1 Introduction
Compounds derived from the coumarin (1,2-benzopyrone) ring system constitute one of the main families of organic fluorescent materials, and they are of considerable importance for laboratory and industrial use [1–3]. Among them are the most currently used laser dyes for the blue-green region. They also provide enzyme substrates for fluorimetric titrations in biomedical analysis, and are at the basis of numerous fluorescent probes and advanced photophysical systems. At a wider scale, coumarin derivatives are intensively used for the colouring or fluorescent whitening of textiles and other materials. In contrast, the neighbouring series of iminocoumarins [4–10] has surprisingly received very little attention until now. However, iminocoumarins are versatile compounds, which can be easily modified by substitution on the imino group. This allows various groups, such as fatty chains, to be introduced on the fluorescent moiety, and gives access to a new generation of fluorescent compounds, designed for specific purposes. An interesting example is the design of a Ca2+ indicator, in which an iminocoumarin group both acts as a signalling unit and bears a lipophilic chain that bestows on the sensor strong affinity for cell membranes [11,12]. By comparison, introducing an alkyl chain in a coumarin is a tedious work.
The easy preparation of iminocoumarin derivatives makes them very attractive, but it is also important to know whether their spectroscopic properties can compete with those of coumarins, which often are intensely coloured, brilliant dyes, with high molar extinction coefficients and high quantum yields. To our knowledge, very few optical studies deal with iminocoumarins. For instance, Karasev et al. [6] have reported that 2-benzimidazolyl-iminocoumarin was less fluorescent than its coumarin analogue (the fluorescence quantum yield being, respectively, 0.03 and 0.19 in acetonitrile), and that this is also the case when the compounds are substituted by a diethylamino group at the 7-position. Consequently, these authors have concluded that no efficient luminophores are encountered to date among 7-diethylamino-iminocoumarins [13]. However, it seems possible to us that the behaviour observed is linked to the presence of the benzimidazolyl fragment, because immobilization of the latter by subsequent reaction leads to highly fluorescent dyes [13,14]. In our team, we recently reported the synthesis of 10 new iminocoumarins that bear a cyano group as an electron-withdrawing moiety at the 3-position [15]. A preliminary spectroscopic study was carried out in dichloromethane [16]. It revealed that among all the compounds considered, those bearing a strong electron-donor group, that is a diethylamino group, at the 7-position displayed superior fluorescence properties. These compounds are dye 1 that bears a free imino group, and dye 2 that is the corresponding N-ethoxycarbonyl derivative (Fig. 1). The aim of the present paper is to report their spectroscopic and photophysical properties studied in several different solvents, and to make a comparison with the behaviour of the corresponding coumarin derivative, 3. To do so, the three dyes were studied by UV–vis absorption spectroscopy and by steady-state fluorescence spectroscopy, and the data were processed using classical solvatochromic scales.
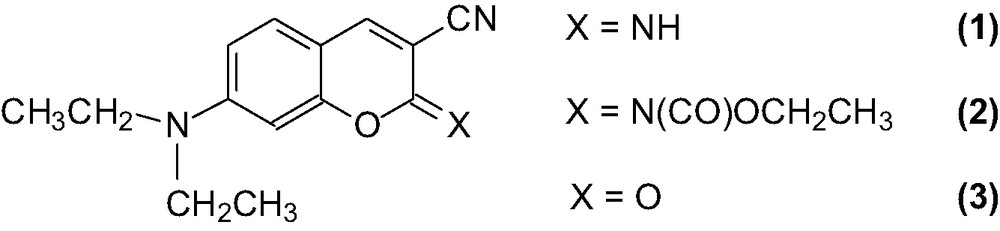
Chemical structure of the iminocoumarin (1 and 2) and coumarin (3) derivatives.
2 Experimental
2.1 Materials
Spectrometric and analytical grade solvents from Prolabo and SDS were used without further purification for spectrophotometric measurements. Absolute ethanol and dimethylsulphoxide were from freshly opened bottles and contained less than 0.1% and 0.01% water, respectively, as specified by the manufacturer. Ultrapure water with a resistivity of 16 MΩ cm was produced using a Milli-Q apparatus (Millipore). Dyes 1–3 were synthesized as previously described [15]. Dye 1 resulted from the condensation of o-hydroxybenzaldehyde with an equimolecular amount of malononitrile in the presence of piperidine as the catalyst, and was recrystallised in ethanol. Dye 2 was obtained by reacting 1 with ethyl chloroformate, and was recrystallised in methanol. Dye 3 was prepared by hydrolysis of 1 in a mixture of acetic acid and ethanol (30:70 v/v). After heating during 1 h, the reaction mixture was left to cool down, yielding crystals of 3.
2.2 Preparation of the solutions
The three dyes were readily soluble in organic solvents. In water, dye 1 dissolved slowly. In contrast, dyes 2 and 3 required previous dissolution in a tiny amount of diethyl ether before being placed into water. The aqueous solution was then bubbled with nitrogen and gently heated under stirring to remove traces of ether. It was checked on compound 1 that the same absorption and fluorescence spectra were obtained whether ether was used to dissolve the dye or not. All solutions were filtered on paper filter before spectroscopic measurements.
2.3 Apparatus
The measurements were conducted at 25 °C in a temperature-controlled cell holder. UV–vis absorption spectra were recorded on a Hewlett-Packard 8452A diode array spectrophotometer. The experimental error was estimated to be 2 nm on the band maximum. Steady-state fluorescence work was performed on a Photon Technology International (PTI) Quanta Master 1 spectrofluorometer. All fluorescence spectra were corrected. The fluorescence quantum yields (Φ) were determined using the classical formula: Φx = (As × Fx × nx2 × Φs)/(Ax × Fs × ns2) where A is the absorbance at the excitation wavelength, F is the area under the fluorescence curve, and n is the refractive index. Subscripts s and x refer to the standard and to the sample of unknown quantum yield, respectively. Coumarin 6 in ethanol (Φ = 0.78) was taken as the standard [17]. The dipole moments were calculated using the Accelrys software (AMPAC/MOPAC program, version 2001) on a Silicon Graphics Indigo 2 R10000 station. The program Origin 6.0 (Microcal ™ Software) was used for multiple regression analyses.
3 Results and discussion
3.1 UV–visible absorption spectra
The three dyes were prepared as previously described [15], and their absorption spectrum was recorded in 18 different organic solvents, in which they were readily soluble. The dye concentration was between 2 × 10–5 and 3 × 10–5 M. Whatever was the solvent used, the absorption spectrum exhibited an intense band at long wavelengths. This band can be attributed to charge transfer (CT) that occurs between the electron donor group, i.e. the diethylamino group, and the electron-withdrawing pole, constituted by the carbonyl or imino group, and by the cyano group that reinforces the electron-attracting strength on this side of the molecule. As an example, the spectra recorded in toluene and dimethylsulphoxide are given in Fig. 2. Let us recall that the molar extinction coefficient ε, previously measured in dichloromethane at the maximum of the CT band, was found to be 41 200, 39 400 and 46 600 M–1cm–1 for 1, 2 and 3, respectively.
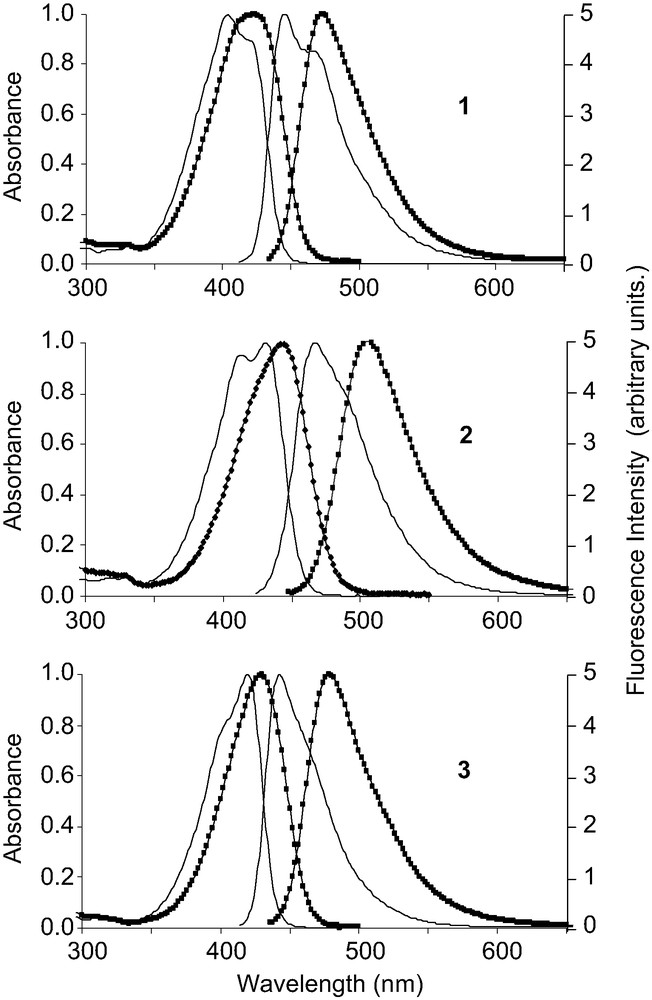
Normalized UV–vis absorption and emission spectrum (λex = λabsmax) of dyes 1–3 (around 2.4 × 10–5 M for absorption and 1.2 × 10–6 for emission) dissolved in toluene (plain line) and dimethylsulphoxide (squares).
For compound 1, the shape of the CT band varied strongly with the solvent (Fig. 2). In low-polarity solvents, it showed a peak at short wavelengths, followed by a shoulder at long wavelengths. In contrast, in strongly polar solvents, the maximum was situated at long wavelengths. For compounds 2 and 3, whatever the solvent, the CT band showed a shoulder at short wavelengths and a peak at long wavelengths. In some cases, for example for 2 in toluene, the shoulder was quite strong and formed a second maximum. In strongly polar solvents, the CT band of all compounds was unresolved. All spectral characteristics are gathered in Table 1.
Maximum UV–vis absorption (λabs) and emission (λem) wavelengths. When two values are given, the value in italics corresponds to a shoulder or to a peak of minor intensity, and the underlined value is the one that was used in the calculation. Taft and Kamlet π*, α and β parameters (from Ref. [18]). a: The solvent parameters were taken identical to those of 1-butanol
Solvent | 1 | 2 | 3 | π* | α | β | |||
λabs | λem | λabs | λem | λabs | λem | ||||
p-Xylene | 402, 416 | 444, 463 | 410, 430 | 466 | 404, 418 | 441, 458 | 0.43 | 0.00 | – |
Butyl acetate | 404, 416 | 456 | 418, 430 | 486 | 418 | 457 | 0.46 | 0.00 | – |
Ethyl propionate | 404, 416 | 454 | 414, 429 | 482 | 417 | 456 | 0.47 | 0.00 | 0.42 |
1-Butanol | 424 | 466 | 437 | 504 | 424 | 468 | 0.47 | 0.79 | 0.88 |
2-Butanol a | 422 | 464 | 436 | 499 | 424 | 466 | – | – | – |
2-Propanol | 422 | 465 | 436 | 501 | 424 | 467 | 0.48 | 0.76 | 0.95 |
1-Propanol | 425 | 467 | 438 | 504 | 425 | 470 | 0.52 | 0.78 | – |
Toluene | 404, 416 | 445, 466 | 412, 432 | 469, 484 | 402, 418 | 444, 460 | 0.54 | 0.00 | 0.11 |
Ethanol | 424 | 468 | 438 | 505 | 424 | 472 | 0.54 | 0.83 | 0.77 |
Dioxane | 402, 416 | 448, 465 | 412, 430 | 475 | 416 | 450 | 0.55 | 0.00 | 0.37 |
Ethyl acetate | 404, 416 | 454, 468 | 416, 430 | 482 | 418 | 458 | 0.55 | 0.00 | 0.45 |
Tetrahydrofuran | 406, 418 | 456, 473 | 416, 432 | 487 | 418 | 460 | 0.58 | 0.00 | 0.55 |
Benzene | 406, 416 | 446, 466 | 414, 432 | 470 | 406, 422 | 445 | 0.59 | 0.00 | 0.10 |
Methanol | 424 | 469 | 440 | 508 | 426 | 474 | 0.60 | 0.93 | 0.62 |
Acetonitrile | 418 | 465 | 436 | 497 | 424 | 475 | 0.75 | 0.19 | 0.31 |
Pyridine | 421 | 464 | 426, 442 | 496 | 429 | 470 | 0.87 | 0.00 | 0.64 |
Dimethylformamide | 422 | 470 | 440 | 502 | 426 | 478 | 0.88 | 0.00 | 0.69 |
Dimethylsulphoxide | 424 | 473 | 442 | 506 | 430 | 480 | 1.00 | 0.00 | 0.76 |
3.2 Fluorescence spectra
For all fluorescence measurements, the dye concentration was around 1.2 × 10–6 M and absorbance at the excitation wavelength was below 0.05. In our previous work where the dyes were studied in dichloromethane, we showed that the shape of the emission spectrum did not depend on excitation wavelength, which indicates that only one species is fluorescent in solution [16]. In the present work, we chose to record the emission spectra by exciting at the absorption maximum. The emission characteristics are collected in Table 1.
The emission spectra of the three dyes were unresolved and unsymmetrical, with a tail extending to long wavelengths (Fig. 2). Only in the less polar solvents could a shoulder be distinguished at the right side of the peak. The shape of the emission spectrum was quite similar for the three dyes. It must be noted that the emission spectrum was the mirror image of the absorption spectrum for compound 3 in all solvents investigated, but this was only true for 1 and 2 in polar solvents. Curiously, in low-polarity solvents, the emission spectrum did not reflect the absorption spectrum, and this was particularly noticeable for compound 1.
3.3 Analysis of the absorption and emission data
When examining Table 1, it appears that for the three dyes the absorption and emission spectra were shifted to the red with increasing solvent polarity. This effect called positive solvatochromism can be analysed by using polarity scales. Among many available polarity scales, the Taft and Kamlet scale offers the distinct advantage of separately assessing the influence of both the polarity and hydrogen-bonding capacity of the medium [18,19]. It uses the empirical parameters π*, α, and β, which, respectively, measure the solvent dipolarity/polarizability, hydrogen-bond donor acidity, and hydrogen-bond acceptor basicity. The Taft and Kamlet equation relates these parameters to the absorption or emission data, expressed in wavenumber (). It can be written as:
(1) |
(2) |
The spectroscopic data must be processed according to these equations. It is usual practice to take the band maximum, as long as the same spectral bands are considered [20]. However, the absorption spectrum of compound 1 varied strongly in shape from one solvent to another, and considering the absorption maximum lead to a shift that did not correspond to the actual shift of the whole spectrum. Consequently, in this case, we decided to consider the long-wavelength shoulder of the absorption spectrum, instead of the most intense peak, for the aprotic solvents of low polarity. In this way, the set of data obtained gave an accurate account of the spectrum shift. In all other cases, the band maximum was normally taken into account. Coefficients s, a and b were thus determined by multiple regression analysis of the absorption and emission data, for the three compounds. The results are gathered in Table 2. Satisfactory correlation coefficients were obtained in every case.
Linear correlations obtained by plotting versus π*, α and β according to the Taft and Kamlet equation (r = correlation coefficient)
Compound | Absorption | Emission | ||||||||
10–4 0 (cm–1) | 10–3s (cm–1) | 10–3a (cm–1) | 10–3b (cm–1) | r | 10–4 0 (cm–1) | 10–3s (cm–1) | 10−3a (cm−1) | 10–3b (cm–1) | r | |
1 | 2.45 | –0.68 | –0.34 | –0.24 | 0.98 | 2.34 | –1.73 | –0.58 | –0.71 | 0.96 |
2 | 2.39 | –1.30 | –0.45 | – | 0.97 | 2.22 | –2.36 | –1.34 | – | 0.90 |
3 | 2.46 | –1.31 | –0.43 | – | 0.94 | 2.36 | –2.80 | –1.05 | – | 0.89 |
According to this analysis, the absorption spectrum of the three dyes closely depends on solvent polarity/polarizability and acidity, which both induced a red shift. The spectrum of compound 1 was also shifted to the red with increasing solvent basicity. From a general point of view, the three compounds were more sensitive to variations of polarity/polarizability than to variations of the other parameters. Interestingly, very close results were obtained in absorption for dyes 2 and 3, while dye 1 was slightly less sensitive to changes of its environment.
The three compounds were more sensitive to the solvent nature in emission than in absorption. Compounds 2 and 3 now exhibited a slightly different behaviour, dye 3 being the most sensitive to solvent polarity, and dye 2 the most sensitive to solvent acidity.
3.4 Estimation of the excited-state dipole moments
In the first part of this work, we focused on the specific interactions that take place between the fluorophores and the solvent molecules. We shall now use a second approach, which assumes the fluorophore to be a point dipole held in the centre of a spherical cavity, in a homogeneous and continuous dielectric medium, and which considers the collective influence of the entire set of surrounding solvent molecules on the fluorophore.
It can be noted that the gap between the absorption and the fluorescence spectrum of our dyes increases in solvents of increasing polarity. Marked positive solvatochromism like the one encountered here generally indicates that the dipole moment of the molecule increases when passing from the ground state to the excited state. The enhancement of intramolecular charge transfer that occurs upon excitation induces reorientation of the solvent cage around the excited molecule. The change in dipole moment can be calculated by relating the Stokes shifts (abs – em) to the solvent orientational polarizability f(D,n), owing to Kawski's equation (Eq. (3)) that is currently used for ellipsoidal fluorophores [21]:
In this equation, h is Planck's constant, c is the speed of light and a0 is Onsager's cavity radius. The solvent orientational polarizability f(D,n) is calculated from Eq. (4):
(4) |
(5) |
One can thus calculate (μe – μg) from Eq. (6):
(6) |
The Stokes shifts were thus calculated for our three compounds in the various solvents, and are collected in Table 3.
Stokes shift (abs – em) and solvent orientational polarizability parameter f(D,n), calculated from D and n values given in references [23] (a), [24] and [25] (b), and [26] (c)
Stokes shift (cm–1) | ||||
Solvent | 1 | 2 | 3 | f(D,n) |
p-Xylene | 1414 | 1797 | 1248 | 0.007 a |
Butyl acetate | 2108 | 2680 | 2042 | 0.412 a |
Ethyl propionate | 2012 | 2563 | 2051 | 0.464 b |
1-Butanol | 2126 | 3042 | 2218 | 0.750 c |
2-Butanol | 2145 | 2896 | 2126 | 0.741 c |
2-Propanol | 2191 | 2976 | 2172 | 0.778 c |
1-Propanol | 2116 | 2990 | 2253 | 0.780 c |
Toluene | 1566 | 1826 | 1401 | 0.029 c |
Ethanol | 2217 | 3029 | 2398 | 0.813 c |
Dioxane | 1717 | 2356 | 1816 | 0.042 c |
Ethyl acetate | 2012 | 2509 | 2089 | 0.489 c |
Tetrahydrofuran | 1994 | 2614 | 2184 | 0.549 c |
Benzene | 1616 | 1872 | 1225 | 0.004 c |
Methanol | 2263 | 3042 | 2377 | 0.855 c |
Acetonitrile | 2418 | 2816 | 2532 | 0.859 c |
Pyridine | 2201 | 2463 | 2033 | 0.649 c |
Dimethylformamide | 2420 | 2807 | 2554 | 0.836 c |
Dimethylsulphoxide | 2443 | 2862 | 2422 | 0.840 c |
The ground state dipole moment μg of the three dyes was obtained by semi-empirical quantum chemical calculations, using the AM1 program, after the geometry of the molecules was fully optimised (Table 4). The dipole moment values range between 6.50 and 7.65 D, and are in agreement with those experimentally determined for other coumarins, as reported in the bibliography [27–29]. The Onsager cavity radius a0 was also calculated from the energy minimized structures obtained with the AM1 calculations. It is defined as the radius of a spherical cavity surrounding the molecule, but it is usually equated to the molecular van der Waals radius. The maximum distance where charge separation can occur across the molecule must be considered, rather than the actual molecular axis of the molecule [30]. For the three dyes, this distance lies between the average coordinates of the nitrogen atom of the diethylamino group on one side of the molecule, and the nitrogen atom of the cyano group on the other side. It was estimated to 9.0 Å. The cavity radius a0 was taken as half of this distance, that is 4.5 Å.
Ground state dipole moment μg obtained with AM1 calculation for dyes 1–3; Slope m issued from the correlation of Stokes shift with solvent orientational polarizability; Correlation coefficient r; Difference μe – μg and calculated excited state dipole moment μe
Compound | μg (D) | m (cm–1) | r | μe – μg (D) | μe (D) |
1 | 7.00 | 870 | 0.942 | 2.82 | 9.82 |
2 | 6.50 | 1320 | 0.948 | 3.47 | 9.97 |
3 | 7.65 | 1162 | 0.930 | 3.25 | 10.90 |
The Stokes shift values were plotted versus f(D,n) as shown in Fig. 3. Straight lines were obtained, one running roughly parallel to other. Interestingly, alcohols fit reasonably to the same correlations established for aprotic solvents, confirming that specific solute–solvent hydrogen bonding plays a minor role in the observed spectral shifts. It can also be noted that in dioxane, the Stokes shift value is quite high for compounds 2 and 3, and the corresponding point does not fit the line well. The anomalous behaviour of dioxane has long been recognized and attributed to the presence of three spatial conformations of widely different dipole moments [20]. The slope of the plots was then calculated, with satisfactory correlation coefficients (Table 4), and the change of dipole moment of the fluorophores upon excitation was finally obtained using Eq. (6).
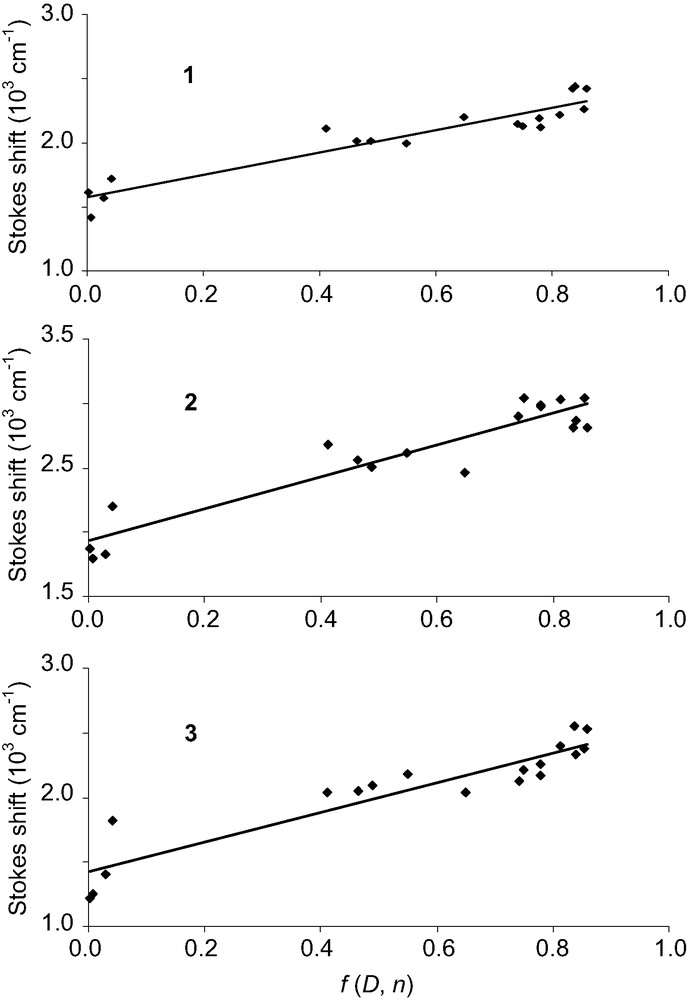
Plot of the Stokes shift (abs – em) versus solvent orientational polarizability parameter f(D, n) for dyes 1–3.
It appeared that the dipole moment of all three compounds under study was higher in the first excited state than in the ground state, as generally reported for coumarins studied by the same method [27,28,31–33]. The difference μe – μg was close to that determined for 7-amino-4-trifluoromethyl-coumarin (Coumarin 151) [33] and only slightly higher than that of 7-diethylamino-4-methyl-coumarin [27]. It is interesting to note here that the excited state dipole moment was higher for coumarin 3 than for the iminocoumarin derivatives 1 and 2, due to the presence of the strongly electronegative oxygen atom on the electron-withdrawing group.
3.5 Fluorescence quantum yields
The fluorescence quantum yield of compounds 1–3 was measured in four different organic solvents, in addition to that already measured in dichloromethane [16] (Table 5). Moreover, since the dyes are designed for use in biological medium, their behaviour in water was also investigated. Aqueous solutions were prepared as described in the experimental section. Among the three dyes, compound 1 seemed to be the most easily soluble in water. However, the absorption spectrum was wide at long wavelengths, and its shape varied with subsequent dilution, with a shift of the absorption maximum. It was noted that the excitation spectrum depended on emission wavelength, and conversely, the emission spectrum varied with excitation wavelength. This indicates a heterogeneous medium, probably made of a mixture of dissolved dye and microparticles, although solutions were carefully filtered. It can be noted that exactly the same results were obtained when dye 1 was dissolved in diethyl ether before being incorporated into water (see experimental part). Besides, compound 1 was progressively hydrolysed in water (pH = 6) as evidenced by thin layer chromatography. In these conditions, measuring the quantum yield was meaningless. No reliable determination of the absorption and emission maxima was possible either, and this is the reason why these data were not used above in the solvatochromic approach. Such difficulties were not encountered for dyes 2 (λabs = 452 nm and λem = 518 nm in water) and 3 (λabs = 435 nm and λem = 480 nm), whose quantum yields could be accurately measured.
Fluorescence quantum yield of compounds 1, 2 and 3 in different solvents. Excitation at the maximum absorption wavelength. The error on the quantum yield values is estimated to be 5%. a: From Ref. [16]
1 | 2 | 3 | |
Toluene | 0.48 | 0.85 | 0.87 |
Dichloromethane a | 0.84 | 0.85 | 0.82 |
Ethyl acetate | 0.78 | 0.79 | 0.59 |
Dimethylsulphoxide | 0.34 | 0.18 | 0.078 |
Ethanol | 0.33 | 0.17 | 0.034 |
Water | – | 0.031 | 0.012 |
It is obvious that the three dyes behaved differently, as long as the quantum yield was considered. Let us first consider non-protic solvents. In a low-polarity solvent, such as toluene, dye 1 exhibited a moderate quantum yield, which increased in solvents of medium polarity, and then decreased again in polar solvents, while remaining at an interesting value. In contrast, the quantum yield of dye 2 was high in solvents of low and moderate polarity, then decreased in polar solvents. Compound 3 followed the same trend, but its quantum yield decreased more abruptly with increasing solvent polarity. If considering now protic solvents, the fluorescence efficiency of 1 and 2 was moderately decreased in ethanol (with respect to DMSO) while that of 3 was significantly decreased. For instance, in ethanol, the quantum yield of 3 was only one fifth of that of 2, and one tenth of that of 1. It is possible that in this solvent, like in DMSO, water traces induced a slight decrease of the quantum yield. In water, the quantum yield of 2 and 3 was reduced again with respect to ethanol, and that of 2 was now quite low.
The quenching action of polar non-protic solvents on the luminescence of dipolar solutes has been frequently reported in the bibliography. Different mechanisms have been proposed to explain this phenomenon, and the following is possibly the most generally evoked. It is considered that since solvation by polar solvents lowers the energy of the polar excited state, it reduces the gap between the excited state and the ground state, and thus favours radiationless deactivation pathways [20]. This mechanism could explain why the fluorescence of the three dyes decreases in strongly polar solvents. It is possible that compound 3 is more sensitive to this mechanism than the two other compounds. The involvement of a twisted internal charge transfer process, leading to a non-fluorescent excited state, can also be considered, as reported for some 7-alkylamino-substituted coumarins [34]. Such a mechanism would be favoured by the presence of a strong electron-withdrawing group, which could explain the difference observed between iminocoumarins and coumarin.
The quenching action of protic solvents such as alcohols and water is generally explained by the formation of hydrogen bonds and protonation in the excited state. This process can be explained by the fact that the excited molecule is more polar than the ground state molecule, and is thus more able to interact with a proton of the solvent.
Protonated iminocoumarins are known to be fluorescent, but they are generally formed in acidic medium and give rise to a shift of the emission spectrum [10]. No abnormal behaviour was observed here on the emission spectrum, and processing the emission data with the polarity scales indicates that only weak specific effects occur in the excited state in organic solvents. Consequently, it seems that protonation of the excited state is not efficient for iminocoumarin derivatives in our experimental conditions.
In contrast, the formation of hydrogen bonds is very likely, since the analysis of the spectroscopic data with the Taft and Kamlet scale indicates that specific effects take place with protic solvents. This mechanism could take place in the three compounds. It is understandable that it occurs more easily in the coumarin series, where the functional group bears a strongly electronegative oxygen atom.
4 Conclusions
This study allowed us to have a deeper insight into the solvatochromic behaviour of our compounds. Kawski's approach showed that the variation of the dipole moment upon excitation was roughly comparable in the three compounds, which indicates that changing the nature of the electron-withdrawing group did not affect the general behaviour of the molecules upon excitation. The approach that uses the π* scale underlined strong similarities in the solvatochromic behaviour of compounds 2 and 3, while compound 1 appeared to be less sensitive to a change in the solvent nature. Finally, the study of the quantum yields revealed that the three dyes actually act differently from a photophysical viewpoint. It appeared that coumarin 3 can advantageously be replaced by iminocoumarin 2, especially when the polarity and proticity of the medium increases. Owing to the convenient synthesis of this type of compounds, many different molecules could be obtained for use as fluorescent probes for precise applications.
Acknowledgements
We are grateful to Mrs. Christiane Couturier-Vidal for performing AM1 calculations. We also would like to thank one of the referees for very valuable comments.