1 Introduction
Starting in the late 1960s, we have been involved in an unfolding story whose main character is the fascinating material titanium dioxide (TiO2). This story, whose keywords are TiO2 and light, began with photoelectrochemical solar energy conversion, and then shifted into the area of environmental photocatalysis and photo-induced hydrophilicity, and most recently into the commercialization of TiO2-based photocatalytic products. In this account paper, we will briefly trace the development of TiO2 photocatalysis on the base of our own contributions, summarize the present commercialized TiO2-based products, and highlight the future approaches for TiO2 photocatalysis.
2 Fundamental studies
2.1 Discovery: water photo-splitting on TiO2 electrodes
In the late 1960s, one of the authors of this paper (AF) began to study oxide semiconductors that would respond to light, while he was still a graduate student at the University of Tokyo. In those days, the study of the photocurrent response of ZnO semiconductor electrodes in aqueous solution was very active in Germany and the US [1]. Soon, it became apparent that the chemical reaction that was responsible for the flow of current was the dissolution of the zinc oxide itself. About that time, the author was able to obtain a single crystal of the rutile form of titanium dioxide, another semiconductor. The crystal was transparent, like glass, and most importantly, it was an extremely stable material in the presence of aqueous electrolyte solutions. The author (A.F.), connected a complete electrochemical circuit, with titanium dioxide and platinum black as the two electrodes, as illustrated in Fig. 1. When the surface of the TiO2 electrode was irradiated with light (λ < 415 nm), photocurrent flowed from the platinum counter electrode to the TiO2 electrode through the external circuit. The direction of the current revealed that the oxidation reaction (oxygen evolution) occurred at the TiO2 electrode and the reduction reaction (hydrogen evolution) at the Pt electrode [2]. This fact shows that water can be decomposed, using UV–visible light, into oxygen and hydrogen, without the application of an external voltage, according to the following equations:
(1) |
(2) |
(3) |

Schematic diagram of an electrochemical photocell. (1) n-type TiO2 electrode; (2) platinum back counter electrode; (3) ionically conducting separator; (4) gas buret; (5) load resistance and (6) voltmeter.
The overall reaction is:
(4) |
This study attracted worldwide attention, since it demonstrated the possibility of generating hydrogen as a clean energy source from water and solar irradiation. Further, the study on water photo-splitting was extended to the heterogeneous photocatalytic approach, i.e. essentially using photoelectrochemistry but without an external circuit. Photocatalytic water splitting has been studied intensively with TiO2 suspensions, with Pt deposited on the TiO2 as a cathodic catalyst [3]. This field remains active, with novel layered metal oxides being the favored materials [4].
2.2 Photocatalysis
Ever since 1977, when Frank and Bard first examined the possibilities of using TiO2 to decompose cyanide in water [5], there has been increasing interest in environmental applications [6,7]. TiO2 is close to being an ideal photocatalyst in several respects. For example, it is relatively inexpensive, highly stable chemically, and the photogenerated holes are highly oxidizing. As shown in Fig. 2, the redox potential for photogenerated holes is +2.53 V versus the standard hydrogen electrode (SHE) in pH 7 solution. After reaction with water, these holes can produce hydroxyl radicals (•OH), whose redox potential is only slightly decreased. Both are more positive than that for ozone. The redox potential for conduction band electrons is –0.52 V, which is negative enough to reduce dioxygen to superoxide, or to hydrogen peroxide. Depending upon the exact conditions, the holes, •OH radicals, O2–, H2O2 and O2 itself can all play important roles in the photocatalytic reaction mechanisms.
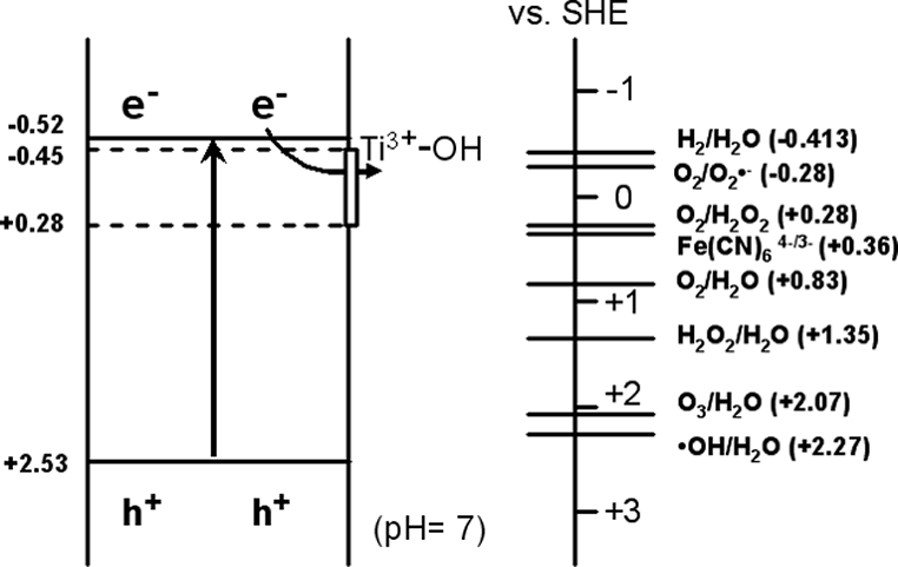
Schematic diagram showing the potentials for various redox processes occurring on the TiO2 surface at pH 7.
We started our research on photocatalytic environmental purification in the early 1990s. Besides the fundamental studies, we have been very interested in the practical environmental applications of TiO2 photocatalysis. Thus, our research has been focused on the photocatalytic processes and mechanisms on immobilized TiO2 photocatalysts (glass, tile, and paper, etc.) under weak UV illumination in the range from 1 μW cm–2 to 1 mW cm–2 [8–13]. We have preferred weak UV illumination in our studies, since the ultraviolet light in direct sunlight is generally 2–3 mW cm–2 in Japan, and that in a reasonably well-lit room is approximately 1 μW cm–2. Some interesting and important results have been reported during our studies, and these will be described briefly below; most are closely related to practical applications.
The first example we show here is the removal of indoor odors by immobilized TiO2 films under weak UV illumination. Odors that are objectionable to humans are due to compounds which are present only on the order of 10 parts per million by volume (ppmv). We found that, at these concentrations, weak UV light of 1 μW cm–2 was sufficient to decompose such compounds when TiO2 photocatalysts were present. Actually, the quantum yield for a simple photocatalytic reaction, e.g., 2-propanol oxidation, on a TiO2 film in ambient air, reached a maximum value when the light intensity was extremely low, as shown in Fig. 3 [14]. The maximum quantum yield observed was ~28% for 1000 ppmv 2-propanol under tens of nW cm–2 UV illumination. The reported maximum QY for acetaldehyde photocatalytic decomposition was even greater than 100%, due to the existence of a radical chain-type process [15]. Thus, it is even possible to keep indoor air clean with TiO2-containing wallpaper under the illumination of fluorescent lamps.
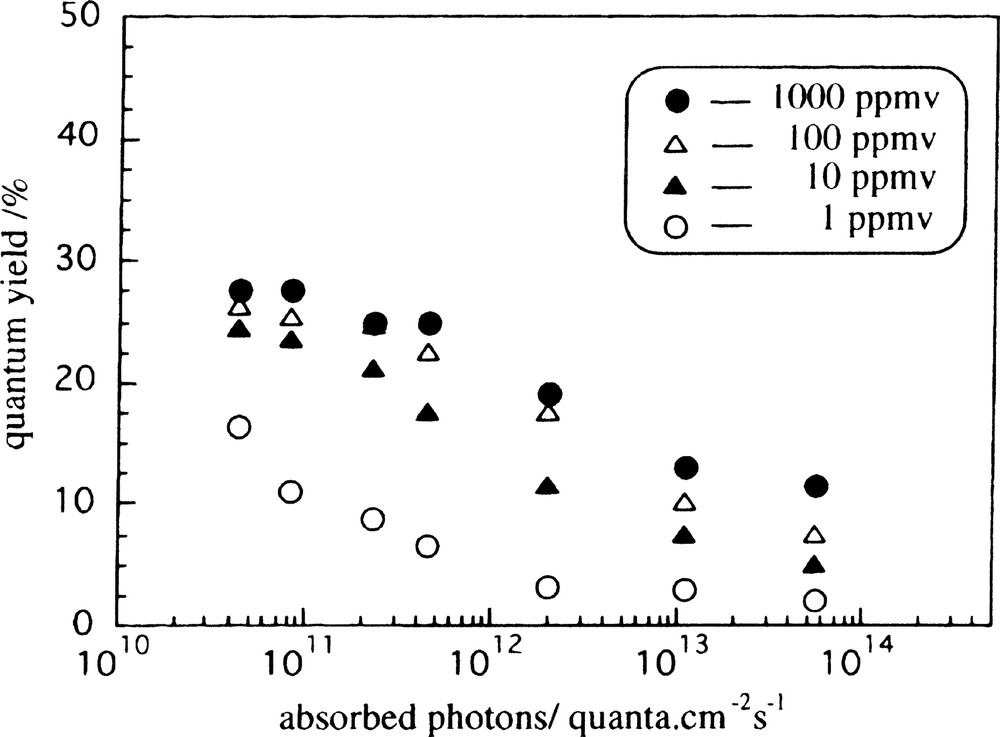
Quantum-yield dependence on absorbed photons at various initial 2-propanol concentrations [17].
The second example we show here is the antibacterial effect of TiO2 under weak UV illumination. In a typical experiment, 150 μl of an E. coli suspension, containing 3 × 104 cells was placed on an illuminated TiO2-coated glass plate (1 mW cm–2). Under these conditions, there were no surviving cells after only 1 h of illumination [16]. By contrast, after 4 h under UV illumination without a TiO2 film, only 50% of the cells were killed. In addition, the dead cells could be decomposed completely by the TiO2 photocatalyst [17]. TiO2-based antibacterial products, such as tiles, fibers, and sprays, etc., have been commercialized in Japan. Antibacterial tiles have been applied to the floors and walls of several hospital operating rooms in Japan, where sterile conditions are crucial. After installing the tiles, the bacterial counts on the walls decreased to negligible levels in a period of 1 h. Surprisingly, the bacterial counts in the surrounding air also decreased significantly [8].
The third example we show here is a self-cleaning TiO2 surface under weak UV illumination. In our study, we found that organic compounds, such as octadecane, glycerol trioleate, and poly (ethylene glycol) (MW 500 000), could be decomposed completely on the TiO2 surface under weak UV illumination (1 mW cm–2) at significant rates, with the evolution of CO2 as the only detectable gas-phase product [18]. This observation is important, because it shows that the TiO2 surface has the ability to self-regenerate. Most soilage of the interiors of buildings comes from organic substances. Photocatalysts are not especially useful for breaking down large volumes of soilage, but they are capable of destroying it as it accumulates. TiO2 photocatalysts thus hold great potential as quiet, unobtrusive self-cleaning materials.
The fourth example we show here is the photocatalytic decomposition of endocrine-disrupter chemicals (EDCs) in water. Natural estrogens of 17β-estradiol (E2) and estron (E1) are basic female sex hormones and are well known to exhibit estrogenic activities potently, even at a very low concentrations (~ 10–9 M). Recently, they have been reported to be important EDCs in the aquatic environment, which may be associated with increased incidences of hermaphrodite carp and trout in British rivers that receive significant inputs of domestic effluents [19]. We found that the two compounds (E1 and E2) dissolved in water could be decomposed completely by TiO2 photocatalysts under weak illumination [20,21]. Most importantly, the intermediates produced during photocatalytic reactions did not exhibit any potent estrogenic activity in the treated water. We designed a photocatalytic reactor using TiO2-modified-PTFE mesh sheets as photocatalysts, and applied this to treat the water discharged from the Kitano sewage treatment plant at the Tama River near Tokyo. Concentrations of E1 and E2 in the discharged water were 140 and 15 ng l−1, respectively. Under UV illumination (1.2 mW cm–2), about 90% of the initial E1 was decomposed in a short time, with very good reproducibility, as shown in Fig. 4 [21]. Thus, TiO2 photocatalysis can be applied in water treatment as a novel method for removing natural and synthetic estrogens effectively, without generating biologically active intermediates.
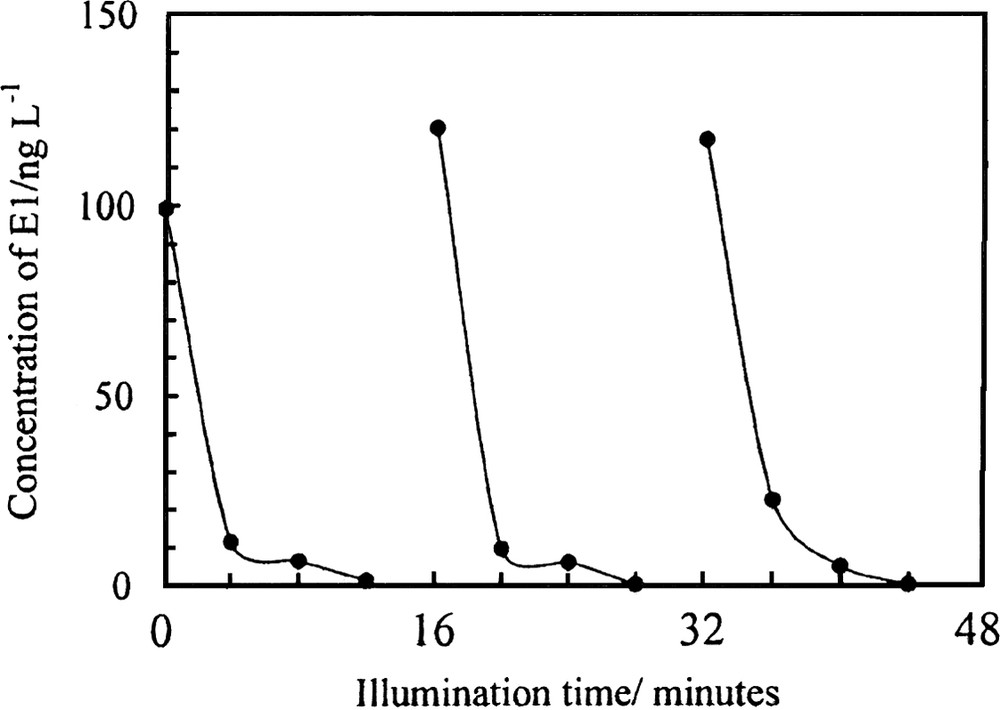
Concentration change of E1 in discharged water from a sewage treatment plant during photocatalysis [21]. The measurement was repeated three times (UV light intensity, 1.2 mW cm–2; water temperature, 15 °C).
2.3 Photo-induced superhydrophilicity
The superhydrophilicity of TiO2 was actually discovered by accident in work that was being carried out at the laboratories of TOTO Inc., in 1995. It was found that, if a TiO2 film was prepared with a certain percentage of SiO2, it acquired superhydrophilic properties, i.e. a water contact angle of ~ 0° after UV illumination. In further study, we found that the photo-induced superhydrophilicity was an intrinsic property of the TiO2 surface, as shown in Fig. 5 [22,23]. The mechanism for this process was proposed on the basis of the reconstruction of the surface hydroxyl groups under UV irradiation [24]. As illustrated in Fig. 6, photoexcited electrons are captured by molecular oxygen, while the holes diffuse to the TiO2 surface, being trapped at lattice oxygen atoms. Subsequently, the hole trapping weakens the binding energy between the Ti atom and the lattice oxygen, and another adsorbed water molecule breaks this bond, forming new hydroxyl groups. In the dark, the hydroxyl groups gradually desorb from the surface in the form of H2O2 or H2O + O2.
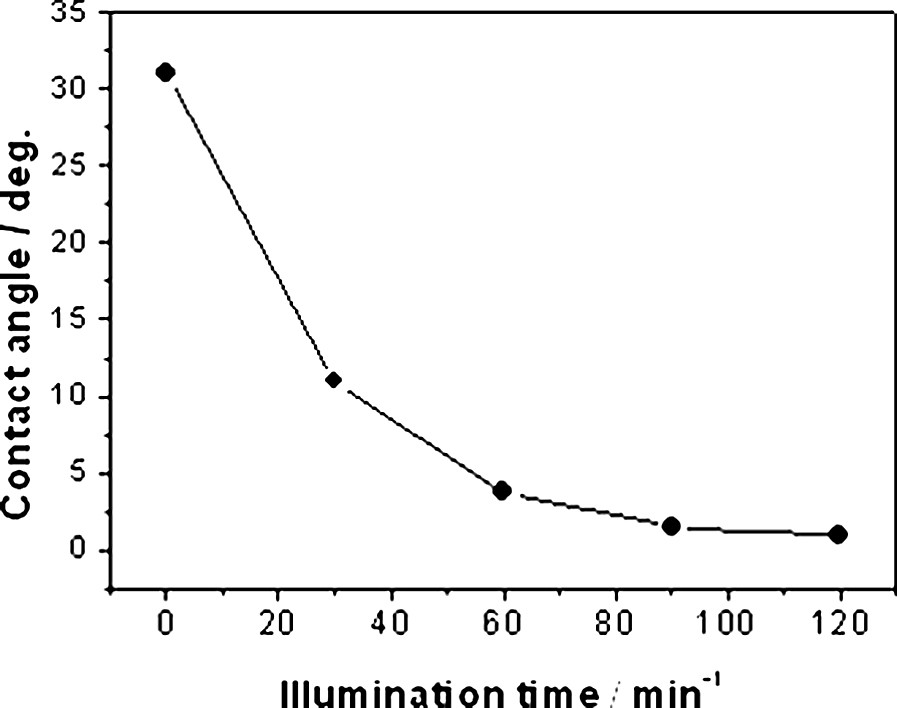
Water contact angle as a function of time under UV illumination (1.1 mW cm–2) for a polycrystalline TiO2 film on glass.
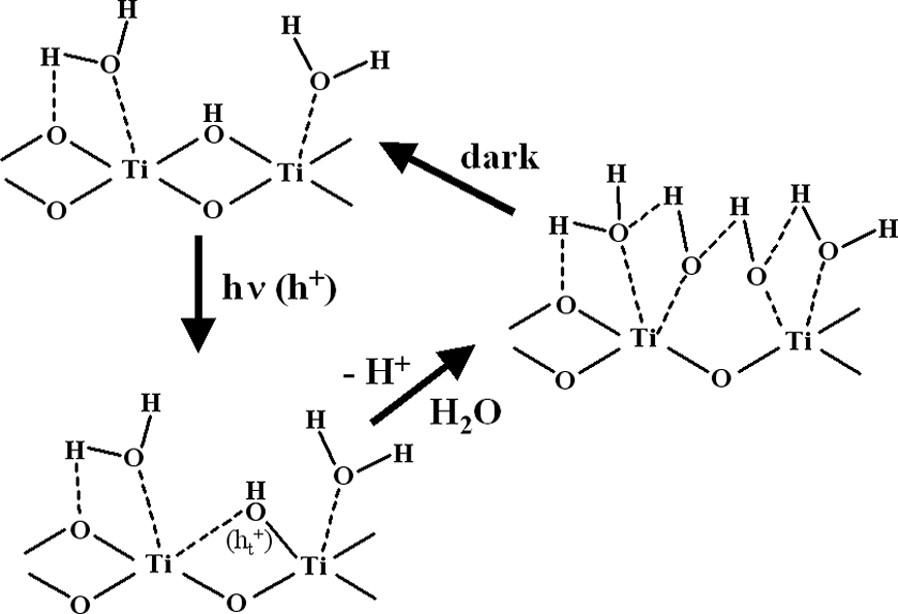
Surface reconstruction on TiO2 during the reversible hydrophilic changes [24].
The photo-induced hydrophilic conversion is a different process from the photocatalytic decomposition of organic contaminants. Strontium titanate, which has almost the same photocatalytic oxidation power as TiO2, does not become hydrophilic by means of UV irradiation [25]. However, WO3, which shows a photo-induced hydrophilic conversion, does not exhibit photocatalytic activity [26]. It is one of the unique aspects of TiO2 that there are actually two distinct photo-induced phenomena, i.e. the photocatalytic phenomenon and the photo-induced superhydrophilic phenomenon. Even though they are intrinsically different processes, they can, and in fact must, take place simultaneously on the same TiO2 surface. Depending on the composition and the processing, the surface can have more photocatalytic character and less superhydrophilic character, or vice versa.
There is an extremely wide range of applications for superhydrophilic technology. For example, an antifogging surface can be prepared by the superhydrophilic effect. Fogging of the surfaces of mirrors and glass occurs when humid air condenses, with the formation of many small water droplets, which scatter light. On a superhydrophilic surface, no water droplets are formed. Instead, a uniform film of water can from on the surface, and this film does not scatter light. The superhydrophilic property also helps the self-cleaning process on TiO2, which will be discussed in the next section.
3 Present situation of TiO2 photocatalysis
3.1 General
The commercialization of TiO2-based photocatalytic products commenced in the mid-1990s in Japan. However, this industry has grown very quickly, and its market reached ca. 30 billion Japanese yen in Japan in 2003. More than 2000 companies have joined in this new industry, whose products can be mainly divided into five categories, i.e. exterior construction materials, interior furnishing materials, road construction materials, purification facilities, and household goods, etc., as shown in Table 1. Among the five main categories, the self-cleaning exterior construction materials occupied the biggest market share, as depicted in Table 2. However, the market share of purification facilities grew at the highest rate, from 9% in 2002 to 33% in 2003, which was mainly due to the contribution of air purification products, including air cleaners and air conditioners. We will emphasize the above two categories in the next section.
TiO2-based photocatalytic products that have appeared on the market in Japan
Categories | Products | Properties |
Exterior construction materials | Tiles, glass, tents, plastic films, aluminum panels, coatings, | Self-cleaning |
Interior furnishing materials | Tiles, wallpaper, window blinds, | Self-cleaning, antibacterial |
Road-construction materials | Soundproof walls, tunnel walls, road-blocks, coatings, traffic signs and reflectors, lamp covers | Self-cleaning, air-cleaning |
Purification facilities | Air cleaners, air conditioners, purification system for wastewater and sewage, purification system for pools | Air-cleaning, water-cleaning, antibacterial |
Household goods | Fibers, clothes, leathers, lightings, sprays | Self-cleaning, antibacterial |
Others | Facilities for agricultural uses | Air-cleaning, antibacterial |
Market share of the photocatalytic products by categories in 2 recent years
Year | 2002 (%) | 2003 (%) |
Exterior construction materials | 61 | 44 |
Interior furnishing materials | 20 | 13 |
Road-construction materials | 6 | 4 |
Purification facilities | 9 | 33 |
Household goods | 4 | 5 |
Others | – | 1 |
3.2 Self-cleaning exterior construction materials
TiO2-based self-cleaning exterior products, including tiles, glass, tents, etc., have been widely applied in Japan. Such products can keep clean by the action of sunlight and rainwater, based on the photocatalytic and superhydrophilic properties of TiO2. That is, as shown in Fig. 7, gradually adsorbed organic soilage such as oil is decomposed by the photocatalytic property of TiO2, while organic contaminants and dust can be washed off by rainwater because of the superhydrophilic property of TiO2. It is not true that a superhydrophilic self-cleaning surface will never turn dirty, since the self-cleaning process is dependent on the illumination conditions of sunlight, the amount of rainfall, and the accumulation rate of soilage, etc. But it is really true that such a self-cleaning surface will retard the rate of contamination, and thus save a lot of time and cost for cleaning maintenance, which is very difficult for high buildings and flexible plastic materials (e.g. domes, canopies and tents).

Schematic diagram of the decontamination process occurred on the superhydrophilic self-cleaning surface.
TOTO Inc. is the main producer of TiO2-based self-cleaning tiles in Japan. Its self-cleaning tiles have been applied on more than 5000 buildings in Japan according to statistical data for 2003. Among these, the most famous building is the Maru building, which lies in the central business district of Tokyo. As shown in Fig. 8, the self-cleaning tiles are resistant to staining from water that runs off a roof. Generally, the common tile-covered building has to be cleaned every 5 years. However, the self-cleaning tiles are predicted to stay clean for more than 20 years by TOTO Inc., and thus save much of the cost for maintenance. In addition, self-cleaning tiles can decompose air pollutants such as nitrogen oxides (NOx), and reduce the amounts of detergents used; therefore, it is truly an environmentally benign construction material.

Photograph showing alternating self-cleaning (A) and ordinary (B) exterior wall tiles that have been placed so that water from a corrugated metal roof runs off onto the wall [23].
TiO2-based self-cleaning glass is also an important commercial self-cleaning product. Such glass can maintain extreme visual clarity even on a rainy day, since water does not bead, but instead spreads evenly across the surface. If the amount of water is relatively small, the water layer becomes very thin and evaporates quickly. If the amount of water is larger, it forms a sheet-like layer that also has high visual clarity. It may be surprising that TiO2-coated glass can maintain the light transmittance properties of common glass, since the higher refractive index of TiO2 enhances the surface reflection. Actually, TiO2 nanoparticles are dispersed in a SiO2 matrix in the coating for self-cleaning glass. The composition of the coating is carefully controlled so that its refractive index is close to that of glass.
Another important TiO2-based self-cleaning product is self-cleaning polymer film materials. Taiyo Kangyo Co. is the main manufacturer of self-cleaning tents for storage uses in Japan. The tent material, made from flexible poly (vinylchloride) film, is difficult to clean. Coating the PVC film with a TiO2 layer can resolve the soilage problem. However, an intermediate layer with an inorganic-organic graded structure is necessary to avoid the photodegradation of the PVC film by the TiO2 coating. Based on this technique, a self-cleaning tent showed high stability during a 4-year outdoor exposure test.
3.3 Uses in air cleaners
Another important application of TiO2 photocatalysts is in the purification of indoor air. Malodorous substances such as ammonia, hydrogen sulfide, acetaldehyde, toluene, methyl mercaptan, etc., involve serious risks to health or comfort. Their concentrations in indoor air are always low, which is very suitable for TiO2-based air purification. A photocatalyst-type air cleaner is typically composed of TiO2-based filters, UV lamps, and a fan for air circulation. The filters feature honeycomb-type construction or three-dimensional porous structure for minimum pressure drop. Fig. 9 shows a picture of TiO2-coated porous ceramics filters for air cleaner uses. TiO2 nanoparticles are coated on or dispersed in the body of filters with active carbon, zeolite, etc., as co-adsorbents. In contrast to the single function of adsorption for active carbon filters in conventional air cleaners, the TiO2-based photocatalyst filter can decompose the adsorbed pollutants instead of accumulating them, and thus it exhibits better air-cleaning performance, as shown in Fig. 10. In addition, the photocatalyst-type air cleaner can also kill the bacteria floating in indoor air, which is very important for the applications in hospitals, institutions for the elderly, and schools, etc.
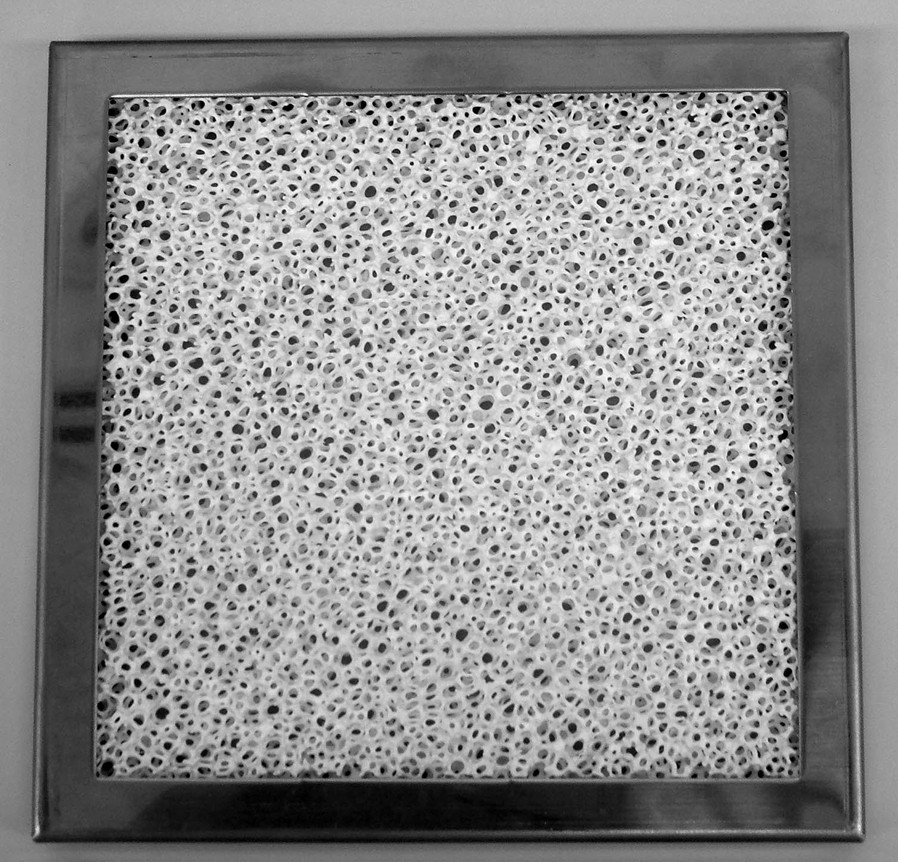
Photograph of TiO2-based porous ceramics filters for air cleaner uses.

Example of cleaning nitrogen oxide (NOx) in a typical apartment room. Curve a: natural decrease of NOx in room; curve b: NOx concentration change when an air cleaner containing only activated carbon adsorbent was working; curve c: NOx concentration change when an air cleaner containing irradiated photocatalyst on activated carbon was working; curve d: environmental standard for NOx (data courtesy of Daikin Industries, Ltd.).
The air cleaners are available in various sizes, which have been developed to clean the air in a car or in an entire factory or hospital. After long-term use, the filter may be poisoned by HNO3 or H2SO4 formed during removal of ammonia or hydrogen sulfide. The poisoned filter can be regenerated by simple washing with water. Now, there are more than 30 companies manufacturing photocatalyst-type air cleaners in Japan. Some air-conditioners have also been equipped with TiO2 photocatalytic filters.
4 Future perspectives
4.1 Visible-light photocatalysts
One of the major challenges for the scientific community involved in photocatalytic research is to increase the spectral sensitivity of photocatalysts to visible light, which composes the largest part of solar radiation. Anatase TiO2, which is the most photoactive phase of TiO2, only absorbs ultraviolet light with wavelengths shorter than 380 nm, as shown in Fig. 11. However, the UV part of direct sunlight is generally in the range of 2–3 mW cm−2 in Japan. The content of ultraviolet light in indoor illumination is significantly smaller than that in sunlight, because the fluorescent lamp mainly emits visible light.
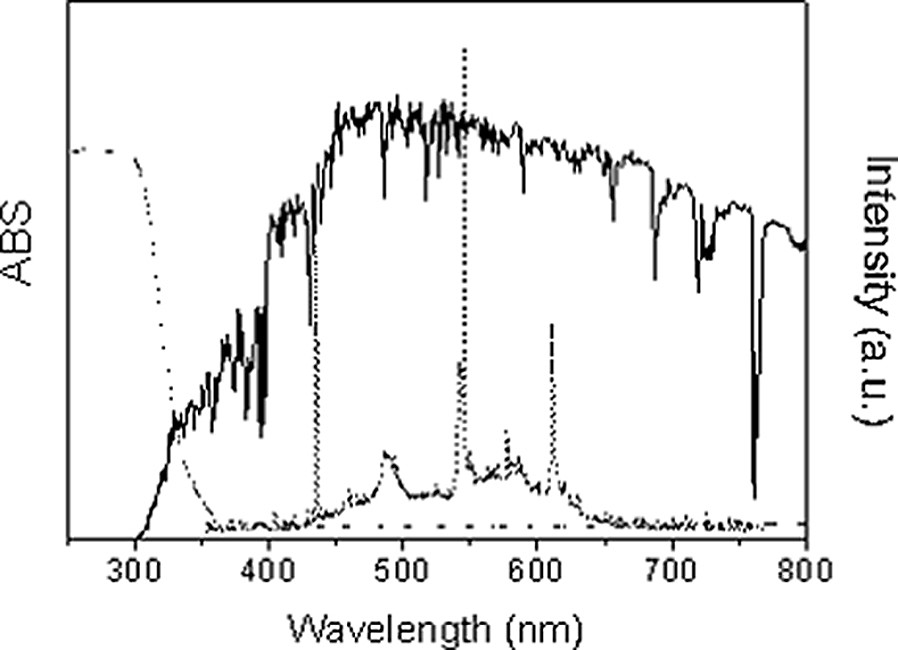
Spectra of AM 1.5G sunlight (solid line) and a fluorescent lamp (dashed line), and absorption spectrum of anatase film photocatalyst (dotted line).
To utilize the visible light for photocatalysis, several different approaches have been proposed:
- • Utilization of semiconductors, such as CdS, Cu2O, Fe2O3, WO3, etc., which can absorb visible light [6]. However, this attempt failed, since no semiconductors thus far could meet the requirements of band gap, chemical stability, and photocatalytic activity together. The recently reported InTaO4 photocatalyst can photo-split water with visible light, but its efficiency was lower than 1% [4].
- • Doping of photocatalysts with metal ions (for example Cr3+, Fe3+), which create local energy levels within the band gap of the photocatalyst, with corresponding absorption bands lying in the visible spectral range [27,28]. It was assumed that the photoexcitation of such impurities should lead to the generation of free charge carriers to initiate surface chemical processes. However, the efficiency of such systems under visible light strongly depended on the preparation method used. In some cases, such doped photocatalysts showed no activity under visible light and lower activity in the UV spectral range compared to the non-doped photocatalysts because of high carrier recombination rates through the metal ion levels.
- • Doping of photocatalysts with non-metal atoms, such as N, S and C. Asahi et al. reported significant red shifts (up to 540 nm) of the spectral limit of the photoactivity of TiO2 photocatalysts doped with N [29]. They interpreted such results in terms of band gap narrowing due to mixing of the p states of the dopants with O 2 p states forming the valence band of TiO2, as illustrated in Fig. 12. A similar conclusion has also been reached by Ohno et al. [30] for the spectral changes observed for S-doped TiO2.

Schematic diagram of the electronic structures of TiO2 (left), TiO2 with light N-doping (middle) and TiO2 with heavy N-doping (right).
Among the three approaches, the third one tends to be the best for the development of photocatalysts utilizing visible light. Actually, commercial visible light-type TiO2 (VL-TiO2) photocatalysts based on the third method, i.e. the anion doping method, have been commercially available in the form of powders and colloidal solutions in Japan. VL-TiO2-based filters for air cleaners are also available commercially. It is apparent that all of the applications of TiO2 photocatalysis discussed above can be promoted with VL-TiO2, especially for indoor antibacterial and self-cleaning applications, and the applications in water cleanup with solar light. The present problem for the anion-doped TiO2 photocatalysts is that their photocatalytic activities under visible light are much lower than those under ultraviolet light. Much effort must be devoted to overcome this obstacle.
4.2 Light source
So far, the most common light source in use in TiO2 photocatalysis is the black-light-type UV lamp, which emits light in the UV-A bands (λmax = 355 nm). A black-light tube is basically a fluorescent lamp with a different phosphor coating. This coating absorbs harmful short-wave UV-B and UV-C light emitted from energized mercury atoms, and emits UV-A light. The black glass tube itself blocks most visible light, so in the end, only benign long-wave UV-A light and some blue and violet light pass through. The tube-type black light is a relative cheap, low power UV source, and most importantly, TiO2 can utilize its emission highly efficiently. Therefore, it has been widely applied in commercial air cleaners and water cleaners.
However, the volume of the tube-type black light is relative large, so that it cannot meet the requirements of miniaturized photocatalytic devices. Recently, light-emission diodes that can emit ultraviolet light have appeared on the market. Fig. 13 shows the spectral distribution of two ultraviolet light LED (UVLED) devices produced by the Izumi Optical Devices Co., Japan. Each device (5 × 5 × 0.3 cm), consisting of 25 isolated LEDs, operates at DC 24 V. The peak emissive wavelengths are 370 and 400 nm for the two devices, respectively. The device with the emission of 370 nm is well suited for anatase-type TiO2 photocatalysts. The device with the peak emission of 400 nm, however, may be utilized by rutile-type or VL-type TiO2 photocatalysts. UVLED devices are almost ideal light sources for miniaturized photocatalytic devices. However, their price is much higher than that of the tube-type black light, due to the usage of a GaN-based luminescent layer. Further reductions in production costs are necessary for the practical application of UVLEDs in photocatalysis.
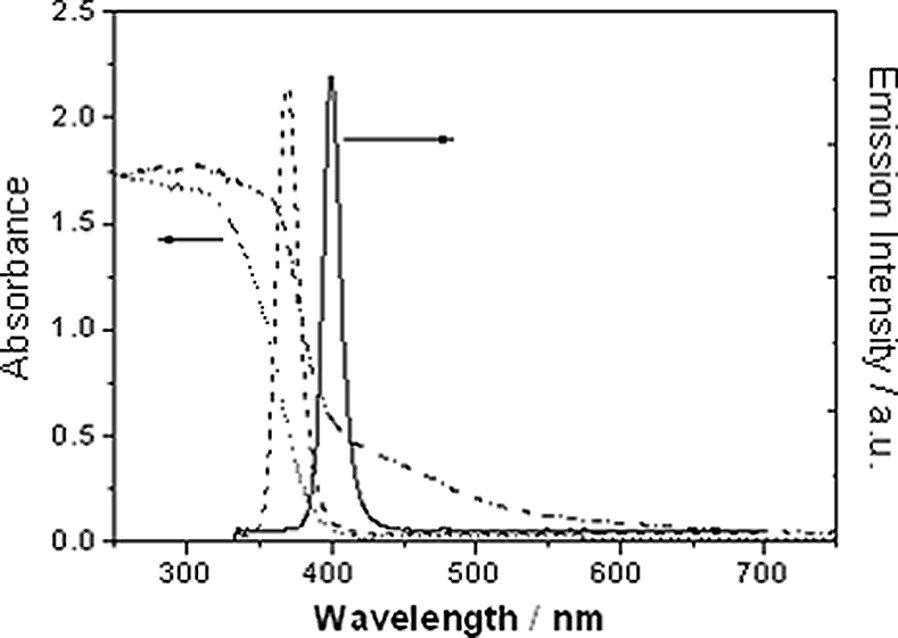
Emission spectra of the UVLED with central emission at 400 nm (straight line) and the UVLED with central emission at 370 nm; and absorption spectra of anatase TiO2 photocatalyst (dotted line) and VL-TiO2 photocatalyst (dash-dotted line).
4.3 Standardization
To establish a set of standard evaluation methods for photocatalytic products is another urgent subject for TiO2-based photocatalysis. The market for photocatalytic products has grown to tens of billion Japanese yen in Japan in a short period from the mid-1990s and is expected to reach one trillion yen in the near future. However, despite the vast enthusiasm of the photocatalysis industry, consumers often cannot understand well the performance of photocatalytic products, or cannot make reasonable comparisons among the products from several producers, because of a lack of standard evaluation methods for photocatalytic products.
We started the Photocatalysis Standardization Committee in Japan in September of 2002. This committee is made up of four subcommittees, in charge of standard evaluation methods for self-cleaning products, air purification products, water purification products, and antibacterial products. The committee, composed of ca. 60 members from experts, manufacturers and users, is sponsored by the ministry of economy, Trade and Industry (MITI) of Japan. The first proposal, photocatalytic materials–air purification test procedures, has been proposed to the Japan Industry Standardization (JIS) committee, and has also been proposed to ISO/TC206. This committee has also made great progress in the evaluation methods for self-cleaning products, antibacterial products, and water purification products, etc.
The standardization of photocatalytic products has also started in Europe. In Asia, China and South Korea have also started standardization procedures. We expect that international standard evaluation systems for photocatalytic products, which are vital for the healthy development of the photocatalysis industry, will come into being in the world in the near future.
4.4 Popularization
This past April, Professor Ken-ichi Honda and the author (A.F.) were awarded the Japan Prize for Chemical Technology for the Environment, for our pioneering work on photochemical catalysis and its applications for the environment. It is a great academic honor for us, and it means that the importance of TiO2 photocatalysis for environmental cleanup has received wide acceptance from the academic community. However, the future of TiO2 photocatalysis is dependent more on the acceptance from the public.
On July 21 of this year, we opened the Photocatalysis Museum in the Kanagawa Academy of Science and Technology (KAST). It is the first museum for photocatalysis in the world. More than 40 main manufacturers exhibit their photocatalytic products in the Museum, which represents the current commercialized photocatalytic products in Japan. Also, there is a corner for all kinds of books on semiconductor photocatalysis inside the Museum, which are available for visitors. Moreover, we have offered the Museum an additional function for testing the performance of photocatalytic products. Fig. 14 shows the result of an outdoor test of a self-cleaning tent produced by the Taiyo Kogyo Co. of Japan. The left half of the tent was coated with titanium dioxide on the surface of PVC plastic film, while the right half of the tent exposed the plastic film directly to the ambient environment. This test was started on the opening day of the museum. After 2 months, the right part of the tent had already turned gray due to soilage; however, the TiO2-coated left part has stayed white, which clearly exhibits the self-cleaning function of the TiO2 coating.

Photograph of a tent (Taiyo Kangyo Co.) whose left half has been coated with a TiO2 self-cleaning layer after an outdoor exposure test for 2 months in the Photocatalyst Museum of KAST.
There have been more than 3800 visitors visiting the Museum during the first 2 months, including students, housewives, engineers, and government officials. We expect that the Museum can help the visitors to literally get in touch with TiO2 photocatalysis, and thus it can popularize the concepts of photocatalytic environmental cleanup to the public. We also expect to see more and more public facilities similar to the Photocatalysis Museum appearing in the world.
Acknowledgements
The authors thank Dr. D.A. Tryk for carefully reading the manuscript.
Vous devez vous connecter pour continuer.
S'authentifier