1 Introduction
The dye-sensitized nanocrystalline solar cells (DSSCs) have been researched extensively since first reported in 1991 [1]. This solar cell is comprised of a working electrode and a counter electrode separated by an electrolyte. The term dye-sensitized refers to the dye monolayer that is chemisorbed onto the nanostructured semiconductor surface, to generate the working electrode. The dye absorbs light to produce an electron that is injected into the semiconductor film. This electron flows through to the transparent conducting oxide (TCO) film on the substrate and around a circuit, where it can carry out electrical work, to the counter electrode and back into the electrolyte to reduce the I3– to I–, catalyzed by the platinum layer. The I– then reduces the dye back to its ground state, and is re-oxidized to I3–. Thus, the initial state of the solar cell is regenerated and further energy producing reactions can then occur.
Room temperature ionic liquids have been an area of research interest due to their negligible vapor phase, high ionic conductivity, wide electrochemical window and excellent thermal and chemical stabilities, as well as their potential for application in a variety of electrochemical device fields [2–5]. In particular, the ionic liquid (IL) systems based on ethylmethylimidazolium (emIm) cation seem to be promising for the high-performance DSSCs incorporating non-volatile electrolytes [6–9]. One of the major barriers to the application in such devices is still the relatively high viscosity of ionic liquids compared to those of organic solvents. This high viscosity leads to a slow rate of diffusion of redox species. These combination have triggered our interest in the DSSC properties of further ethylmethylimidazolium-based ILs with anions which are close relatives of dicyanamide [10,11], as the viscosity of the ILs can be varied by replacing different anions. In this study, the effect of three different anions, tricyanomethanide (TCM), dicyanamide (DCA) and thiocyanate (SCN) with the same emIm cation, on viscosity, ionic conductivities and their performance in DSSCs have been investigated.
2 Experimental
The three ethylmethylimidazolium-based ILs, ethylmethylimidazolium tricyanomethanide (emImTCM), ethylmethylimidazolium dicyanamide (emImDCA) and ethylmethylimidazolium thiocyanate (emImSCN), were synthesized as described in our previous publications [10–12]. Their chemical structures are shown in Scheme 1. Ethylmethylimidazolium iodide (emImI) and PMII were synthesized according to the reported procedure [13]. All manipulations for the preparation were carried out under an inert atmosphere in a glove box. Other chemicals were used as received.
Conductance measurements were performed using a locally designed dip cell probe consisting of two platinum wires sheathed in glass. The sample was loaded into the cell and an O-ring fitted between the sample vial and the probe wall. The cell constant was determined with a solution of 0.01 M KCl at 25 °C. Conductivities were obtained by measurement of the complex impedance spectra between 1 MHz and 10 KHz on a HP 4284A dielectric interface. The temperature was varied in 5 °C (± 1 °C) intervals using an Eurotherm 2204e interfaced to the HP and a cartridge heater set in a brass block with a cavity for the cell. A T-type thermocouple was set in the block adjacent to the cell. The conductance was determined from the first real axis touchdown point in the Cole–Cole plot of the impedance data.
The viscosities of the ionic liquids were measured with an ASTM Kinematic Viscometer 400 (Standard Test ASTM D-445, IP-71, ISO-3104) at 25 °C. Kinematic viscosity in centistokes was obtained by multiplying the efflux time in seconds by the viscometer constant. To obtain the viscosity in centipoise (Cp) the kinematic viscosity in centistokes is multiplied by the density in grams per milliliter. Measurements of the electrochemical windows of the ionic liquids were carried out in a nitrogen atmosphere (dry-box) using a MacLab potentiostat, and MacLab software. Electrodes consisted of a glassy carbon working electrode, a platinum wire counter electrode, and a silver wire pseudo reference electrode.
DSSCs were constructed from TiO2-coated TCO plates (TiO2 working electrodes were produced from a slurry consisting of 4 ml distilled water, 2 g of a preformed TiO2 nanopowders (P25, Degussa), 100 μl Triton X-100 (Lancaster) and 25 μl acetylacetone (BDH). The slurry was coated using a doctor blade technique on F:SnO2 glass (TEC8, Hartford Glass Co.), then sintered at 450 °C for 0.5 h From profilometry (AltiSurf 500, Cotec), it was determined that the film thickness was 10 ± 1 μm. Pt-catalyzed counter electrodes were produced by coating a thin film of a 5 mM H2PtCl6 solution in isopropanol on F:SnO2 glass. The electrodes were dried at 50 °C for 15 min and then heated at 2 °C min–1 to 385 °C for 15 min before annealing to room temperature. Prior to dye adsorption at 80 °C, the semiconductor electrodes were heated at 450 °C for 15 min to remove any adsorbed water. The hot electrodes were immersed in a 0.3 mM N3 dye ([Ru(dcbpy)2(SCN)], where dcbpy = 4,4′-dicarboxylic acid-2,2′ bipyridine, solution in a 1:1 solution of butanol and acetonitrile for 20 h). A 200 μm thick Surlyn™ gasket was then cut to fit around the TiO2 film on the electrodes and the cells sealed by heating. The IL electrolytes were sandwiched between the electrodes by back filling the electrolytes under vacuum, separately.
The current–voltage curves were determined under illumination with a 21 V, 80 W halogen lamp (Phillips). Labview 6.1 (National Instruments) drove a Kiethley 2400 source meter to record the current across a linear voltage sweep from –0.2 to 0.8 V. Light intensity was determined by an externally calibrated photodiode. Cells were mounted on a heat sink and measurements were limited to less than 1 min in order to minimize temperature rises.
3 Results and discussion
Fig. 1 shows that the structures of the TCM and the SCN ILs are close relatives of the DCA IL, which is a known low melting point and low viscosity IL. The TCM anion and its conjugate acid, cyanoform, HC(CN)3, has been known for many years. Compared to the DCA IL and the SCN IL, a reduced ion-pairing effect with the cation maybe expected for the planar structure and the stronger charge delocalization on the TCM anion.

Ethylmethylimidazolium-based ILs.
The conductivity of the three ILs, compared in Fig. 2, was found to be very high between room temperature and 70 °C for all three ILs. The conductivity provides information on the mobility of the ions and ion-pairing phenomenon, which are expected to influence the photovoltaic performance and in particular the fill factor of the solar cells. The conductivity of the TCM IL at 25 °C is 20 mS cm−1 and the SCN IL is 18 mS cm−1 at 25 °C. These conductivities are superior to that of emImTFSA (8.8 mS cm−1), the anion that has been most popular for the application in lithium batteries, but quite similar to that for the DCA IL (22 mS cm−1) at 25 °C.
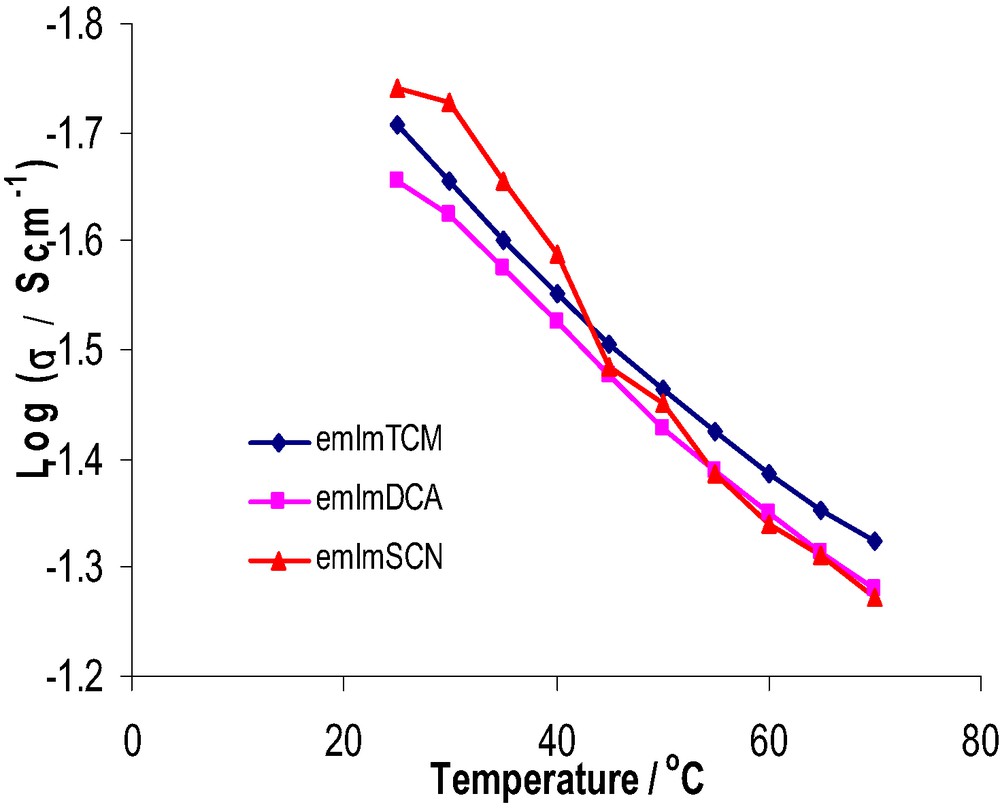
Conductivities of the three ethylmethylimidolium-based ILs.
Table 1 shows that the TCM IL has the lowest viscosity and narrower window of electrochemical stability among the three ILs. Importantly this window is sufficiently wide to allow the use of this IL as a electrolyte for DSSCs. Among the three ILs, the strongest charge delocalization occurs over three cyanide groups on the TCM anion and, thus, the weakest ion-pairing with the emIm cation, providing the emImTCM IL with the lowest viscosity. Although the TCM IL has very similar conductivity and electrochemical stability to the DCA IL, it is less hygroscopic than the DCA IL. This property also provides a much better framework for DSSC construction and longevity.
Viscosities and electrochemical windows of the ILs at 25 °C
emImTCM | emImDCA | emImSCN | |
Viscosity (Cp) | 19.56 | 28.09 | 32.89 |
Electrochemical window (V, Ag/Ag+) | –1.6 to 1.2 | –1.3 to 1.6 | –1.5 to 0.8 |
Fig. 3 presents typical photocurrent density–voltage curves obtained under 30% Sun light intensity using different IL electrolyte systems. As described in Section 2, the photoanodes have not been optimized. Moreover, the used 200-μm-thick SurlynTM to seal the cells meant that the cells were much thicker than the cells sealed with 25-μm-thick SurlynTM (unavailable to us at the time) [14]. Therefore, the cell efficiencies are lower than would be expected for fully optimized cells [6–9]. However, this is the first report for emImTCM electrolyte system in the literature that has been applied to DSSCs. These results are very promising in comparison to other previously published systems such as DCA and SCN [7–9]. A typical electrolyte contained 0.1 M iodine and 0.45 M N-methylbenzimidazole (NMBI) in a mixture of PMII and emImTCM (volume ratio of PMII to emImTCM is 7:13). To facilitate comparison with the other electrolytes used in Fig. 3, the concentration and molar ratio of [I–] to [I2] were always kept constant (lithium iodide was the source of lithium ions).

I–V curves of DSSCs comprising of the ILs at 30% Sun light intensity, where (a) is emImTCM doped by PMII/I2, (b) is emImTCM doped by emImI/I2, (c) is emImTCM doped by emImI and LiI/I2, (d) is emImDCA doped by PMII/I2, (e) is emImDCA doped by emImI/I2, (f) is emImDCA doped by emImI and LiI/I2 and (g) is emImSCN doped by PMII/I2.
The photovoltaic data for DSSCs operated with the TCM IL performed best under 30% Sun light intensity when the initial optimized electrolyte composition is used. Under the same light intensity, the SCN IL performed best under the initial electrolyte conditions without addition lithium ions. A conversion efficiency of 2.1% at 30% light intensity was observed for the TCM IL doped with lithium ions, iodine and emImI. Efficiencies of 0.7% and 1.7% at the same light intensity were observed in terms of the DCA and the SCN ILs, respectively. The TCM IL performed well for both short circuit current density and open-circuit photovoltage with the use of emImI instead of PMII and/or the introduction of lithium ions. The TCM IL performed the best as it has the weakest ion-pairing with the anion as well as the lowest viscosity. This combination provides good permeation through the nanstructured working electrode and good mass transport. The DCA IL also performed well when emImI was used instead of PMII but was not better than the TCM IL. However, the addition of lithium ions was found to slightly improve the photovoltaic performance of the DCA IL.
4 Conclusion
Room temperature ionic liquids incorporating TCM and SCN as anions have, for the first time, been used as electrolytes systems in DSSCs. It was found that the TCM IL could be more useful than the DCA IL for application in DSSCs duo to a combination of a lower viscosity, when compared with DCA IL, lower hygroscopic native and sufficiently high conductivity. The SCN is very promising when a light intensity is less than 30% Sun light intensity. Comparing the TCM and the DCA ILs systems, the short circuit current and open-circuit voltage were found to be higher for the lower viscosity TCM IL system under 30% Sun light intensity. Optimization of the electrolyte systems and DSSC construction is being carried out in order to improve cell performance under conditions of higher light intensities, especially up to 1 Sun conditions.
Acknowledgements
We are grateful to the Australian Research Council for financial support under the Discovery Project Scheme (DP0210226). David Menzies was financially supported by a Monash Graduate Scholarship. The authors would like to thank Dr. Sophie Cerneaux, Monash Solar Cells Group, for the French translation.