1 Introduction
Inorganic compounds containing low-valent molybdenum are generally characterized by metal clusters of diverse sizes and geometries. In reduced molybdenum chalcogenides, the Mo clusters can accept a variable number of electrons (often called metallic electrons or ME) available for Mo–Mo bondings. The ME count, which govern the physical properties, can be modified through topotactic reduction–oxidation reactions at low temperatures. This is particularly well exemplified by the silver deinsertion from Ag3.6Mo9Se11 that yield the isomorphous metastable compound o-Mo9Se11 [1]. The latter phase is a superconductor with 32 ME per Mo9 cluster while the parent ternary compound is a semiconductor and have 35.6 ME per Mo9. Previous works have shown that the indium molybdenum cluster chalcogenides such as InMo6S8, In3.7Mo15S19, In3.3Mo15Se19, In2Mo15Se19 or In2Mo6Se6 are also good candidates for topotactic reduction–oxidation reactions at low temperatures [2–6]. Indeed, the indium can be removed easily from the latter ternary compounds by oxidation with I2 or HCl gas to give the corresponding metastable binary compounds. From the binaries thus obtained, new ternary phases can synthesized by electrochemical reactions or by low temperature diffusion of metals having low melting point [3]. By using the latter technique we could prepare the new mercury molybdenum chalcogenides Hg~4.2Mo15X19 (X = S, Se), the syntheses and crystal and electronic structures of which are described in this paper.
2 Experimental section
2.1 Syntheses
The compounds Hg~4.2Mo15X19 (X = S, Se) were obtained in three steps. The first one was the synthesis of the solid-state compounds In3.7Mo15S19 and In3.3Mo15Se19 then the obtaining of the binaries Mo15X19 (X = S, Se) by ‘chimie douce’ and, finally the synthesis of the title compounds by inserting mercury into the Mo15X19 compounds at low temperature. All these steps were performed on single-crystals.
2.1.1 In3.7Mo15S19 and In3.3Mo15Se19
Starting materials used for the synthesis of In3.7Mo15S19 were MoS2, In2S3 and Mo, all in powder form. For In3.3Mo15Se19, the initial products were MoSe2, InSe and Mo. Before use, Mo powder was reduced under H2 flowing gas at 1000 °C during 10 h in order to eliminate any trace of oxygen. The molybdenum disulfide and diselenide were prepared by the reaction of sulfur and selenium, respectively, with H2 reduced Mo in a ratio 2:1 in an evacuated (ca. 10–2 Pa Ar residual pressure) and flame-baked silica ampoule, heated at 800 °C during 2 days. The indium sesquisulfide was obtained by heating at 800 °C stoichiometric amounts of indium metal and sulfur powder in an evacuated sealed silica ampoule. InSe was synthesized from indium and selenium shots heated at 450 °C in an evacuated sealed silica ampoule. All starting reagents were found monophasic on the basis of their powder X-ray diffraction diagram recorded with an Inel curve sensitive position detector CPS 120 using Cu Kα1 radiation. In order to avoid any contamination by oxygen and moisture, the starting reagents were mixed, ground together in a mortar and then cold-pressed in a purified argon-filled glove box. The pellets (ca. 3 g) of the starting reagents were then loaded in molybdenum crucibles (depth: 2.5 cm; diam.: 1.5 cm), which were previously cleaned by heating at about 1500 °C in a high frequency furnace for 15 min under a dynamic vacuum of about 10–3 Pa and then sealed under a low argon pressure (300 h Pa) using an arc welding system. For In3.7Mo15S19, the molybdenum crucible was heated at a rate of 50 °C h–1 up to 1120 °C and held there for 48 h, then cooled at 100 °C h–1 to 1000 °C and finally cooled down to room temperature in the furnace. For the selenide, the temperature of reaction was 1300 °C. The resulting products were black and air-stable and were found as pure phases on the basis of their X-ray powder diffraction diagram.
2.1.2 Mo15S19 and Mo15Se19
Single-crystals of the binary compounds were obtained from oxidation of single-crystals of In3.7Mo15S19 and In3.3Mo15Se19 by iodine in silica tube sealed under vacuum.
The end of the tube containing the crystals of the indium compounds and an excess of iodine was placed in a furnace with about 3 cm of the other end sticking out of the furnace, at about room temperature. The furnace was then heated at 300 °C for 96 h. At the end of the reaction, crystals of InI3 and I2 were obtained at the cool end of the tube.
2.1.3 Hg~4.2Mo15X19 (X = S, Se)
The mercury ternary phases were prepared by diffusion of mercury into crystals of Mo15X19 in silica tube sealed under vacuum at 400 °C during 96 h. In both cases, an excess of mercury was used.
2.2 Single-crystal X-ray studies
The X-ray diffraction data were collected on an Nonius Kappa CCD diffractometer using graphite-monochromated Mo–Kα radiation (λ = 0.71073 Å). The COLLECT program package [7] was employed to establish the angular scan conditions (ϕ and ω scans) used in the data collections. The data sets were processed using EvalCCD [8] for the integration procedure. For both crystals, an absorption correction was applied using the description of the crystal faces and the analytical method described by de Meulenaar and Tompa [9]. The structures were refined using SHELXL-97 [10]. The positions of the Mo and S atoms in In3.7Mo15S19 [2] were used in the first stage of the refinement. The Hg positions were revealed by subsequent difference Fourier syntheses. Refinement of the occupancy factor of the Hg3 atoms led to the following stoichiometries: Hg4.145(6)Mo15S19 and Hg4.278(6)Mo15Se19. Relevant crystallographic data are listed in Table 1, and selected bond distances are given in Table 2.
X-ray crystallographic and experimental data for Hg4.145Mo15S19, and Hg4.278Mo15Se19
Formula | Hg4.145Mo15S19 | Hg4.278Mo15Se19 |
Formula weight (g mol–1) | 2879.90 | 3796.86 |
Space group | P63/m | P63/m |
a (Å) | 9.5368(1) | 9.8856(2) |
c (Å) | 18.5673(3) | 19.2059(3) |
Z | 2 | 2 |
V (Å3) | 1462.46(3) | 1625.44(5) |
ρcalcd (g cm–3) | 6.473 | 7.745 |
T (°C) | 20 | 20 |
λ (Å) | 0.71073 | 0.71073 |
μ (mm–1) | 29.190 | 46.636 |
R1a | 0.0426 | 0.0493 |
wR2b | 0.1027 | 0.1112 |
a R1 = Σ∣∣Fo∣–∣Fc∣∣/Σ∣Fo∣.
b wR2 = {Σ[w(F2o−F2c)2]/Σ[w(F2o)2]}1/2.
Selected interatomic distances for Hg4.145Mo15S19, and Hg4.278Mo15Se19
Hg4.145Mo15S19 | Hg4.278Mo15Se19 | |
Mo1–Mo1 (×2) | 2.6910(4) | 2.6871(14) |
Mo1–Mo1 (×2) | 2.7182(5) | 2.7100(8) |
Mo2–Mo2 (×2) | 2.6819(4) | 2.6714(12) |
Mo2–Mo3 | 2.7123(4) | 2.7200(8) |
Mo2–Mo3 | 2.7493(5) | 2.7674(9) |
Mo3–Mo3 (×2) | 2.7484(7) | 2.7591(13) |
Mo1–Mo2 | 3.2739(5) | 3.4866(9) |
Mo1–X1 | 2.4455(13) | 2.5667(11) |
Mo1–X1 | 2.4466(10) | 2.5816(10) |
Mo1–X1 | 2.4856(15) | 2.6107(14) |
Mo1–X2 | 2.4925(9) | 2.6510(12) |
Mo1–X4 | 2.4331(15) | 2.5440(12) |
Mo2–X1 | 2.5014(9) | 2.6640(12) |
Mo2–X2 | 2.4769(13) | 2.6102(10) |
Mo2–X2 | 2.4374(15) | 2.5736(10) |
Mo2–X3 | 2.5760(6) | 2.6665(10) |
Mo2–X5 | 2.4127(13) | 2.5338(11) |
Mo3–X2 (×2) | 2.4442(11) | 2.5930(8) |
Mo3–X3 | 2.4518(13) | 2.574(2) |
Mo3–X3 | 2.4570(12) | 2.574(2) |
Hg1–X1 (×3) | 3.3218(7) | 3.4211(10) |
Hg1–X2 (×3) | 3.2035(12) | 3.2485(10) |
Hg1–X5 | 2.5618(17) | 2.6974(13) |
Hg2–X3 (×3) | 3.5129(15) | 3.6247(9) |
Hg2–X2 (×6) | 3.7953(11) | 3.8857(9) |
Hg3–X2 (×2) | 2.7369(11) | 2.7124(11) |
Hg3–X3 | 2.4276(13) | 2.561(2) |
Hg3–X4 (×2) | 2.7403(14) | 2.9577(14) |
Hg1–Hg2 (×2) | 2.6356(3) | 2.6441(6) |
2.3 Theoretical calculations
Calculations have been carried out within the extended Hückel formalism [11,12] with the program YAeHMOP [13]. The exponents (ξ) and the valence shell ionization potentials (Hii in eV) were (respectively): 1.817, –20.0 for S 3 s; 1.817, –13.3 for S 3p; 1.956, –8.34 for Mo 5 s; 1.921, –5.24 for Mo 5p; 2.649, –13.68 for Hg 6 s; 2.631, –8.47 for Hg 6p. Hii values for Mo 4d and Hg 5d were set equal to –10.50 and –17.50, respectively. Linear combination of two Slater-type orbitals of exponents ζ1 = 4.542 and ζ2 = 1.901 with the weighting coefficients c1 = c2 = 0.5898, and ζ1 = 6.436 and ζ2 = 3.032 with the weighting coefficients c1 = 0.6438 and c2 = 0.5215 were used to represent the Mo 4d and Hg 5d atomic orbitals, respectively. The density of states (DOS) and Crystal Orbital Overlap Populations (COOP) were obtained using a set of 18 k points.
2.4 Magnetic measurements
The magnetic susceptibility was measured using a SQUID magnetometer (MPMS-XL, Quantum Design).
3 Results and discussion
3.1 Crystal and electronic structures
A view of the crystal structure of Hg4.15Mo15S19 is shown in Fig. 1 as example. The molybdenum-chalcogen frameworks of the Hg~4.2Mo15X19 (X = S, Se) compounds are similar to those of the parent compounds In3.7Mo15S19 and In3.3Mo15Se19. They consist of an equal mixture of and (X = S, Se) cluster units linked through interunit Mo–X bonds (Fig. 2). The first unit is similar to that encountered in the ternary molybdenum chalcogenides MxMo6X8 (M = Na, K, Ca, Sr, Ba, Sn, Pb, rare earth metal, 3d element; X = S, Se or Te), known as Chevrel phases. It corresponds to an Mo6 octahedron surrounded by 8 face-capping inner (6 X1 and 2 X4) and 6 apical (X2) ligands. The Mo9 core of the second unit results from the face sharing of 2 octahedral Mo6 clusters. The Mo9 cluster is surrounded by 11 (6 X2, 3 X3 and 2 X5) atoms capping the faces of the bioctahedron and 6 apical (X1) ligands above the ending Mo atoms. In the sulfide compound, the Mo–Mo distances within the Mo6 clusters are 2.6910(4) Å (2.6871(14) in the selenide) for the intra-triangle distances (distances within the Mo3 triangles formed by the Mo atoms related through the threefold axis) and 2.7182(5) Å (2.7100(8) in the selenide) for the inter-triangle distances. The Mo–Mo distances within the Mo9 clusters are 2.6819(4) and 2.7484(7) Å (2.6714(12) and 2.7591(13) Å in the selenide) for the intra-triangle distances between the Mo2 and Mo3 atoms, respectively, and 2.7123(4) and 2.7493(5) Å (2.7200(8) and 2.7674(9) Å in the selenide) for those between the Mo3 triangles. The and units are centered at 2b and 2c positions and have the point-group symmetry and 3/m, respectively. The chalcogen atoms bridge either one (X1, X2, X4 and X5) or two (X3) Mo triangular faces of the clusters. Moreover the chalcogens X1 and X2 are linked to a Mo atom of a neighboring cluster. The Mo–X bond distances range from 2.4167(11) to 2.5032(14) Å within the Mo6X8 unit and from 2.3908(8) to 2.6262(11) Å within the Mo9X11. Each unit is interconnected to 6 units (and vice-versa) via interunit Mo1–X2 bonds (respectively, Mo2–X1) (Fig. 2) to form the three-dimensional Mo–X framework, the connectivity formula of which is . It results from this arrangement that the shortest intercluster Mo1–Mo2 distance between the Mo6 and Mo9 clusters is 3.2739(5) Å in the sulfide and 3.4866(9) Å in the selenide, indicating only weak metal–metal interaction.
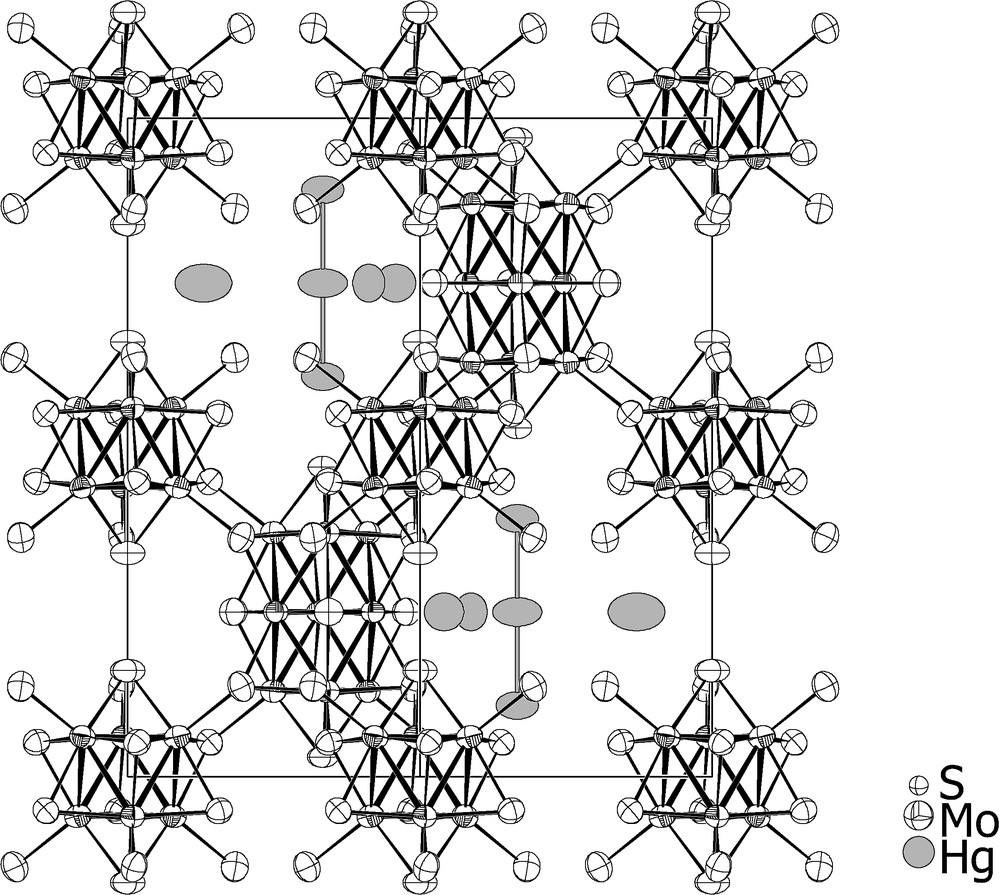
View of the crystal structure of Hg4.145Mo15S19, (97% probability ellipsoids).
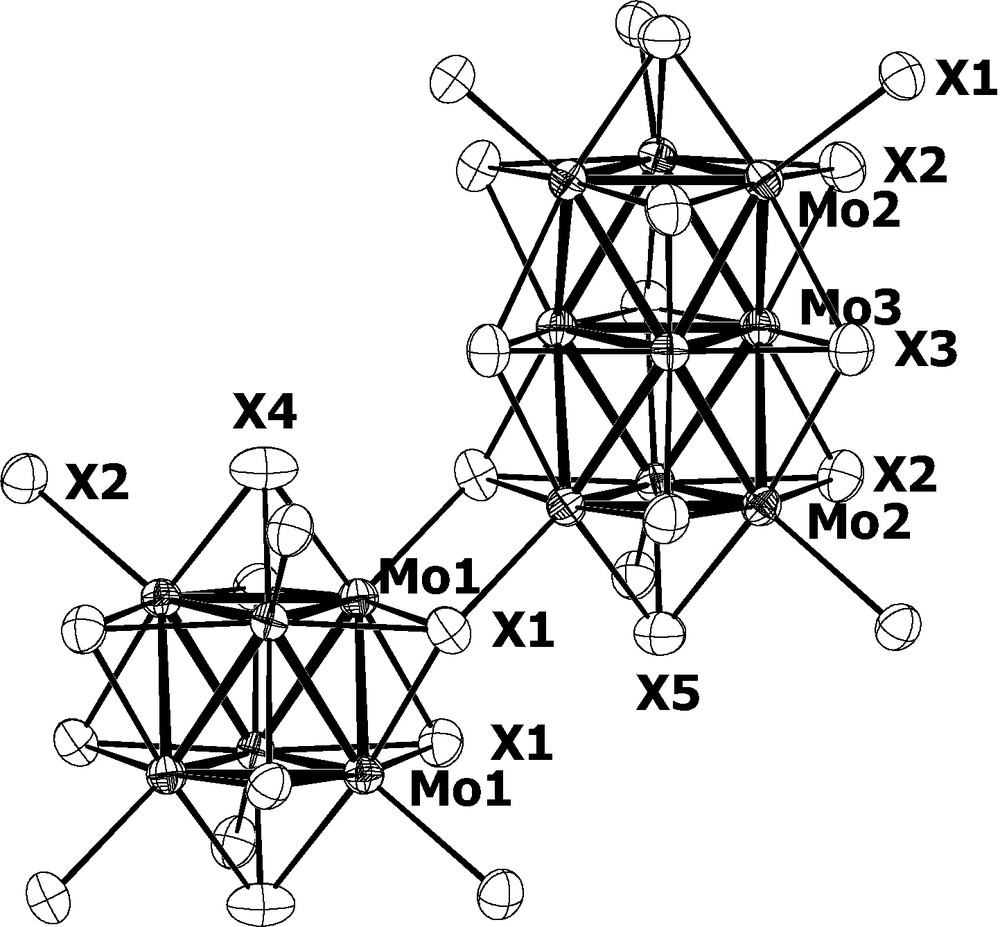
The Mo6S8S6 and Mo9S11S6 units.
In both compounds, the mercury is present either as Hg2+ cations (ie Hg3) or forming linear trinuclear clusters Hg32+units (i-.e. Hg1 and Hg2) (Fig. 3).. The Hg2+ cations occupy the sites in which the In3+ cations are located in the indium parent compounds (Fig. 3). The latter position corresponds to a triangular group of distorted octahedral cavities around the threefold axis, which are formed by two Mo6X8 and three Mo9X11 units. These cavities are only partially occupied with 1.145(6) and 1.278(6) Hg2+ cations per triangular group for the sulfide and selenide compounds, respectively. The Hg3–S distances range from 2.4276(13) to 2.7403(14) Å and, the Hg3–Se ones from 2.561(2) to 2.9577(14) Å.
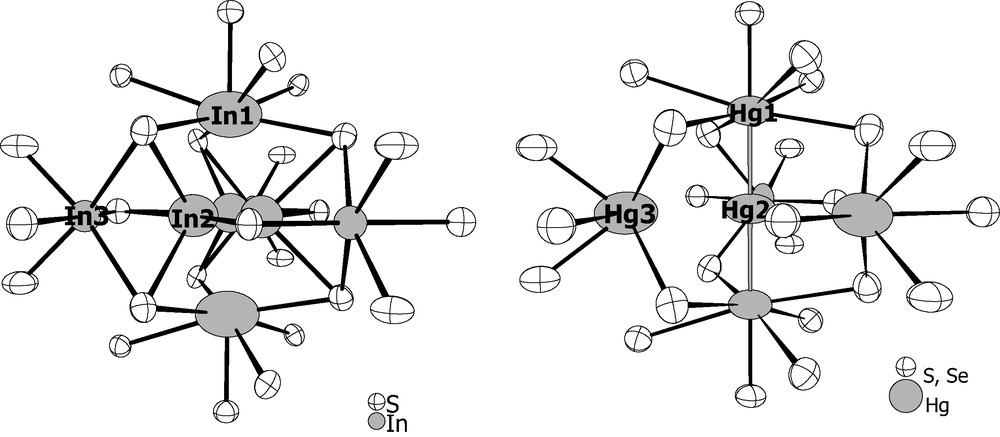
Views of the In and Hg sites in In3.7Mo15S19, (left) and Hg~4.2Mo15X19 (right).
The Hg32+ cluster, which is for the first time observed in a chalcogen environment, resides in the two large cavities that are occupied by the monovalent indium cations related through the mirror plane in In3.7Mo15S19 and In3.3Mo15Se19. The Hg32+ cluster is linear and centrosymmetrical with Hg1–Hg2 distances of 2.6356(3) Å in the sulfide and 2.6441(6) Å in the selenide (Fig. 4). The latter distances are somewhat longer that those observed in the other inorganic compounds such as Hg3(AsF6)2 [14], Hg3(AlCl4)2 [15], Hg3(NbF5)2(SO4)2 [16] and Hg3(TaF5)2(SO4)2 [17] containing quasi- or linear Hg32+ groups in which the Hg–Hg distances range from 2.551 to 2.562 Å. The coordination environment about the mercury atoms is also different. The environment of the terminal mercury atoms Hg1 is similar to that of the monovalent indium cations and thus consists of seven chalcogen atoms. The Hg1–X distances range from 2.5618(17) to 3.3218(7) Å in the sulfide and, from 2.6974(13) to 3.421(1) Å in the selenide. The shortest Hg1–X5 bond is collinear with the Hg–Hg bonds and is, most likely, covalent in character. The central mercury atoms Hg2 is surrounded by nine chalcogen atoms forming a tricapped trigonal prism. The distances between the Hg2 mercury and the capping chalcogen atoms are 3.5129(15) Å and 3.6247(9) Å for the sulfide and selenide, respectively, while the other distances between Hg2 and the chalcogens forming the trigonal prism are 3.7953(11) and 3.8857(9), respectively. Hg1–Hg2 distances are consistent with the presence of metal–metal bonding in the Hg3 unit. The expected metallic electron (ME) count for linear Hg3–X2 units with two metal–metal bonds is 34 [17]. Such a ME count induces a + 2 charge on the Hg3 cluster. This oxidation state is also the one observed for the Hg3 clusters present in the inorganic compounds mentioned above. Considering Hg2+ and Hg32+ cations in the title compound, the total ME count of Mo6 and Mo9 clusters is equal to 56.4. Previous theoretical studies have shown that optimal ME counts, i.e. complete filling of Mo–Mo bonding and non-bonding levels separated from Mo–Mo antibonding levels by a significant energy gap, are equal to 24 and 36 for such Mo6 and Mo9 clusters, respectively [18,19]. Assuming weak interaction between the clusters in the solid-state structure, band structure of the title compound showing a significant energy gap for the ME count of 24 + 36 = 60 is expected. Total and Mo9 projected density of states (DOS) sketched in Fig. 5 were computed for the model compound Hg4Mo15S19 where only one of the three Hg3 equivalent crystallographic position is occupied. The Fermi level for the ME count of 56 (that corresponds to the presence of four Hg atoms in the unit cell) cuts a narrow peak of DOS mainly centered on d orbitals of the Mo6 cluster. Metallic properties can be envisioned for the title compound. This was confirmed by magnetic susceptibility measurements that were carried out on batches of single-crystals of about 100 mg in an applied field of 20 Oe using a dc SQUID magnetometer. Indeed, Hg4.145Mo15S19 and Hg4.278Mo15Se19 show a diamagnetic shielding signal below 4 K indicating a superconducting transition. Finally, it should be mentioned that a band gap of 0.20 eV separating overall Mo–Mo bonding and non-bonding bands from Mo–Mo antibonding bands is reached for the ME count of 60. Such an electron count would render the material semiconducting through suitable doping. Indeed, Mo–Mo and Mo–S overlap populations hardly change overall upon additions of electrons. Analyses of the COOP (not shown here) indicate that the intercluster Mo1–Mo2 and intra Mo9 cluster Mo3–Mo3 should slightly lengthen, as well as Mo–S distances, whereas other Mo–Mo bonds of Mo6 and Mo9 units should slightly shorten when electrons are added.
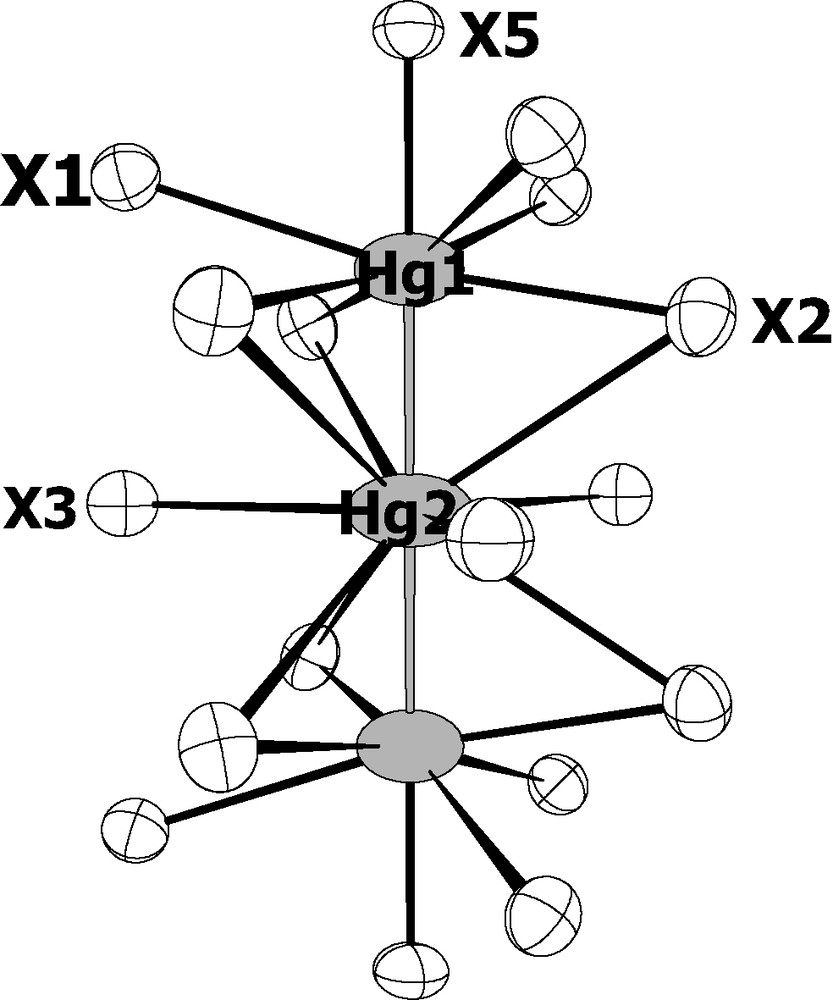
The Hg3 cluster with its chalcogen environment.
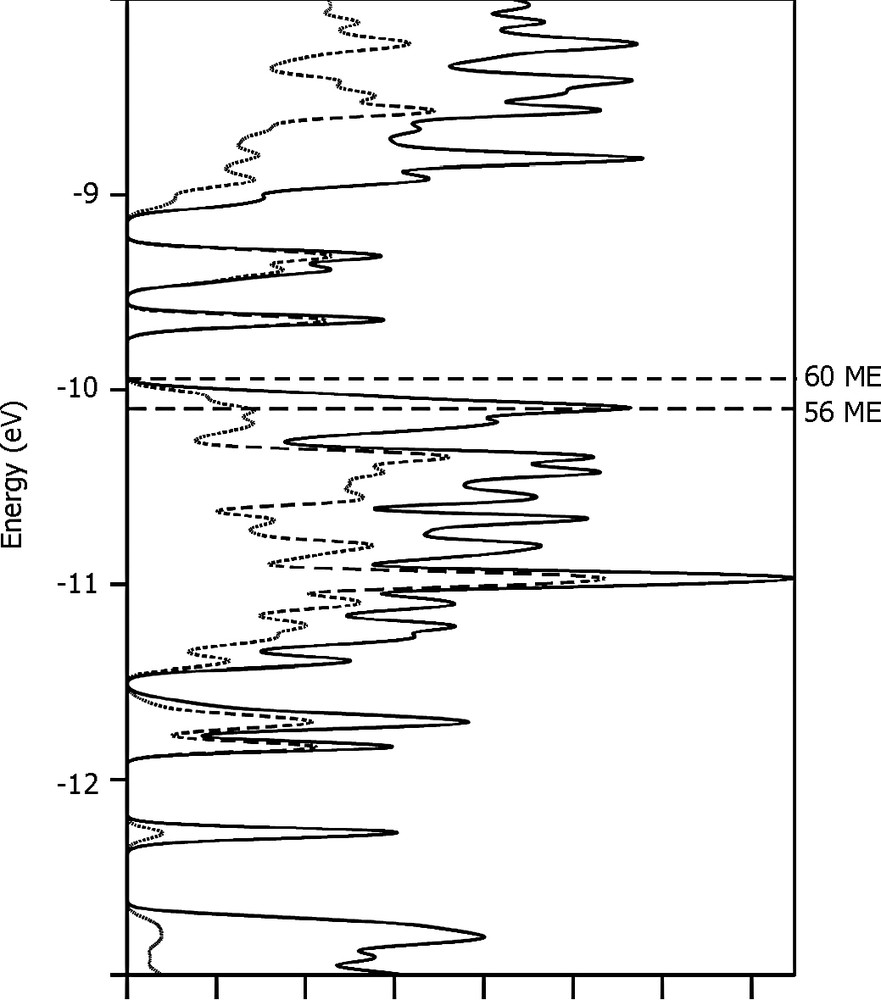
EHTB calculations for Hg4Mo15S19: total density of states (plain) and Mo9 contribution (dotted).
4 Supplementary material
Further details of the crystal structure investigation may be obtained from the Fachinformationszentrum Karlsruhe, D-76344 Eggenstein-Leopoldshafen, Germany (fax: +49 7247 808 666; e-mail: crysdata@fiz-karlsruhe.de), on quoting the depository number CSD-414276 and CSD-414277.