ratio is indeed the presence of unreacted chlorosilane or its hydrolysed products (chloropropyldimethylsilanol or bis(chloropropyl)tetramethyldisiloxane BCPTMDS, Fig. 1c) inside the channel of non-extracted samples (Fig. 9) assuming that the silicon NMR signals of these species are superimposed at the ‘M’ resonance. The retention of non-grafted molecular silane in surfactant containing samples strongly support the idea that the surfactant confined in the channel plays the role of solvent, which strongly competes with toluene chosen here to wash out the sample. This is a drawback for chemical analysis and for determination of the grafting level if the surfactant is not removed. As a consequence, the coverage calculated from the M/Q ratio obtained from NMR is overestimated when the surfactant is present, as it is the case for LUS-CP3 and also for LUS-CP1. By contrast, for LUS-CP2 containing much less surfactant, the coverage from M/Q ratio is expected to be close to the effective value. Moreover for LUS-CP4, where only traces of surfactant are left after grafting, the coverage calculation from M/Q ratio is correct and consistent with full coverage.
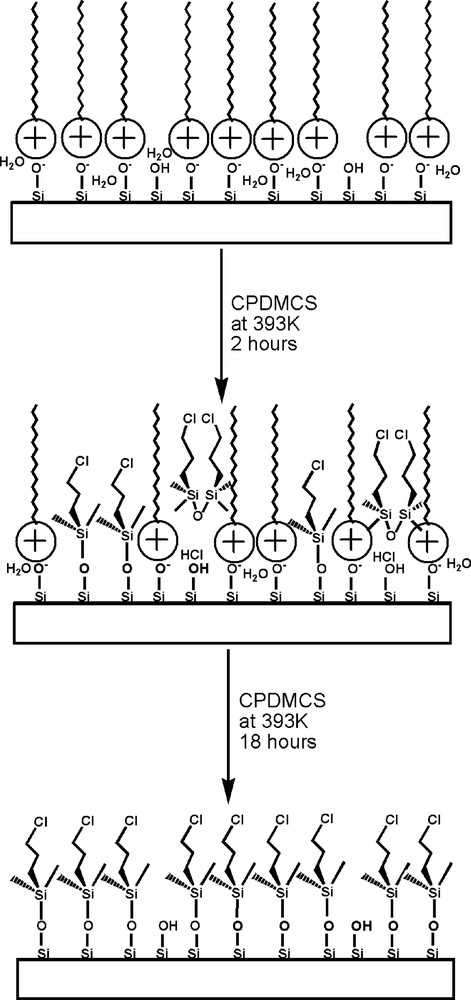
Scheme of surface evolution during CPDMS grafting: as-made LUS-1 (top), after 2 h LUS-CP3 (middle), after 18 h LUS-CP4 (bottom).
Considering now the grafting of two functions, both strategies are quantitatively compared in Table 3. When the CPDMS was grafted in the first step, HCl was liberated partially displacing the templating surfactant. The surfactant molecules remaining entrapped inside the pores are associated with chloride ions (see above). They do not play anymore the role of molecular patterning, since they do not interact electrostatically with the surface. In this case, the distribution of the grafted CPDMS function is likely to be diffusion-controlled and concentrated in the entrance of the channels. Then the grafting of the second function, TMS, is likely to occur where the CPDMS has not been grafted. Furthermore, the presence of ungrafted species observed by 29Si NMR makes the value of coverage uncertain. By contrast, when TMS was grafted in the first place, the surfactant was retained inside the channels, leading to a more homogeneous distribution of the grafted function as proposed earlier [18]. Then during the grafting of the second function, CPDMS, full coverage was achieved maintaining ca. 75% of the first function on the surface (Table 3). Though no displacement of the first function would have been better, these results show that it is possible to covalently bind to the surface the second function, according to the decrease of the Q3 species in 29Si NMR (Fig. 6). Furthermore, 13C NMR (Fig. 5) shows that the surfactant is removed during the CPDMS grafting since a more polar solvent (toluene/acetonitrile) has been used than in previous cases (Table 1). Since most of the TMS is retained, it is likely that the chlorosilane is grafted at the very same place occupied before by the surfactant. This masking effect should be a warrant of homogenous distribution of CPDMS among the TMS functions at a molecular level, as schematised in an ideal manner in Fig. 10.
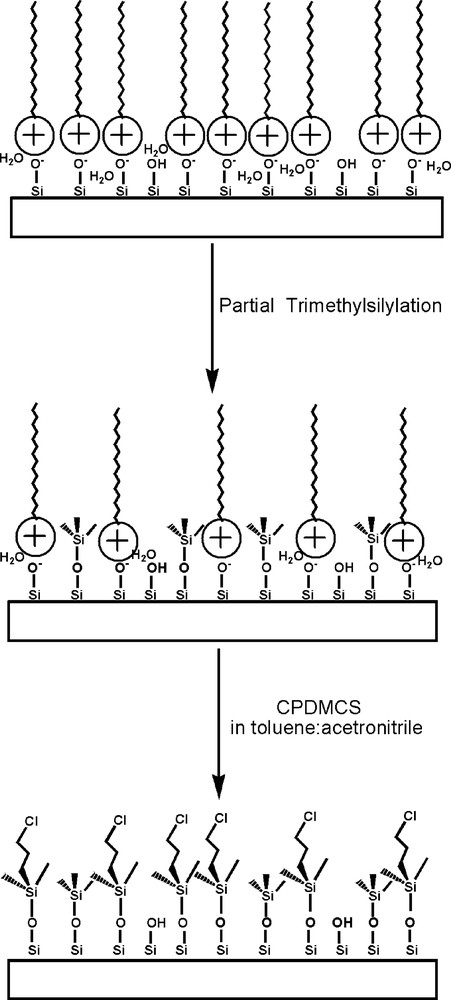
Strategy for dual functionalisation: as-made LUS-1 (top), partially silylated material LUS-PS (middle), dual functionalised silica LUS-PS-CP5 (bottom).
At this point, it is important to figure out how to apply this technique in order to obtain site isolation. The best that can be done is in the first step to fully cover the surface left around each surfactant head group. Surface coverage of each function has to be taken into account. As already mentioned above, TMS covers 0.43 nm2, that is to say, assuming a 2D hexagonal compact coverage (Fig. 11), a diameter of 0.70 nm. Since full coverage is not known with van der Waals contact, the ammonium head group of the surfactant is assumed to be about the size of tetramethylammonium, i.e. 0.67 nm2. This corresponds to a surface coverage of 0.39 nm2, close to the coverage of TMS. Therefore, the site isolation can be foreseen within the 2D hexagonal compact coverage. As shown on Fig. 11, this is achieved when the TMS/surfactant molecular ratio is 2/1, i.e. 67% and 33% molecular coverage for each function, respectively. This condition is not yet fulfilled here but the present study shows that this goal is reasonably achievable.
5 Conclusion
A novel grafting method has been developed which consists to graft two functions on the surface of templated mesostructured silica trying to achieve a molecular control on both neighbouring and distances3. This has been applied using organotriethoxysilanes, organodimethylchlorosilanes or HMDSO for grafting with displacement of the surfactant. Patterning has been obtained by trimethylsylilation in presence of the surfactant whose retention is a key point for the so-called molecular stencil patterning. Site isolation of the second function by the first grafted function can be obtained but still to be achieved. The present work demonstrates that this is a reasonable target to attain. However, such a study is very difficult since highly precise elemental analysis combined with both quantitative 13C and 29Si solid-state NMR is necessary to obtain unambiguous conclusions. Further work is in progress to optimise both the coverage of the first function and the retention of this function during the grafting of the second one. These bifunctional materials will be in a near future used as starting precursors for various applications in catalysis and in adsorption.
Acknowledgments
We thank the analytical services of the Institut des Recherches sur la Catalyse in Villeurbanne, especially Chantal Lorentz and Marie-Thérèse Gimenez. Both CNRS and ‘Région Rhône-Alpes’ (‘Thématique prioritaire’ No. 301439201/E038131747/ENS006) are acknowledged for their financial support.
1 The nitrogen contents of the samples containing traces of surfactant are below 0.1% which is the detection limitation of the elemental analyser.
Surfactant/SiO2a | TMS/SiO2a | CPDMCS/SiO2a | Coverageb | |
% | ||||
LUS-1 | 0.166 ± 0.001 | 0 | 0 | – |
LUS-CP2 | 0.02 ± 0.005c | 0 | 0.19 ± 0.02c | ~83 ± 9 |
LUS-CP2-C | < 0.006d [~0.0054] | 0.046 ± 0.002 | 0.188 ± 0.004 | 102 ± 2 |
LUS-PS | 0.123 ± 0.001 | 0.093 ± 0.002 | 0 | 40 ± 1 |
LUS-PS-CP5 | < 0.006d [~0] | 0.070 ± 0.002 | 0.164 ± 0.002 | 102 ± 2 |
a Molar ratio calculated considering SiO2 content obtained from weight loss at 1273 K and removing the contribution of the SiO2 formed for the grafted silane.
b Assuming that 0.23 function per silica corresponds to 100% coverage.
c Calculated from 29Si and 13C NMR, the other data from elemental analysis.
d Maximal value due to the detection limit of elemental analyser (0.1% in nitrogen). The 13C NMR estimation of this value is in brackets.
2 TMA diameter = 0.668 nm, calculated from Cerius2 molecular modelling (version 3.5) C–N–C angles of 109°54′, N–C bond distances of 0.148 nm, C–H bond distances of 0.110 nm, and hydrogen van der Waals radius of 0.120 nm. (see [24]).

3 This work has been presented at the Fourth International Mesostructured Materials Symposium in Cape Town, South Africa in May 2004.