1 Introduction
Recently, many researches on the photocatalytic process of water treatment using titanium dioxide to eliminate different contaminants from water have been highlighted. [1–8]. At present, the researchers are searching for the possibility of using TiO2 under visible-light irradiation (the absorption spectrum of TiO2 is then shifted toward the solar spectrum range, over 380 nm). One approach was doping a transition metal into TiO2, another was formation of the reduced TiOx phase of TiO2 photocatalyst [9].
Herrmann et al. [10], investigated the photocatalytic degradation of the pollutants under a visible light on the different kinds of titanium dioxides. They used various TiO2 samples of industrial origins (P25, Hombikat UV 100, TiLCOM HC 120, TiONa PC 10). From their research follows the fact that Degussa P25, despite its moderate specific surface area (50 m2 g−1), was a very efficient photocatalyst [10]. They investigated also a new series of titania, which was comprised of industrially photocatalysts that had been elaborated by Millenium Inorganic Chemicals and were denoted Millenium-PC/10, PC/25 and PC/50, with respective surface areas equal to 11, 23 and 43 m2 g−1, and concluded that Millennium-PC/50 was a better catalyst than Degussa P25. A practical advantage of Millennium-PC photocatalysts is their fast rate of decantation, which is very helpful for final separation of photocatalyst from purified water [13]. They have been also interested in the synergistic effect between titania and activated carbon in an aqueous suspension. The synergy effect took place in a large solar pilot plant scale, working in the near UV-fringe of sun-light [11]. It has been demonstrated that the tedious filtration of titania in slurry can be avoided by using a new photocatalytic material, Millenium PC500 titania coated on Ahlstrom non-woven paper [14].
The different kinds of pollutants were decomposed under a visible light: 4-chlorophenol [10,11,13,14], pesticides [14–16], dyes [14,17], and nitrobenzene. The presence of anions – chloride, carbonate, bicarbonate, sulphate, and nitrate –, initial pH and small amount of FeSO4 (0.01 M) had negligible effect on the photocatalytic degradation of nitrobenzene [12,13], 2-chlorobenzoic acid, and hydrobutanedioic acid [13].
The photocatalytic process under a visible light was applied to different kinds of installations, such as a flow reactor of the pilot plant at Platforma Solar de Almeria (PSA) in Spain [10,11,13], the parabolic concentrator (CPC) [16] and reactor with a multi-step cascade falling-film [14].
In this paper, a photocatalytical activity under visible light on new TiO2 catalysts modified by carbon via carbonisation of n-hexane was presented.
2 Experimental
2.1 Materials
Tytanpol A11 (Police, Poland) was as TiO2 particles for carbon deposition. According to the manufacturer’s data, Tytanpol A11 has a specific surface area of 11.4 m2 g–1 and consists mainly of anatase crystalline phase (min. 98.5%) with particle size of 0.2–0.4 μm.
2.2 Preparation of modified photocatalyst samples
TiO2/carbon catalysts were obtained by keeping of TiO2 in an atmosphere of n-hexane for 3, 4.5 and 6 h at temperatures of 400, 450 and 500 °C. In Fig. 1, the scheme of the installation is presented, which is equipped with the pipe furnace type R40/250/12-C40 (Nabertherm, Germany), where the modification of the catalysts was performed. About 1 g of titanium dioxide in a porcelain boat was placed into the tube of the furnace. The vapours of n-hexane were introduced inside to the furnace by bubbling of pure argon gas through liquid n-hexane at room temperature. The samples were heated up to a programmed temperature (400, 450 or 500 °C) for 40 min and kept for 3, 4.5 and 6 h. After that time, the flow of n-hexane vapour was stopped, and then the pipe furnace was slowly cooled down to the room temperature (about 1.5 h). With increasing the heating time and the temperature, the samples changed from light to intensive grey colour. This colour was caused exclusively by carbon. The change of colour as result of reduction of Ti ions in TiO2 from Ti(IV) to Ti(III) was eliminated. According to Szczepankiewicz [18], a band of ion Ti(III) around 3716 cm−1 should be found, whereas on the our FTIR spectra of photocatalysts modified by carbon, the presence of ion Ti(III) was not observed.
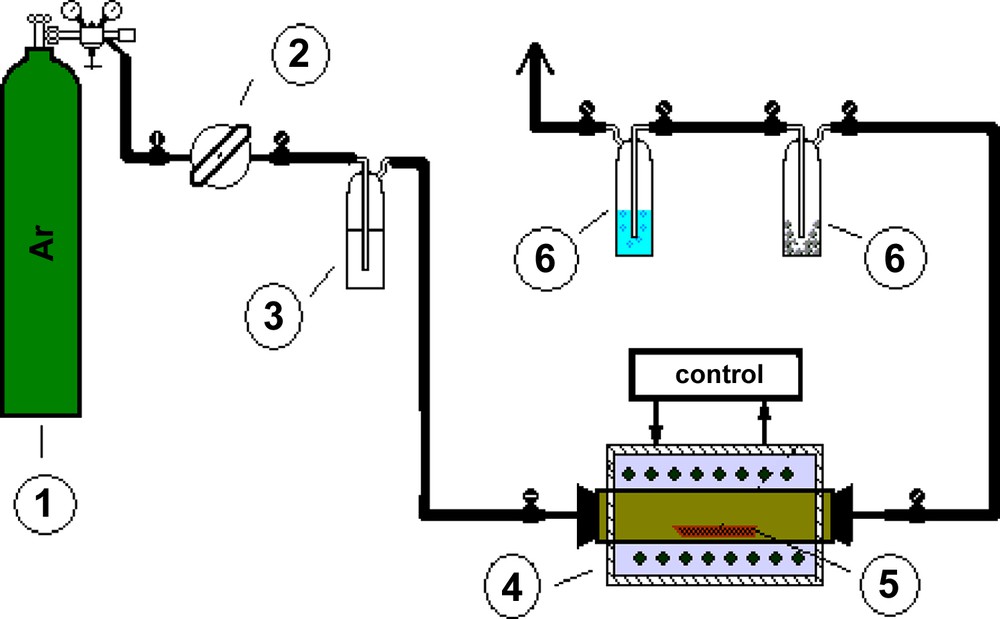
The installation set-up for deposition of carbon on TiO2 by carbonisation of n-hexane. 1–Gas cylinder with argon, 2–controller, 3–washer with n-hexane, 4–pipe furnace, 5–sample, 6–scrubber.
2.3 Experimental procedures and techniques
The photocatalytic decomposition of phenol was carried out in the glass beakers. The sample of 0.1 g was added to 500 ml of aqueous phenol solution with a concentration of 0.1 g l–1. The solution was mixed 24 h with a magnetic stirrer during the irradiation of visible light (light Philips 100 W) with an intensity of about 354 W m–2 (> 400 nm) and UV irradiation 0.16 W m–2 (< 400 nm). The visible-light intensity was measured by the radiation intensity meter LB-901 with external sensors PD204AB (Macam Photometrics Ltd) and CM3 (Kipp and Zonen). In order to determine the concentration of phenol, a few millilitres of the solution were taken from the reaction mixture and loaded in the UV–Vis spectrometer (Jasco, Japan) after filtration by Millipore disk (0.45 μm). The concentration of phenol in the solution was calculated by a computer program, using a calibration curve.
The concentration of carbon was determined by in situ burning of the samples at 1000 °C in a thermogravimetric system (STA 449 C TGA, Netzsch, Germany) under a flow of air of 25 ml min–1. For carbon calculation, the amount of chemisorbed and physisorbed water was taken into consideration.
The photocatalysts were characterised by UV–Vis/DR using Specord M40 spectrometer (Carl Zeiss, Jena, Germany) equipped with an integrating sphere accessory for diffuse reflectance spectra (BaSO4 was used as a reference). Both, spectra and band-gap energy calculation (EG) were performed by the Jasco procedure described elsewhere [19].
The diffuse reflectance FTIR spectra by using FTIR spectrometer (Jasco, Japan) equipped with DRS accessory of Harrick (USA) were recorded.
The turbidity of TiO2 slurry after 24 h of irradiation and 10 min of sedimentation was measured by using a turbidimeter (PCompact, Aqualytic).
3 Results and discussion
The influence of the content of carbon on phenol decomposition after 24 h of visible-light irradiation is presented in Fig. 2a. The catalysts with small carbon content (0–0.15 mass%) and with high carbon content (0.69–0.85 mass%) have almost the same rate of phenol decomposition as the pristine A11. In this case, the adsorption of phenol for the sample with the maximum carbon content (0.85 mass%) was negligible (less than 0.3%).
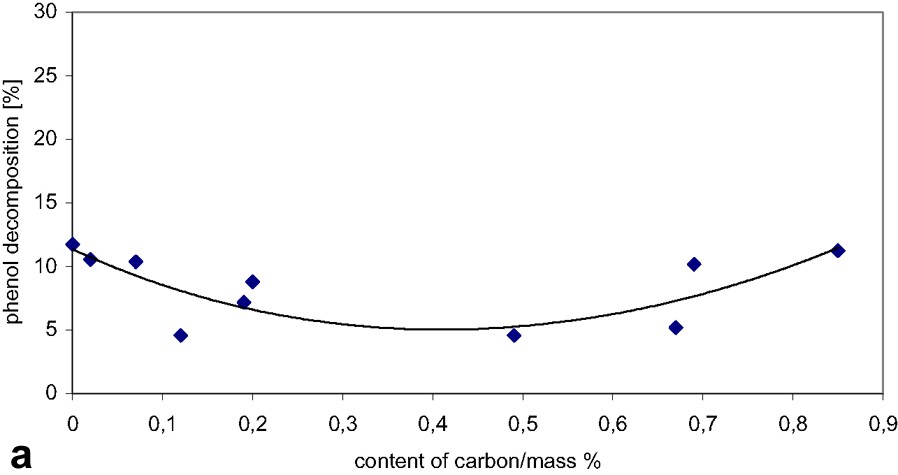


The influence of the content of carbon in TiO2/carbon catalysts on: (a) phenol decomposition after 24 h of the visible-light irradiation; (b) turbidity of titanium slurry after 24 h of the visible-light irradiation and 10 min of sedimentation; (c) the ‘practical efficiency’.
In Fig. 2b, the influence of the content of carbon on the turbidity of phenol solutions is presented. The turbidity parameters of these samples can be compared because the particles size after carbon deposition remains the same as before. With increasing the content of carbon in the catalyst, the turbidity of the solution also increased. But only in the case of two catalysts with the carbon contents 0.69 and 0.85 mass%, the turbidity was definitely lower. The influence of the carbon on the surface of titanium dioxide was described in our previous paper [20].
The photoactivity of the catalysts and their ability to decantation (turbidity) are two important factors that have an influence on the ‘practical efficiency’ of photocatalysts. Araña et al. [21] defined the ‘practical efficiency’ of activated carbon–TiO2 catalysts by taking into account the rate constant for water mineralisation of TiO2 and activated carbon–TiO2 and also their ability to sedimentation. In this experiment, the formula to calculate the ‘practical efficiency’ of the catalysts was insignificantly modified, sedimentation was replaced with turbidity. After modification, the formula is as following:
A–% of phenol decomposition by the catalyst after 24 h of visible light irradiation;
B–normalised turbidity of the sample after 24 h of irradiation and 10 min of sedimentation.
In Fig. 2c, the ‘practical efficiency’, which is a relative value to the pristine catalyst A11, is presented for the present catalysts as a function of carbon content. For the catalysts that have a content of carbon under 0.69 mass%, the value of the ‘practical efficiency’ is around 1, but for the catalysts having the content of carbon from 0.69 to 0.85 mass%, the ‘practical efficiency’ is very high. The ‘practical efficiency’ of the catalyst containing 0.85 mass% of carbon is 14 times higher than that of pristine A11, because it has the smallest turbidity and the same photocatalytic activity as pure A11. It is worthwhile to mention that under UV radiation the present photocatalysts showed the highest value of the ‘practical efficiency’ at 0.2% carbon content [20].
For the catalysts containing small amounts of carbon, such as 0.2%, the character of the catalyst surface changed from hydrophobic to hydrophilic after the long time of photocatalytic reaction under visible light, as shown by the FTIR/DR spectra in Fig. 3a. From the change in spectrum before and after the photocatalytic reaction, the amount of hydroxyl groups was supposed to increase significantly after 24 h of reaction, which revealed that the modification of the surface of TiO2 was not stable enough (the characteristic hydroxyl group bands were found at 2800–3700 and 1550–1800 cm−1).
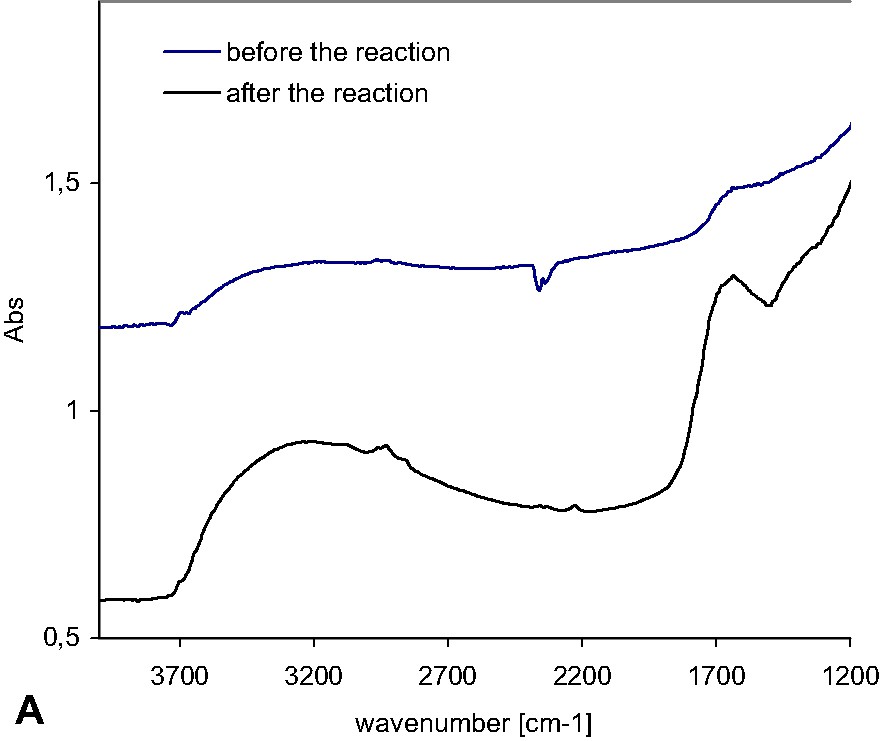
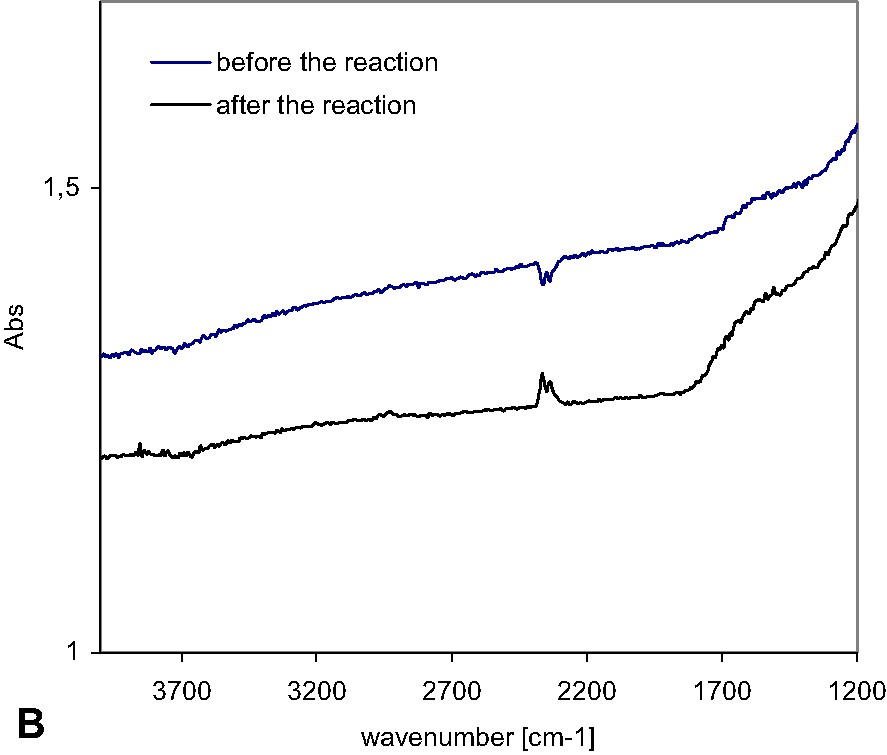
FTIR spectra of TiO2 and TiO2/carbon catalysts heated at: a) 450 °C for 4.5 h, before and after the photocatalytic reaction under visible light. b) 500 °C for 6 h, before and after the photocatalytic reaction under visible light.
For two catalysts with high amounts of carbon, 0.69 and 0.85%, however, the character of their surfaces did not change, as shown by FTIR/DR in Fig. 3b; there is no change in the spectrums. This indicated that the modification of the surface was stable and that the reaction did not change the character of the photocatalysts surface.
For all the catalysts prepared, no difference in band-gap energy EG measured from UV–Vis/DR spectra was detected; in other words, there is no change in EG induced by the carbon modification, independently of the carbon content. The observed values of EG were listed in Table 1. However, we have to point out that when we modified the surface of TiO2 by carbon, the intensity of transmittance of visible light decreases and the catalysts adsorb the visible light, as shown in Fig. 4, e.g. for samples modified at a temperature of 400 °C. The behaviour of the intensity for the rest of the samples was the same.
Calculated energy gap (EG) for studied samples of photocatalysts
Contents of carbon in TiO2/carbon/mass% | Eg [eV] |
0 | 3.31 |
0.07 | 3.36 |
0.12 | 3.36 |
0.19 | 3.38 |
0.20 | 3.38 |
0.49 | 3.39 |
0.67 | 3.34 |
0.69 | 3.42 |
0.85 | 3.42 |
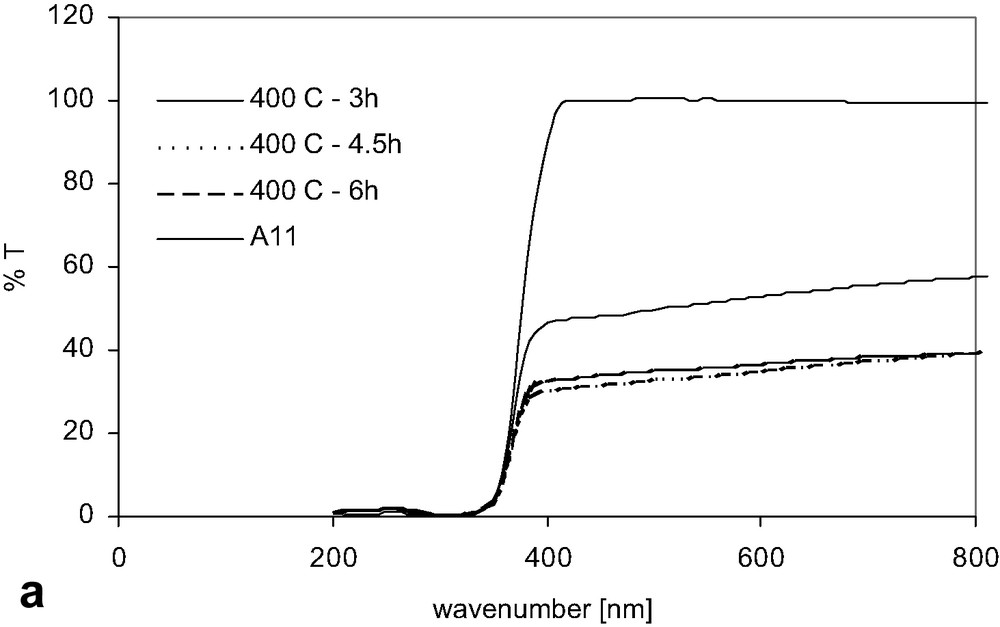

The UV–Vis/DR absorption spectra (a) of a pure TiO2 and the catalysts modified at a temperature of 400 °C and the first derivatives (b) of UV–Vis absorption.
The modification of TiO2 by the 0.69 and more % of carbon deposition via n-hexane improved turbidity of solution after catalyst sedimentation, increasing in this way possibilities of practical application of TiO2 as a photocatalyst in water-treatment systems under visible light.
4 Conclusions
The titanium dioxide of anatase form was modified by carbon deposition from carbonisation of n-hexane vapours at 400, 450 and 500 °C. The resultant catalysts were tested in the decomposition of phenol under visible light. The catalysts modified with 0.85 mass% carbon had only a little lower catalytic photoactivity under visible light than pure TiO2; but showed almost 14-times higher turbidity in the solution than the pristine A11. The presence of carbon in TiO2 reduces the hydroxyl groups on the surface of titanium dioxide via –CH3 (alkenes) groups and in this way changes the character of TiO2 from hydrophilic to hydrophobic. Therefore, the photocatalyst showed a ‘practical efficiency’ 14 times higher than that of A11. Also the samples of highest carbon content (0.85%) form very stable composition with anatase under visible light.
The modification of TiO2 by carbon deposition via n-hexane markedly improved turbidity of solution after catalyst sedimentation, which increases the possibilities of practical application of TiO2 as a photocatalyst in water-treatment systems under visible light.