1 Introduction
In 1991, O’Regan, and Grätzel [1] reported highly efficient dye-sensitized solar cells (DSC). Organic solar cells called bulk heterojunction solar cells used to have been thinned because of low diffusion length leading to low light absorption coefficient. The DSCs succeeded to increase the light absorption efficiency by utilizing the huge surface area of nanoporous TiO2 films as a nano-scale three-dimensional electrode where sensitizing dye molecules were adsorbed. Since the film was prepared from nanoparticles by sintering in air, the fabrication cost was expected to be much lower than the conventional solar cells, while the energy conversion efficiency showed comparable values with that of conventional amorphous silicon solar cells. Thus, since their publication, intensive research and development on DSCs have been carried out to understand the mechanism, and to improve the efficiency and long-term reliability of the solar cells.
DSCs typically consist of a dye-adsorbed nanoporous TiO2 film prepared on transparent conductive oxide. The film is immersed in an electrolyte containing a redox couple and placed on a platinum counter electrode. Here, the energy conversion efficiency is determined by (1) light harvesting efficiency, (2) charge injection efficiency, (3) electron transport and collection efficiency in TiO2 electrodes, and (4) hole transport and collection efficiency in electrolytes. As for light harvesting efficiency and charge injection efficiency, a series of ruthenium dyes with carboxylic groups show wide absorption and high injection efficiency.
An issue of sensitizing dyes is its cost performance. Since Ru is a rare metal of very low annual yield, ruthenium-free organic dyes have been desired. With regard to electron transport and collection efficiency, electron diffusion length (L), which is given by square root of product of electron diffusion coefficient (D) and electron lifetime (τ), is an important factor. To collect most of injected electrons, L must be at least the thickness of the TiO2 electrodes. The thickness is determined by the light absorption coefficients of the dye-adsorbed electrodes. Hole transport and collection efficiency depend on the dye-cation reduction efficiency, conductivity in the bulk electrolytes and the I–/I3– redox efficiency at counter electrodes.
Highly efficient DSCs employ an I–/I3– redox couple and organic liquid solvents. However, the long-term durability of the cells is low due to the difficulty of the sealing of such volatile electrolytes and sublimable I3–. This problem must be solved for long-term reliability in commercialization. Thus, large efforts have been made to replace the liquid solvents and the redox couple with solidified or gelled electrolytes. On the other hand, alternative hole transport systems containing another redox couple like Cobalt complexes may result in shorter electron lifetime, and consequently shorter diffusion length. Here, evaluation and mechanistic understanding of charge transport is needed to design DSC's giving long enough electron diffusion length.
To solve the issues mentioned above, our group and collaborators have been working on replacing organic liquid electrolyte, synthesizing organic dyes, and studying the properties of electron transport in nanoporous TiO2. In this proceeding, the overview of the achievements during the course of the research works is given.
2 Alternative dyes
In general, metal free organic dyes have higher absorption coefficient compare to the metal complexes and can be tuned to wide range of absorption spectrum. In addition, the sensitizing dyes with narrower absorption band lead to colorful and thinner DSCs with improving fill factor. For better performance of DSC, metal-free organic sensitizers must have the following features: (1) Extended π-conjugated system reducing the HOMO-LUMO gap. (2) Appropriate redox potentials of LUMO and HOMO levels towards TiO2 conduction band level and electrochemical potential of redox couples in the electrolytes. (3) Anchoring group(s) which can chemically react with the TiO2 surface. (4) Intramolecular charge transfer (CT)-type absorption that derives from electron donating part to the electron accepting part that bears anchoring groups in the dye molecules. (5) Chemical stability towards to the chemicals in electrolytes.
For several years, Japanese groups have made great contribution to develop novel organic dyes. Dr. Arakawa and his group reported benzothiazole merocyanines [2,3], coumarin dyes [4–7] and polyene molecules [8] as shown in Scheme 1. Merocyanines form J-aggregates on the surface of TiO2 to have strong absorption in red light region. Absorption range of coumarine and polyene was expanded to red region by introduction of methine and oligothiophene units. More recently, Mitsubishi Paper Mills Ltd. and Tohoku University reported novel dyes containing indoline unit (Scheme 2) [9,10] to expand the absorption band. The reported conversion efficiency of 8.00% is the record in organic dye based DSC. The molecular designs bring high and wide absorptivity of the dyes and vectorial electron transfer and long-term durability in the DSC system.
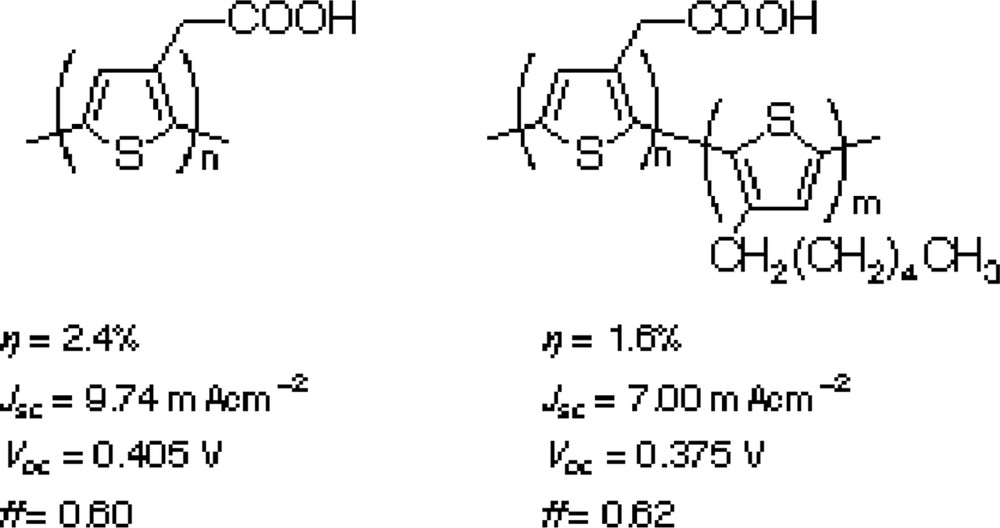

Nippon Kayaku Co., Ltd. and our group also examined polyene dyes and proved that the polyene dye (Scheme 3) gave the conversion efficiency of 6.6% with > 80% IPCE. Collaboration with Prof. Lian of Emory University in USA revealed the ultra fast electron injection to the TiO2 electrode (Fig. 1) [11].
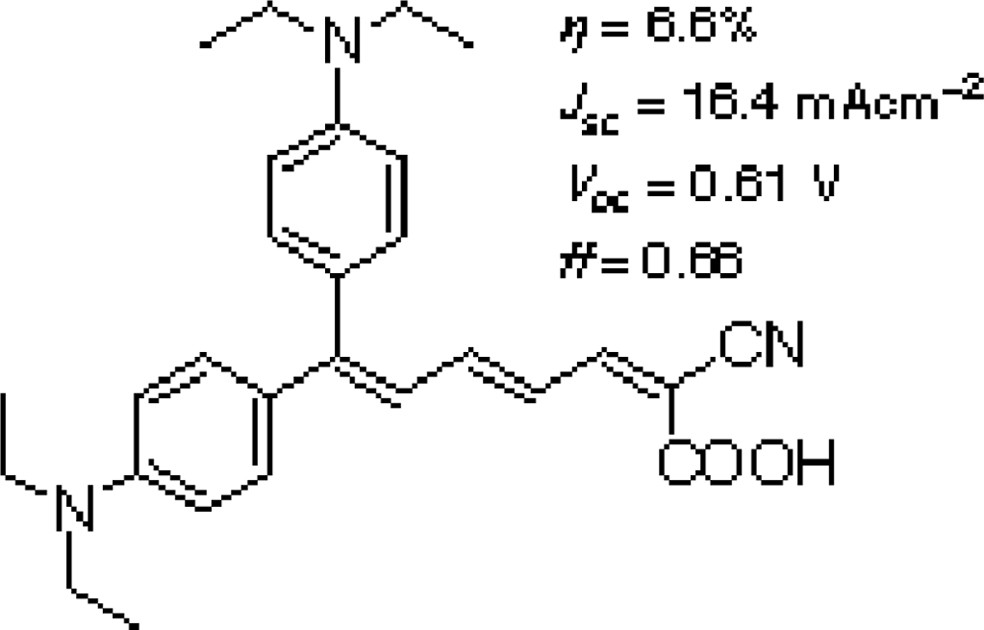

Transient mid-IR absorption of polyene dye-adsorbed mesoporous TiO2 for probing the injected electron signal at 2000 cm–1 after femtosecond 400-nm excitation.
Our group also examined the possibility of application of conjugated polymer as the sensitizer. Polythiophenes with carboxylic group shown in Scheme 4 were applied to mesoporous TiO2 and SnO2/ZnO composite electrodes [12,13]. Although it takes several days for complete penetration of dyes molecules into nano-scale pores of the electrodes, polythiophene-based DSC shows 2.4% and 1.5% conversion efficiencies for respective TiO2 and SnO2/ZnO electrodes. This system can be achieved by enhancing the electron diffusion coefficient of nano-metal oxides electrodes by introducing imidazolium cations on the metal oxide surfaces.
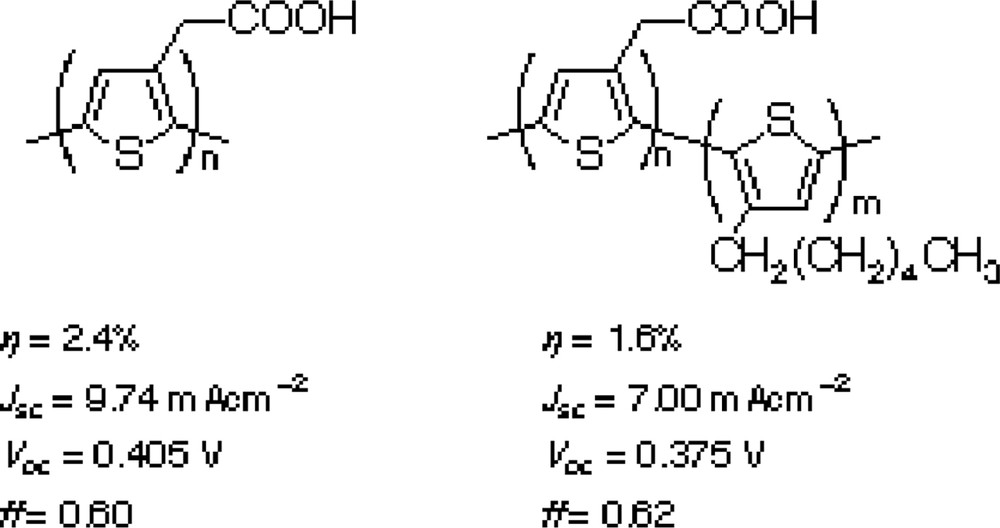
3 Hole transport layer
The requirements for the hole-transport materials are effective reduction of oxidized dye cations, fast enough hole transportation and methods to fill them into the nano-sized porous space of electrodes. To satisfy these and to achieve high conversion efficiency, an electrolyte containing high concentration of I–/I3– redox couple in fluid organic solvents has been used. On the other hand, as it was mentioned in the introduction, the high vapor pressure of organic solvents and iodine has been the reason of low durability of DSCs. A solution would be to replace the liquid with solid p-type semiconductor materials [14]. However, no materials satisfying the above criteria have been found. Another strategy will be gelation of the liquid electrolytes containing the I–/I3– redox couple, and replacement of the liquid electrolytes with room temperature molten salt (ionic liquid) or the gelation of ionic liquids [15–18].
We have fabricated DSCs using ionic liquid consisting of imidazolium cations having various alkyle chains [15]. Improved durability was found from these DSCs, but the energy conversion efficiency could not reach that of DSCs using liquid electrolytes. This was mainly because that the viscosity of the ionic liquids was higher than that of liquid solvents. This fact required higher concentration of I–/I3– redox couple to provide enough conductivity. On the other hand, the higher concentration of the redox couple increased the undesired light absorption, and also facilitated the charge recombination of the electronsin the TiO2 with the I–/I3– redox.
Ionic liquids can be gelled by the co-dispersion of nanoparticles with the ionic liquids. It has been reported that the gelation by silica particles does not decrease the efficiency of DSCs [18]. We have also tried various materials of nanoparticles for the geleation of ionic liquid (Table 1), and found that the dispersion of nanoparticles, especially TiO2, increased the energy conversion efficiency. The diffusion coefficients of I–/I3– ions in the gelled ionic liquid were also measured, showing the increases of the conversion efficiency values. This can be interpreted as due to the increased ion exchange mechanism, i.e. Grotthuss mechanism [15,19]. The largest increase was found when TiO2 particles (P-25) were employed as a nano-particle gelator, impling that adsorption of imidazolium cations on the TiO2 [18b] would align the anionic redox couple by electrostatic force, facilitating electron transport by the ion exchange mechanism (Fig. 1). At the moment, influence of the particle size could not be observed. This may be due to the comparable aggregation trend of the particles, and we expect the better performance once the mono-dispersion methods were developed for organization of nanoparticles like morphology of photonic crystals (Fig. 2).
Performance of Solar cells having different nanoparticles for gelation
Nanoparticles for gelation | Jsc (mA cm–2) | Voc (mV) | FF | η (%) |
TiO2 | 12.5 | 696 | 0.65 | 5.7 |
SnO2 | 12.1 | 679 | 0.61 | 5.0 |
SiO2 | 12.5 | 719 | 0.64 | 5.8 |
ITO | 12.5 | 685 | 0.60 | 5.1 |
Carbon black | 11.0 | 672 | 0.65 | 4.8 |
MW-CNT | 12.0 | 706 | 0.57 | 4.8 |
Ionic liquid | 11.8 | 661 | 0.60 | 4.7 |
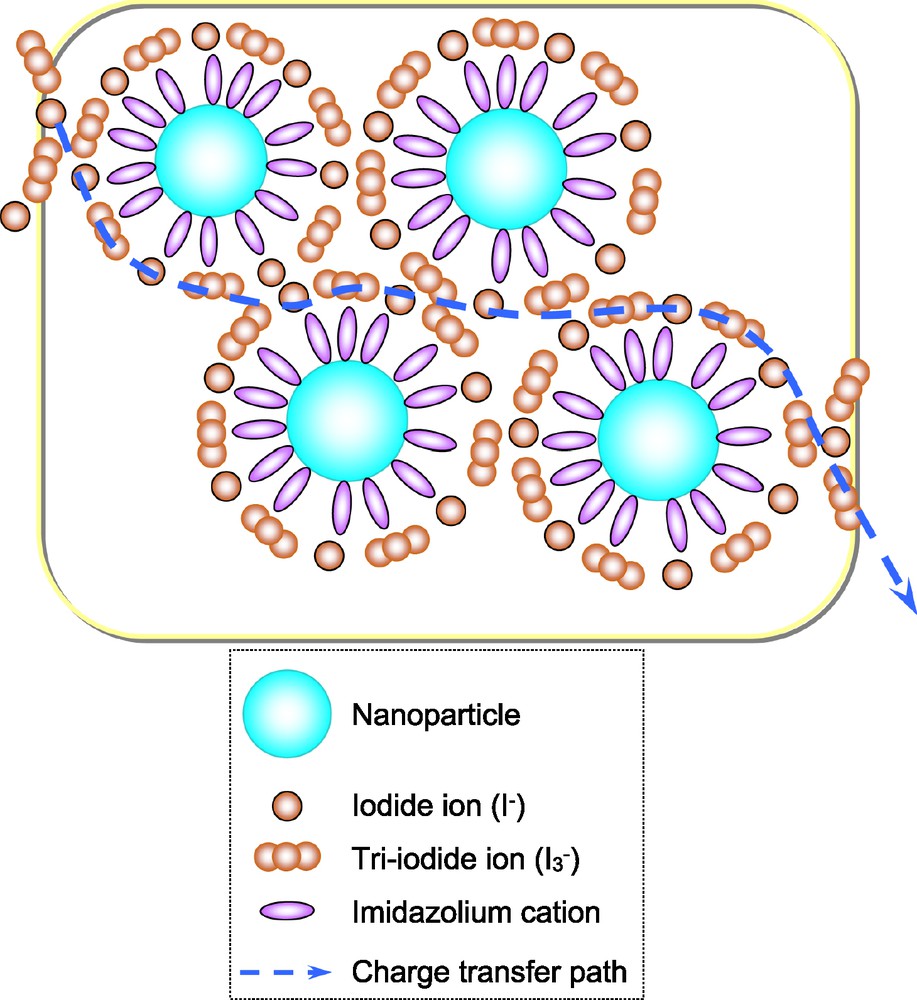
Schematic drawing of a proposed charge transport mechanism in the nano-composite ion-gel electrolyte.
4 Electron transport in nanoporous TiO2
In DSC, the electron transport can be characterized with diffusion and recombination. Since the cations in the electrolyte solution surround the electrons in the nanoporous TiO2, large electric field gradient cannot be formed in the TiO2 film. Thus, the transport should occur mostly with diffusion. The recombination can occur with I3– and oxidized dye cations during the diffusion. The diffusion coefficient and lifetime of electron in TiO2 have been examined by several groups [21–27]. The measured diffusion coefficients showed power-law dependence on electron density. This has been modeled with the events of trapping/de-trapping by intra-band charge traps [24,27]. The measured electron lifetime showed inverse power-law dependence. Apart from the diffusion coefficients, several models have been proposed to explain the observation of the long electron lifetime in TiO2 [22,23,27,28].
4.1 Measurement methods
The electron diffusion coefficients and lifetime can be measured by photo-current or photo-voltage response against change of incident light intensity. The perturbation method of light intensity can be chosen arbitrary. Relationship between D and the current response is obtained by solving the diffusion equation, and the D can be obtained by fitting techniques. Most of groups have used delta (pulsed laser) or sinusoidal function (modulated diode laser or LED) for the perturbation. One concern is that the D itself has dependence on electron density, in other words, light intensity. This means that the diffusion equation should be solved with taking into account of the dependency, or the measurement should be performed under the condition that the D can be approximated to be a constant. The later evaluation was achieved by measuring current response against change of partial light intensity. On the other hand, measurements under continuous light irradiation limit the sample condition, i.e. the sample needs hole transport layer, and should be sensitive against the irradiate light. These required conditions make it difficult to study some influences such as the influence of dye-adsorption. To check the influence of continuous light irradiation, identical samples were measured with and without bias light. Against the expectation, measured values showed the same order and tendency regardless of the bias light (Fig. 3). The result showed also that both methods are valid for semi-quantitative analysis, but both results contain some error and need further development for more quantitative analysis.
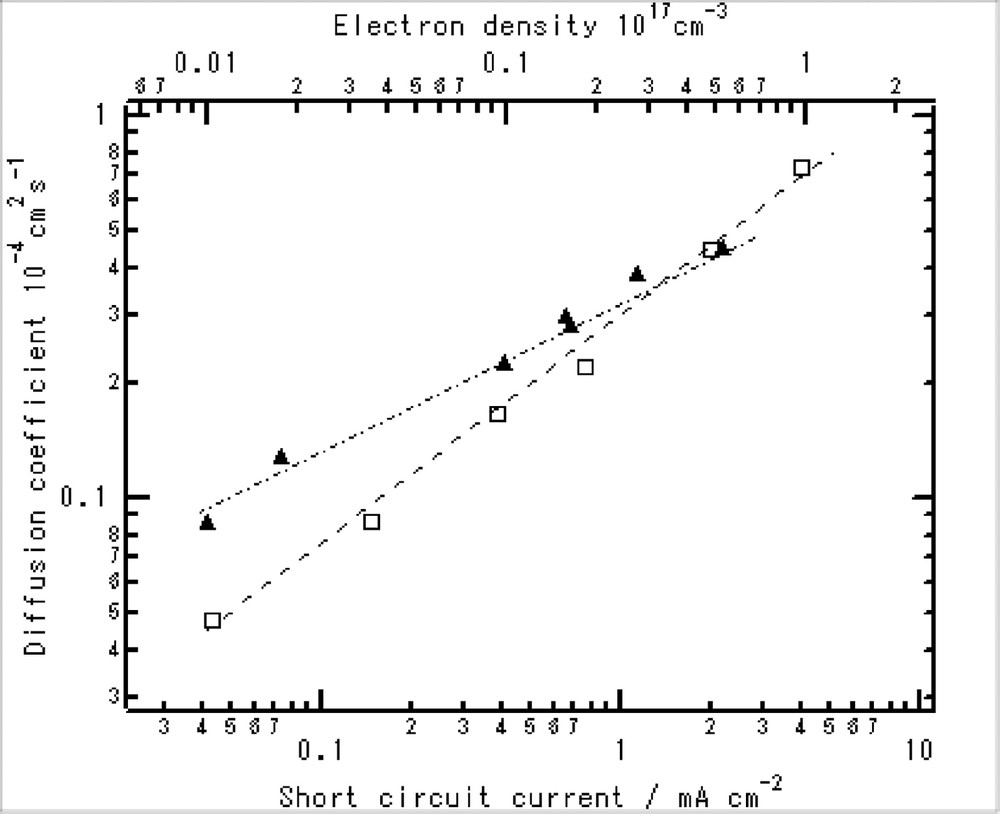
Electron diffusion coefficients in dye-adsorbed nanoporous TiO2 films. The values were obtained by a UV laser pulse induced current transients (closed triangle) and by a green pulsed laser under a continuous light irradiation (open square).
Electron lifetime has been measured by photovoltage response at open-circuit conditions. Typically, small intensity sinusoidal moderated light with large and steady intensity light is used [23]. The photovoltage response is plotted on a complex plane and the frequency giving the minimum of the imaginary components is related with the electron lifetime.
4.2 Parameters influencing diffusion length, i.e. D and τ
4.2.1 Diffusion coefficients, D
As it was mentioned, intra-band charge traps largely influence the electron diffusion coefficients. In addition to this, since the TiO2 film consists of unified TiO2 nano-particle regarded as network of nano-TiO2 wires, the conditions and number of boundary among the particles should influence the D. These suggest that the diffusion coefficients would be significantly influenced by synthesis methods of TiO2 particles, particle size, and film calcination temperatures. Actually, large values of D were observed from the electrode consisted of better crystalline particles and larger particle size, and the electrodes prepared at higher calcination temperatures [25,29]. These can be interpreted as due to less number of deep charge traps and crystalline boundaries.
Another prominent feature of the TiO2 electrode is its large surface area. This leads to the expectation that the surface conditions also influence the D. Typically, several cationic species in electrolytes and dye molecules are adsorbed or anchored on the surface TiO2. We have measured the influence of such cations in the electrolyte, and found that the adsorptive cationic species increase the values of D [20]. We have also measured that the influence of dye adsorption and found that the adsorption also increase the D (Fig. 4) [26]. The rate of the increase was nearly proportional to the surface area. This result can be interpreted as due to that the surface charge traps are filled by the adsorption or reaction of carboxyl groups. On the other hand, no influence on the electron density dependence implies that shallow traps still exist in the nano-net-wire-like TiO2 electrodes.
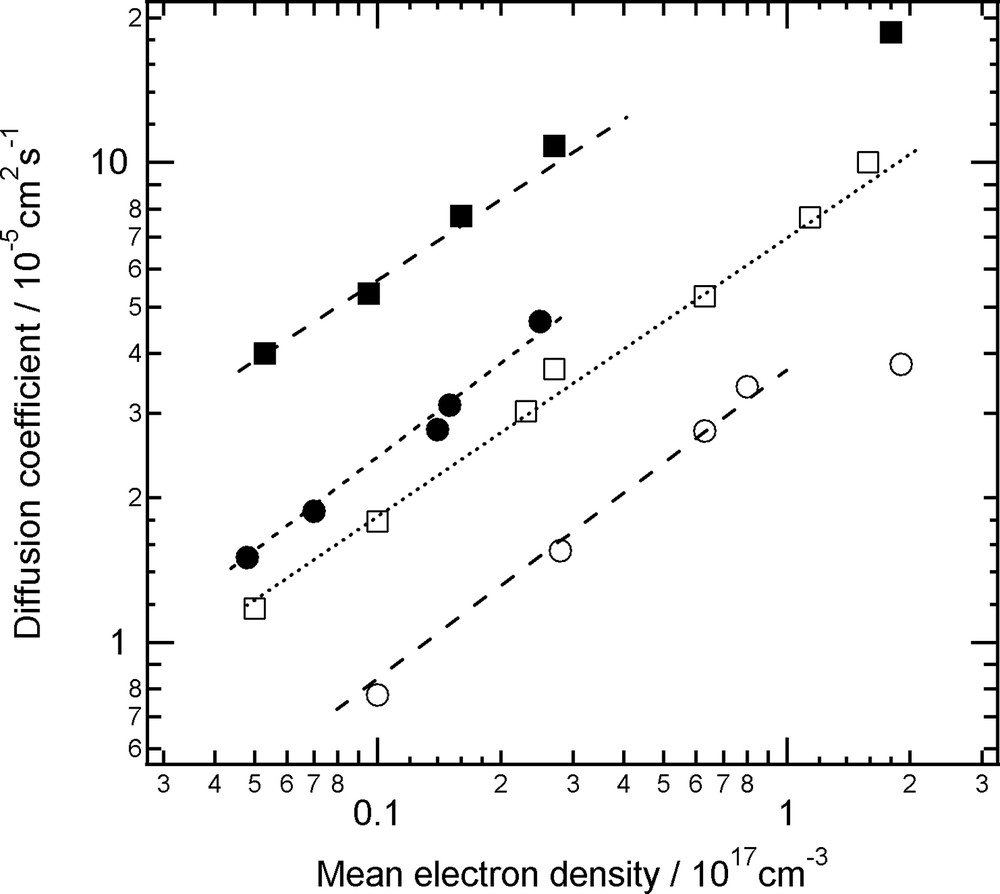
Electron diffusion coefficients in nanoporous TiO2 films with (closed) or without (open) dye adsorption. Electrolytes were 0.7 M of LiClO4 (square) or 0.5 M of TBAClO4 in acetonitrile.
4.2.2 Electron lifetime
Electron lifetime would be related with the density of change traps and/or the energy level of the charge traps. One of important questions is if the charge traps are recombination center. So far, the TiO2 films having larger values of the D tend to have shorter lifetime [25,30]. This suggests that the electron lifetime is limited by the electron transport and most of the traps are not recombination center. This implies also that, in view of electron diffusion length, the slow electron diffusion in DSC is intrinsically compensated by the long electron lifetime because the origin is the same. However, slow electron transport caused by electron scattering at particle boundaries or deep traps cannot be compensated by the trap related electron lifetime. In particular, the boundary conditions such as the number and cross section area, should be paid more attention to increase electron diffusion length.
In DSC, all recombination occurs through the electron transfer at TiO2/dye/electrolyte interfaces. To increase the electron lifetime, increasing the distance or energy barriers between dyed TiO2 and the electron acceptors like I3– is one strategy. The addition of thin metal oxide layer on TiO2 films has been shown to increase the electron lifetime [31]. In view of electrolytes, several species of cations and additives are dissolved to improve the performance. tert-Butylpyridine has been known to increase the open-circuit voltage. The increase could be interpreted with the increase of electron lifetime as due to the adsorption of such bulky compound onto the TiO2 surface. However, this postulation was denied by the electron lifetime measurements [23]. In addition, what is the role of the cationic species in electrolytes? Do they increase or decrease the electron lifetime?
To answer the questions, we measured electron lifetime in DSC employing various compositions of electrolytes [32]. Fig. 5 shows some of results. It was found that DSC using TBA+ showed the longer lifetime than DSCs using Li+ and DMPIm+. The lifetime was independent from the concentration of the cations at least above that of 0.1 M. Here, the interfacial electron transfer rate depends on the difference of free energy, the distance between I3–and dyed TiO2, and reorganization energy between the electron in TiO2 and I3–, and the concentration of electrons and I3–. As for the effect of TBA+, it has been reported that the cations are too large to penetrate into dye molecules on TiO2, while smaller cations would do. This difference will change the thickness of electrical double layer on the TiO2. Since the concentration of I3– in the vicinity of the double layer should be lower than that in the bulk electrolyte solution, thicker double layer will result in improvement of the longer lifetime. On the other hand, for the DSCs employing a cationic redox couple, e.g. Co(dtb-bpy)3, the addition of Li cations resulted in the longer lifetime of electrons due to the relative decrease of cationic redox concentration on the electrical double layer of the surface (Fig. 6) [32].
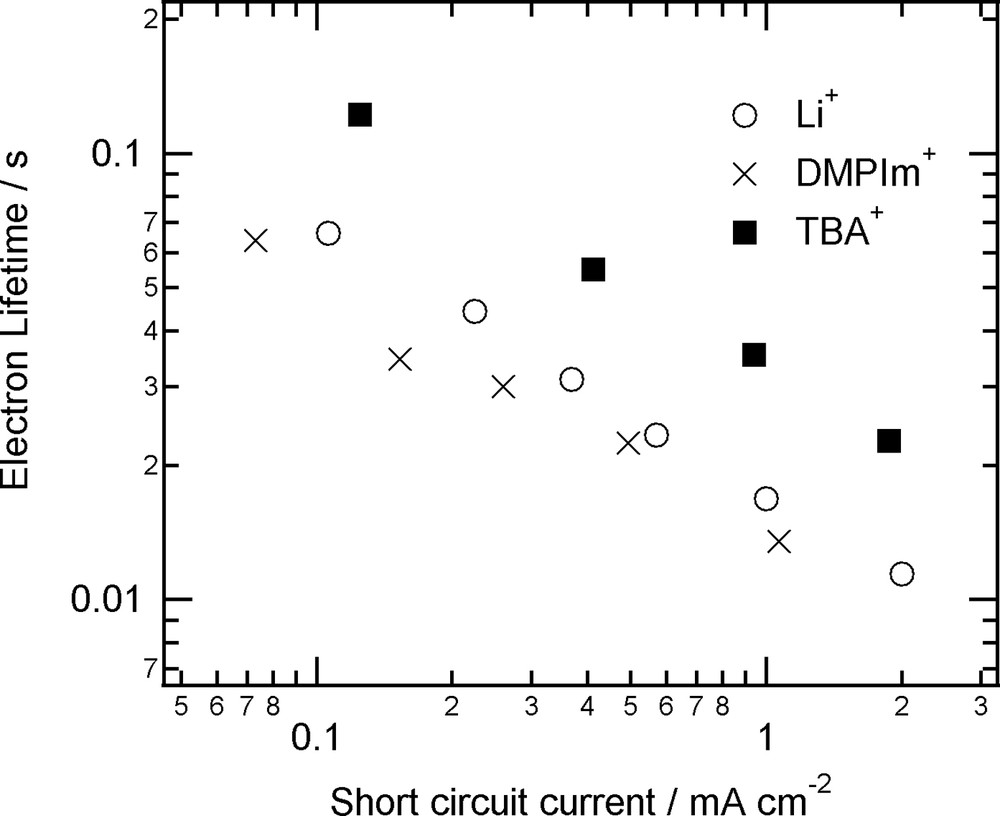
Electron lifetimes in DSCs employing different cations as a counter charge of I–/I3–.

Electron lifetime in DSCs using a Co redox couple with various concentration of Li cations.
4.3 How to improve diffusion length
As it was mentioned, the increase of D would results in the decrease of τ, ending little influence on the diffusion length. Thus, the diffusion length should be improved for example, by the decrease of electrical resistance of the TiO2 particle boundaries. This could be realized by reducing the amount of amorphous state from the TiO2 particles, and by optimizing the cross section area of particle-particle boundaries.
In view of electrolyte, adsorption of Li+ cations and imidazolium cations in electrolytes especially increases the D. On the other hand, non-adsorptive bulky cations like TBA+ increase the electron lifetime. Thus, the admixture of several cationic species at appropriate concentration ratio is desired to increase the L, leading to increase not only of incident photoelectron efficiency (IPCE) but also of fill factors.
4.4 How to improve the performance of dye-sensitized solar cells
The energy conversion efficiency of DSC is never determined only by electron diffusion length. Photocurrent of short circuit, Jsc, and open circuit voltage, Voc, must be increased at the same time. To obtain high Jsc's, Li+ seems to be needed with balance of diffusion length and electrode thickness. On the other hand, Li+ is not desired in view of open-circuit voltage, and thus, the minimum amount of Li+ should be dissolved, and the rest of counter cations of I– and I3– should be chosen from others. The surface conditions of the unified nano-TiO2 particle also influence flat band energy level, thus, the TiO2 electrodes in DSC should also be optimized from both the diffusion length and interfacial structures of these nano-net-wire-like TiO2 electrodes.
5 Conclusions
Dye-sensitized solar cells have multi-pn junctions, composing of unidirectional charge separation devices using dye molecules as a diode. Nano-TiO2 layers can be regarded as excellent and inexpensive nano-net-wires for collecting photoelectron from sensitizing dye molecules. The research on DSCs as death-valley-free devices is advancing competitively both in academic and in industries. Recent two special issues of the Journal of Photochemistry and Photobiology A are also reporting the fruitful research progresses especially in Japan [33]. At IPS-15, Professor Dai and his group of Institute of Plasma Physics in China reported photoelectric conversion efficiency around 6% with 15 × 20 cm2 module. This is the highest conversion efficiency for the large scale DSC tested for outdoor practical use. Further effort for reliability at temperature as high as 80 °C will approve of DSC’s feasibility as low cost solar cells for outdoor long-term use.
Acknowledgements
The author could introduce here some works on DSC that could be accomplished by the following scientists and their group; Professor Yuji Wada and Dr. Takayuki Kitamura, Dr. Yasuchika Hasegawa (Osaka University), Dr. Shogo Nakade (Nokia Research Center), Mr. Teruhisa Inoue (Nippon Kayaku Co.), Professor Tim Lian (Emory University in USA), Dr. Nobuo Tanabe (Fujikura Co.), Professor Masayoshi Watanabe (Yokohama National University). This proceeding manuscript was written through collaboration with Dr. Shogo Nakade, Dr. Takayuki Kitamura and Dr. Nobuo Tanabe. The author also greatly appreciates financial supports form NEDO and Ministry of Education, Science and Culture of Japan.