Lord Porter of Luddenham, better known as Sir George Porter (Fig. 1), served as a member of the International Organising Committee (IOC) of the IPS series of conferences until his death in 2002. As one of his many former students and disciples, I was honoured to be asked to speak about his life and work at IPS-151.
1 Early life
George was born in 1920 in Stainforth, Yorkshire, the only child of parents who married relatively late in life (Fig. 2). He was educated at the village junior school, and then at Thorne Grammar School, Doncaster. He was encouraged in his early enthusiasm for chemistry by his father, who bought him an old bus to use as a laboratory: fifty years later, George was to remark that he was “still very fond of explosions”.
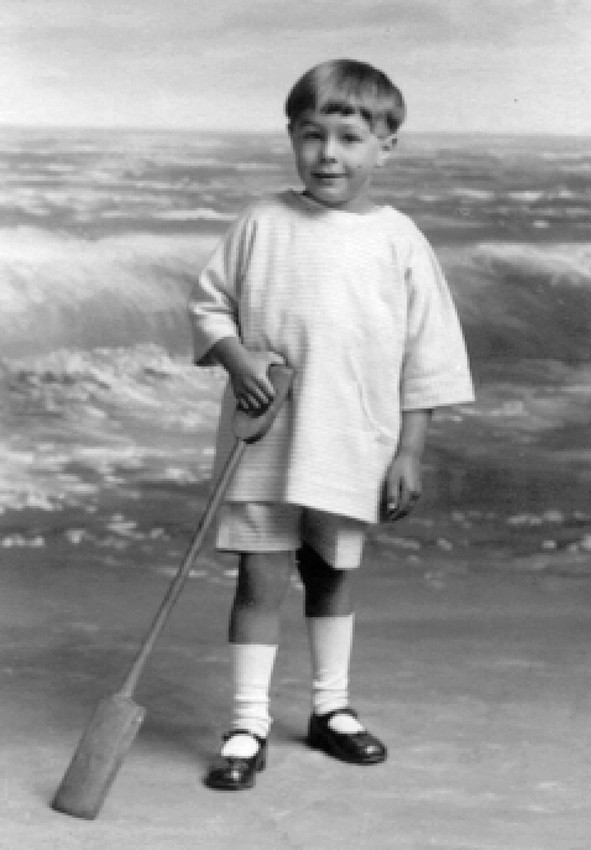
George as a small child.
He went up to Leeds University in 1938 to read chemistry (Fig. 3), but his studies were interupted when he was recruited into the Hankey Scheme to be trained as a radar operator. This led to his war service in the Mediterranean (Fig. 4), and also to his lifelong love of boats and sailing.
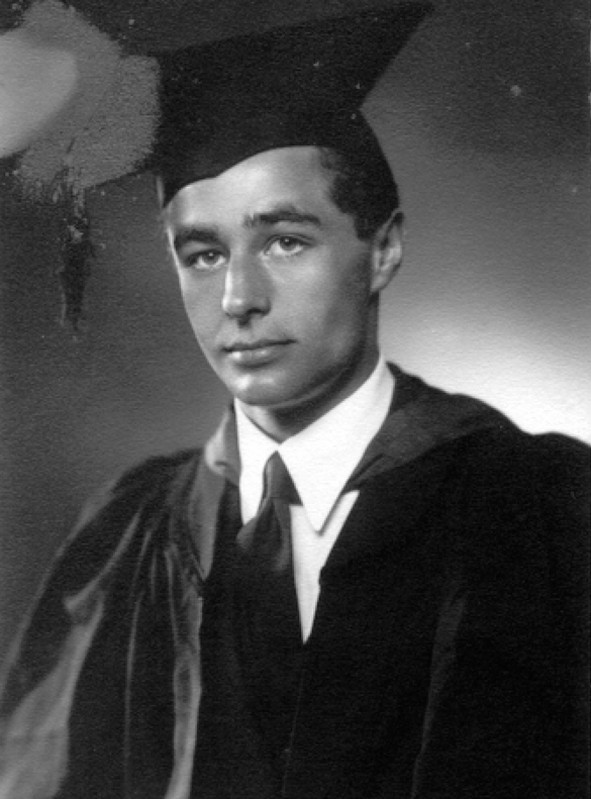
As a young graduate.

As a Royal Naval Volunteer Reserve Radar Officer in World War II.
2 Cambridge
In 1945, George went up to Cambridge to do a PhD with Professor Ronald Norrish. Norrish set George the problem of trying the detect the methyl radicals formed by photolysing mercury dimethyl with an arc light, but this did not work because the continuous light source was not bright enough to form the radicals in detectable concentrations.
George struggled with this technique for about a year until, combining his knowledge of radar pulses with his access to war surplus equipment, he had the brainwave of using the much more intense, short-lived flash of light that could be made by discharging a condenser bank through an inert gas as his light source. He persuaded the Navy to give him a large number of war-surplus condensers, which he set up in a basement room in the old Free School Lane laboratory to discharge a thunderous 2000 microcoulombs through a searchlight. The resulting millisecond-long flash of light produced abundant free radicals, which he detected spectroscopically by sending a second ‘probe’ light pulse through the flashed sample after a (mechanically controlled) time delay.
Thus was born the immensely powerful technique of flash photolysis with which George's name will always be associated. As he pointed out in his Nobel Symposium lecture of 1967, the particular power of this technique is its ability to produce large perturbations in very short times [1]. Indeed, the very first flash photolysis experiment George did, on acetone, resulted not only in the complete decomposition of the acetone, but also in the deposition of filaments of carbon throughout the reaction vessel [2]. He moved on to study the ClO and other inorganic radicals, and then a wide range of organic molecules, establishing the free radical mechanisms of their photochemical reactions in a series of landmark experiments.
Another of George's seminal achievements was the direct detection of the triplet state. Although triplets are quite long-lived at low temperatures, and their phosphorescence had been observed in rigid glasses by Lewis and Kasha in 1944, they are much shorter-lived at room temperature, and were consequently undetectable. In 1952, George and his student Maurice Windsor pared their flash duration down to 25 μs, and were immediately successful in detecting the 200 μs triplet state of anthracene dissolved in hexane, and in making the first record of a triplet-triplet absorption spectrum (Fig. 5) [3]. Using a long heated reaction vessel, George and Frank Wright then measured the absorption spectra of many other triplet states, opening the way to the elucidation of major swathes of organic photochemistry and the related fields of photosensitisation, exciton formation and triplet energy transfer, quenching and annihilation [4].

The first triplet–triplet absorption spectrum (Porter and Wilkinson, 1961).
3 Sheffield
George left Cambridge in 1954 to spend a year as Assistant Director of the British Rayon Research Association in Wythenshawe, Manchester, where he studied the phototendering of dyed cellulose fabrics in sunlight, a problem he and Keith Bridge traced to a triplet-initiated hydrogen abstraction reaction [5]. However, he found the commercial environment restrictive, so when an invitation came from the University of Sheffield in 1955 to become their first professor of physical chemistry, he accepted, and retained this position until 1963, when he succeeded Robert Haworth as Head of Department. As his colleague David Cook noted of George's time in Sheffield:
“He brought with him a breath – a blast, actually – of fresh air into what was still basically a ‘Herr Professor’ world. Whereas previously staff and students alike were called by surname only, George (as all the staff knew him) introduced the kind of informality which is now common everywhere… He also introduced a completely new stream of theoretically oriented research and associated teaching, and during his tenure, he presided over the expansion of this area of science with appointments of academic staff who were to fill the chemistry chairs in many of the new universities [6].”
At Sheffield, George and his group continued pioneering work on the photoprocesses of organic molecules, particularly of ketones (with Frank Wilkinson, Paul Suppan and Alan Beckett) and quinones (with Ted Land and David Kemp). Of note also were George's studies of radiationless transitions with Malcolm Wright [7], and of the acid-base behaviour of excited states and radicals with Geoff Jackson and Ted Land [8]. With Peter Bowers [9], George measured the quantum yields of chlorophyll triplet formation in various media, showing how the photophysics of chlorophyll is influenced by its state of aggregation. With Frank Wilkinson, George made the first observation of triplet-triplet energy transfer in fluid solution [10]. He worked on triplet state decay with Louis Stief and Mort Hoffman, and on the absorption of triplet benzene with Tessa Godfrey. His papers on the mechanism of atom recombination (with Ashley Smith and Tom Glover), diffusion in viscous media (Alex Osborne) and the absorption spectra of aromatic radicals (Barry Ward, Samithra Savadatti and Brian Brocklehurst) were also of highest standard.
4 The Royal Institution
Around this time, George was forming links with the Royal Institution (RI), the venerable scientific establishment in London that boasted Humphry Davy and Michael Faraday among its past directors. In November 1960, he had given his first Friday evening Discourse on the theme of ‘Very Fast Chemical Reactions’ (demonstrating his theme with a matchbox and a piece of nitrocellulose), and in 1963 he accepted the RI's invitation to become Professor of Chemistry there. He remained in Sheffield for the time being, as this was a part-time position, involving no more than a couple of Discourses (The Law of Disorder, 1964; The Chemical Bond since Frankland, 1965) and a series of Schools Lectures (Chaos and Chemical Equilibria, 1963 and 1965; Electrons in Molecules, 1964; Patterns of Chemical Change, 1965).
By the time Sir Lawrence Bragg retired in 1966, George was the obvious choice to succeed him as Director and Fullerian Professor of Chemistry of the Royal Institution. The following year, George won the Nobel Prize for chemistry, together with Ronald Norrish and Manfred Eigen of the Max Planck Institute, Göttingen, ‘for studies of extremely fast chemical reactions, effected by disturbing the equilibrium by means of very short impulses of energy’.
The Royal Institution is a complex organisation. Not only is it a members' club with a great “repertory theatre of science” (Lawrence Bragg's description) devoted to the popularisation of science, it also contains the oldest independent research laboratory in the world, the Davy Faraday Research Laboratory (DFRL), established in 1896. George quickly lost his heart to the place. “I am glad my head is going to join it”, he remarked of the bust of him presented to the RI when he resigned in 1985 on his nomination as President of the Royal Society.
George was a natural communicator, a master of the art of the lecture demonstration (Fig. 6), and a pioneer of scientific programmes on television. His RI Discourse on the second law of thermodynamics was subsequently televised on BBC1 as a series of ten lectures entitled ‘The Laws of Disorder’ in 1965–1966. The first Discourse to be televised was George's ‘Quick as a Flash’, broadcast in December 1967 in celebration of his Nobel prize. He gave two sets of the RI's famous Christmas Lectures: ‘Time Machines’ (1969/70) and ‘Natural History of a Sunbeam’ (1976/77). His programmes for the BBC TV programme Horizon included ‘The Chemical History of a Candle’ (1964), its title borrowed from Faraday's immortal Christmas Lectures ‘adapted for a Juvenile Auditory’ of 1860, a Horizon Special for the centenary of Faraday's death in 1967, and ‘Dr Priestley and Oxygen’ (1983) for the 250th anniversary of Priestley's birth. He also chaired the Controversy series of debates televised from the RI (1971–1975), and for nearly fifteen years (1966–1981) he was a judge for the Young Scientists of the Year competition.
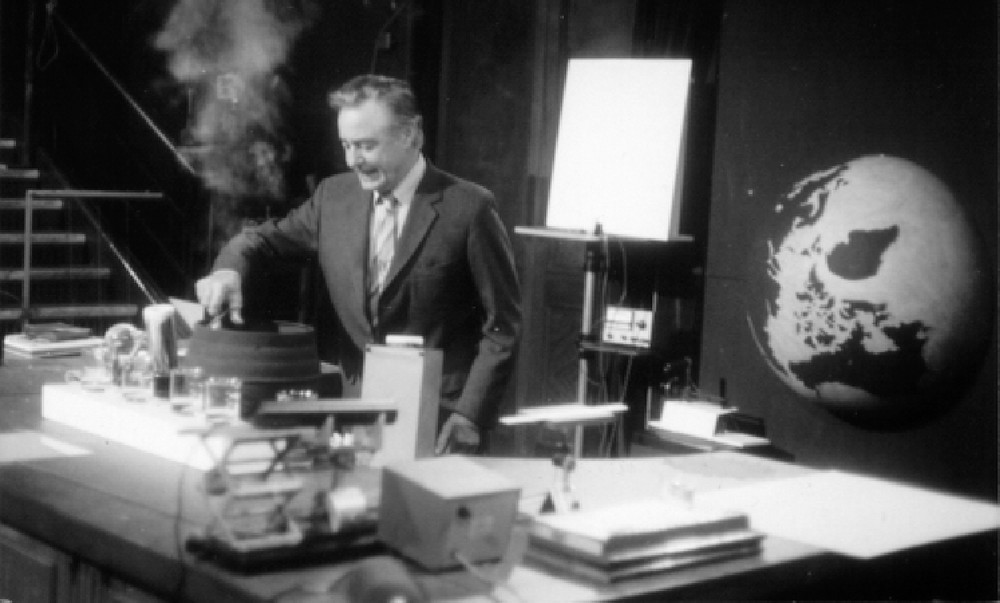
Giving a lecture demonstration at The Royal Institution.
George was an inspirational Director of the Royal Institution, but proud as he was to occupy the study once used by Michael Faraday, the RI's long history presented him with formidable challenges: these included dilapidated fabric, an archaic management structure and inadequate funding. George brought his formidable energy and persuasiveness to remedying these deficiencies, as well as building a strong research team in the DFRL. He raised funds to build the Bernard Sunley Lecture Theatre, endow the Wolfson Chair of Natural Philosophy, and create the Faraday Museum and the Archives Vault, opened by the Queen in 1973. A small company, Applied Photophysics, was formed, with George's former student Mike West as Managing Director, to manufacture the instrumentation developed in the DFRL.
Together with Val Tyrrell, a colleague from Sheffield days who became Secretary of the Royal Institution, George pushed through a reform of the RI's antique system of governance. He expanded the Schools Lectures, launched the extremely successful Mathematics Masterclasses in 1981, and the Royal Institution Centre for the History of Science and Technology in 1982. He presided over the Friday Evening Discourses – in those days still white-tie occasions – and together with his wife Stella entertained stylishly in the spacious Director's flat. He was knighted in 1972, following his two-year term of office as President of the Chemical Society, during which time he steered the amalgamation of the ‘old’ Chemical Society, the Royal Institute of Chemistry, the Faraday Society and the Society for Analytical Chemistry to form what subsequently became the Royal Society of Chemistry.
Meanwhile, George's research group in the DFRL was extending the range of flash photolysis to ever shorter times. Gas discharge flashes could not reach below microseconds, but the invention of the laser in 1960 allowed a huge extension in the accessible time domain. On his arrival in 1966, Mike Topp built the RI's first nanosecond pulse-probe flash photolysis system, based on a giant-pulsed Q-switched ruby laser. Along with a pulse length of only 20 ns, this had two other important novel features: a fluorescent dye emitter that replaced the troublesome spark system hitherto used to create the probe flash, and an optical delay train to control the timing of this flash. The optical delay proved invaluable, not only for nanosecond work, but also for the picosecond work that followed in the 1970s when it became possible to ‘mode-lock’ lasers so that a single Q-switched light pulse became a train of much shorter pulses.
The DFRL under George was a very international place, with post-doctoral students and distinguished scientific visitors from all over the world. For his students, the weekly meetings of the Photochemistry Discussion Group were a highlight, with George testing the speaker's grasp of his or her material between draws on his pipe under the ‘No Smoking’ sign in the Bernard Sunley Lecture Theatre. Work on short-lived reaction intermediates and the triplet state continued, and new areas of photophysics opened up as new, faster techniques were developed. Graham Fleming studied molecular rotational and relaxation dynamics, and cut his theoretical teeth arguing with ‘Prof’ over what the data meant. Godfrey Beddard built a time-correlated single-photon counting system to measure fluorescence lifetimes, and Euan Reid came down from Hull University to set up the RI's first picosecond system.
Another interest that George developed at the RI was in how green plants work. His 1978 study of energy transfer in the light-harvesting system of the red alga Porphyridium cruentum, carried out in collaboration with Jim Barber at Imperial College, is one example of this [11]. Another was his study with Angela Kelly and Menna Weese of model antenna systems built of chlorophylls dispersed in lipids [12]. These turned out to be very bad at energy transfer compared with the real thing. Analysing the failure, George concluded (correctly) that the random arrangement of the pigment molecules in his model systems allowed near-neighbouring pigment molecules to quench each other's excitation, a wasteful process that was avoided in vivo by a non-random arrangement of the pigment molecules. Fifteen years later, when the X-ray determination of the in vivo structures became possible, this was found to be so.
“Pure research, George once remarked, is merely research which has not yet been applied”, and it was lucky for me that he became interested in solar energy conversion around this time. In 1972, I moved from Oxford to London to work with George, switching my field from electrochemistry to photoelectrochemistry in the hope of making myself relevant to the thrust of his research (Fig. 7). Then came the oil price hike of 1973, hot on the heels of the seminal 1972 Nature paper by Fujishima and Honda on the photoelectrolysis of water at TiO2 electrodes. Suddenly there was funding for solar energy conversion and storage. George started a series of researches with Tony Harriman into photochemical hydrogen and oxygen production in sacrificial systems [13].
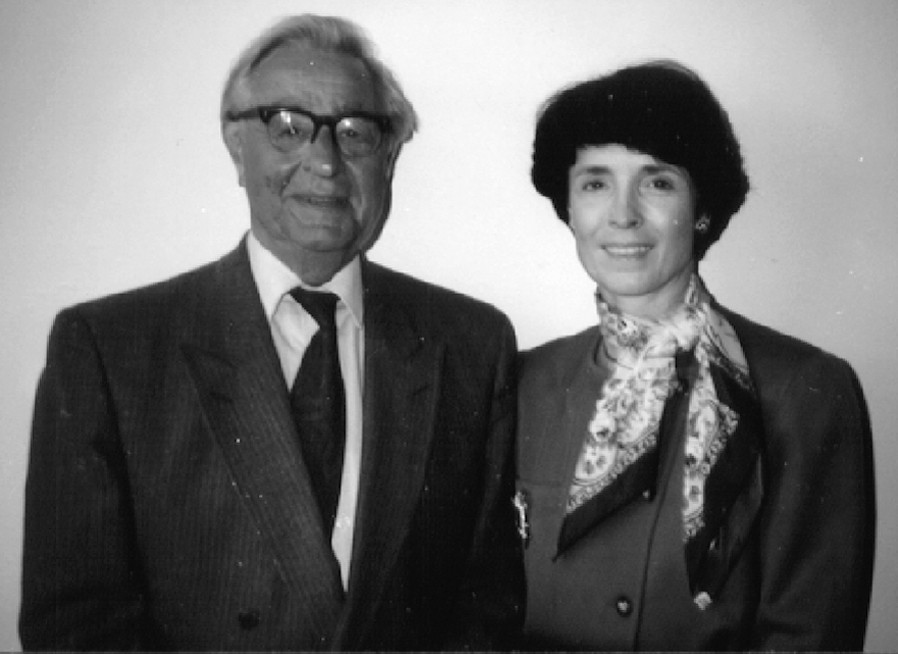
George Porter with Mary Archer, ca. 1974.
I set to work with my first research student, Isabel Ferreira, on photogalvanic cells [14], and with George wrote a review of physico-chemical methods of solar energy conversion, in which we discussed the progress made in recent years [15]. We also sketched out what needed to be done. We grumbled that lack of certainty as to where the pigment molecules are located in photosynthetic antennae and reaction centres was hampering attempts to build functional in vitro models (Deisenhofer, Huber and Michel's paper on the structure of the reaction centre of R. viridis was still some years away). We talked of the involvement of ‘a manganese complex about which relatively little is known’ in the oxygen evolution step of green-plant photosynthesis: how thrilled George would have been to read Jim Barber's Science paper of March 2004, which shows the cubane-like structure of the Mn3CaO4 cluster of Photosystem II to 3.5 Å resolution [16].
George also encouraged me to form the UK Section of the International Solar Energy Society, of which I became the founding Honorary Secretary. The inaugural meeting was held at the RI in January 1974, with George as one of the speakers, and the society rented an office under the eaves of the RI for some years thereafter. Years later, in 1991, George kindly took on the presidency of the National Energy Foundation, of which I was the founder chairman, a position he occupied until I succeeded him in 2000.
5 The Royal Society and the House of Lords
In 1985, George was elected President of the Royal Society, the foremost position in Britain for a scientist. In this capacity, he spoke out on several policy issues involving science, in particular criticising cuts in public funding. In his 1989 Royal Society Anniversary Address, he spoke thus of the complementarity of pure and applied science: “The only sure foundation of security in a technological world is to have a science base which is continually asking whatever questions seem interesting, and is always there to advise and act when the need arises”. George received the Order of Merit in 1989, and was made a life peer in 1990, taking the title Baron Porter of Luddenham.
6 George and IPS conferences
George had a long association with the IPS conference series. According to my records, he first became a member of the International Organising Committee (IOC) for IPS-3 in Boulder (1980). At the conclusion of his natural term of office, he was accorded the status of ‘permanent member’ of the IOC, and remained in that position as a supportive participant until his death. He gave the opening keynote talk, entitled ‘Sun, Energy and Chemistry’, at IPS-4 in Jerusalem (1982), in which he outlined ‘hopes and visions’ for the non-sacrificial dissociation of water with red light. At IPS-5 in Osaka (1984) (Fig. 8), he chaired the closing discussion on what directions future research should pursue. At IPS-6 in Paris (1986), he led a discussion on industrial applications of solar energy, in particular the prospects for amorphous and multicrystalline silicon solar cells and the possible genetic manipulation of plants to improve biomass yields. He gave a plenary talk entitled ‘Energy and Electron Transfer in Plant Photosynthesis’ at IPS-10 in Interlaken (1994), as well as co-authoring a paper with Jim Barber and others from Imperial College on the weakly multimeric state of P680 [17], not to mention singing tenor in the cabaret which I organised (Fig. 9).
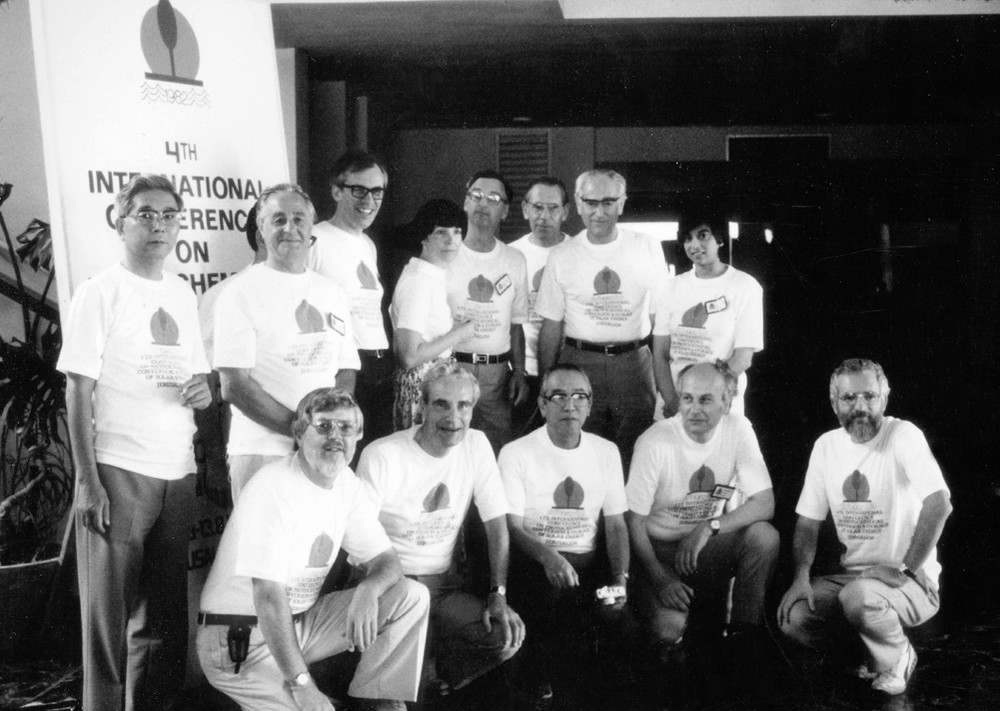
With other members of the IOC of IPS-5 in Osaka (1984). Back row, left to right: Hiroshi Tsubomura, George Porter, Jim Bolton, Mary Archer, Joseph Rabani, Wolfgang Sasse, Heinz Gerischer, Silvia Costa. Front row, left to right: John Connolly, Arnim Henglein, Kenichi Honda, René Bensasson, Art Nozik.
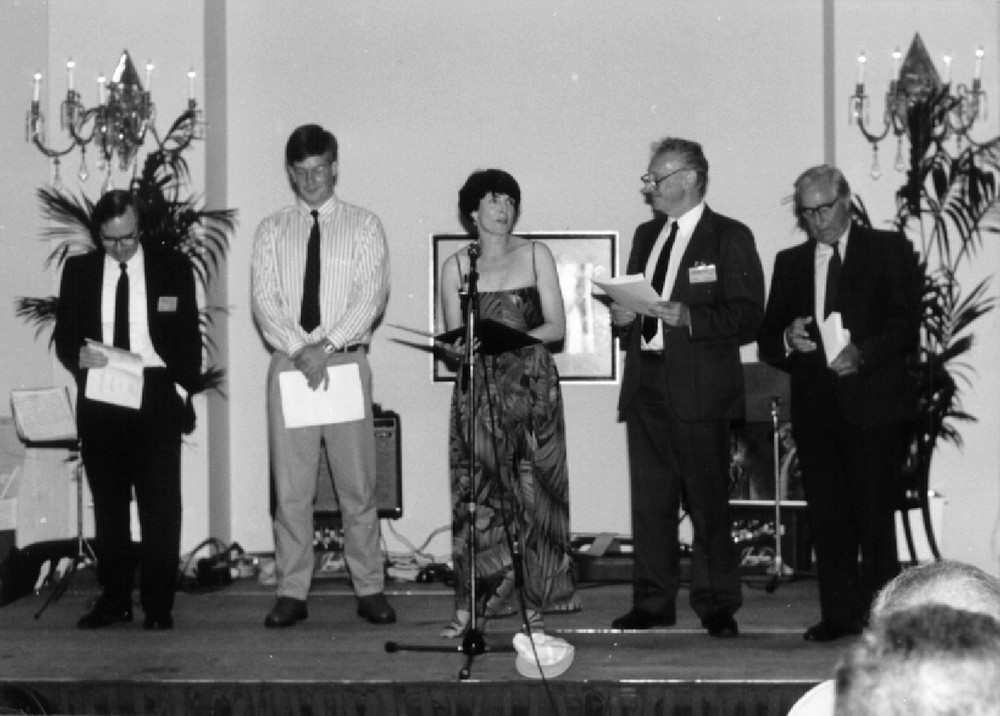
Line-up for the after-dinner cabaret of IPS-10 in Interlaken, 29 July 1994. From left to right: Frank Wilkinson, Colin Grubb, Mary Archer, John Kerry Thomas, George Porter.
7 Imperial College
Research was George's enduring passion, and he went back to it full-time after stepping down from the presidency of the Royal Society in 1990. On taking up this position, he had moved his research group (James Durrant, David Klug, Linda Giorgi, Paula Booth and Ben Crystall) from the RI to Imperial College, where in 1985 he was given an office and research space in the Department of Pure and Applied Biology. In 1987, he became Professor of Photochemistry at Imperial, and the Centre for Photomolecular Sciences, which he chaired from 1990 until his death, was created around him. In collaboration with Jim Barber's group, George spent the final phase of his scientific career at Imperial, studying the primary processes of photosynthesis. It gave him great pleasure that flash photolysis was just then taking its final step into the femtosecond time domain, enabling these processes, which are among the fastest known to chemistry or biology, to be studied. Over fifty years of active research, George was the key influence in lowering the frontier of the time domain that could be studied by flash photolysis by one million-million fold.
He died on 31 August 2002 in Canterbury, truly a peer among scientists.
1 This account is based on the entry on George Porter that I wrote for the 2004 edition of the Oxford Dictionary of Biography, and draws on material kindly provided by Lady Porter and her family, as well as published material.