1 Introduction
The residual quality of paper-based historical documents and its quantitative estimation may be investigated by different procedures before a total loss of mechanical properties occurs. The predominant number of methodologies focuses on the estimation of residual properties of the basic structural component of paper: cellulose. The viscometric determination of average polymerization degree, copper number, discoloration and mechanical tests such as folding endurance, tensile strength and elongation at break represent the series of tests that are the most frequently used.
Recently, the chemiluminescence method has been explored for the measurement of residual oxidizability of the sample [1]. The experiments carried out under programmed increase of temperature and at a certain concentration of oxygen were used for extrapolation of experimental data to ambient conditions of paper preservation. The coincidence between rate constants of cellulose oxidation determined from non-isothermal runs and those from the decrease of the average polymerization degree [1] is a good pre-requisite that the chemiluminescence method is worth of further application in paper degradation studies. Carbonates of calcium and magnesium, which are commonly used for de-acidification of less stable acid papers, are usual paper stabilizers [2] of the first stabilization approach. In the present paper, we have therefore examined the effect of magnesium and calcium carbonates on chemiluminescence of cellulose pulp under conditions of increasing temperature when all potential stages of cellulose degradation may be encountered. At low temperatures, the effect of water present and pH on degradation is important, while at higher temperatures ‘dry’ decomposition of cellulose due to oxygen initiation occurs.
2 Experimental
The chemiluminescence measurements were carried out with Lumipol 3, luminometer produced at the Polymer Institute of the Slovak Academy of Sciences, Bratislava (www.lumipol.com). The instrument has very low dark counts at room temperature (2–4 counts/s). Experiments were performed in the overall flow of gas 3.6 l/h. The mixtures of oxygen and nitrogen were pre-set using Bronkhorst mixing units (Ruurlo, The Netherlands).
DSC measurements were carried out on a Mettler Toledo DSC 821 instrument in an atmosphere of oxygen at the rate of heating 10 °C/min.
Whatman (Wh) filter paper No. 1 (Maidstone, UK) considered to be pure cellulose, has been used in this study. The samples containing Ca(HCO3)2 and Mg(HCO3)2 were prepared by immersion of the sheet of original paper into solutions of pre-determined concentrations of Ca(HCO3)2 and Mg(HCO3)2 (0.91 mmol/l) or MMMC (2% in methanol), respectively, and dried at room temperature. The measured samples are listed in Table 1.
Characterization of samples
The sample No. | Additive | pHa | Note |
1 | Whatman, reference | ||
2 | Mg(HCO3)2 | 9.8 | Sample 1, 30 min immersed in neutralisation agent |
3 | Ca(HCO3)2 | 9.3 | Sample 1, 30 min immersed in neutralisation agent, 0.91 mmol/l |
4 | MMMCb | 10.1 | Sample 1, 30 min in neutralization agent, 2% wt. |
5 | HCl | 3.5 | Sample 1, acidified by 0.005 M HCl |
6 | HCl + Ca(HCO3)2 | 9.1 | Sample 5, 30 min immersed in neutralisation agent |
7 | HCl + Mg(HCO3)2 | 9.2 | Sample 5, 30 min immersed in neutralisation agent |
8 | HCl + Mg(HCO3)2 | 7.2 | Sample 5, acid sheets interleaved with sheets impregnated by neutralization agent, 3.5 atm, 3 days |
9 | HCl + Ca(HCO3)2 | 7.1 | Sample 5, acid sheets interleaved with sheets impregnated by neutralization agent, 3.5 atm, 3 days |
10 | HCl + MMMC | 7.0 | Sample 5, acid sheets interleaved with sheets impregnated by neutralization agent, 3.5 atm, 3 days |
11 | 4.4 | Original paper made in the Slavošovce paper mill, Slovakia, medium fine-grained, acidic | |
12 | Original paper 11, aged 24 days at 105 °C |
a pH after paper drying at room temperature;
b methanol solution of methoxy magnesium methyl carbonate (MMMC).
3 Results and Discussion
Chemiluminescence–temperature runs for Whatman cellulose containing different additives are shown in Fig. 1. One can immediately notice that magnesium carbonate in cellulose having pH higher than 9 under room conditions gives the most intense chemiluminescence signals at elevated temperatures. The generally accepted relation between the chemiluminescence intensity I and the rate of oxidation w:
(1) |
(2) |
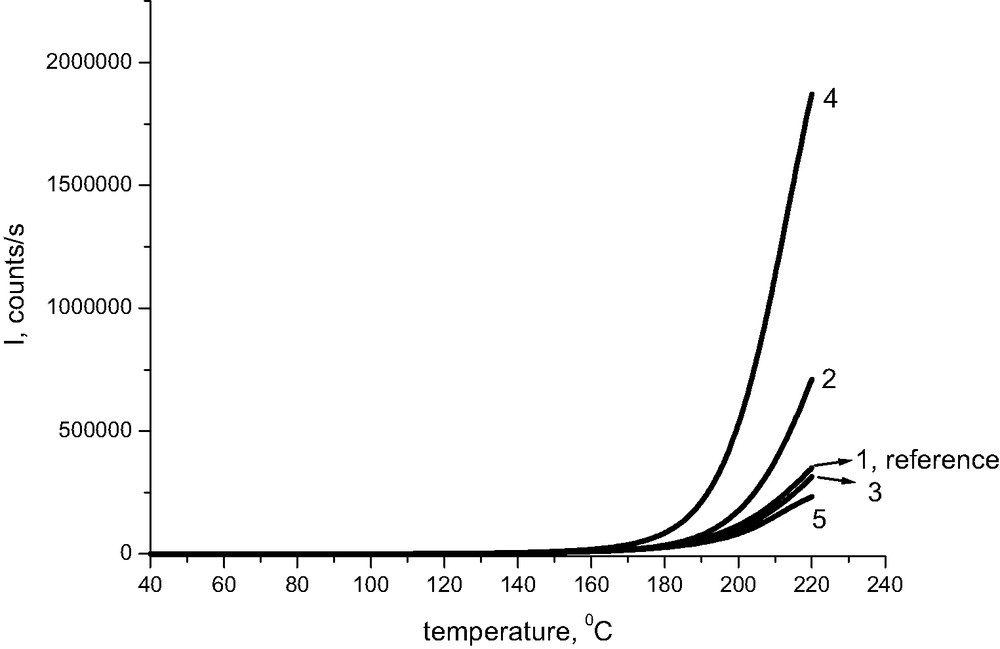
Chemiluminescence from cellulose pulp treated with some additives, oxygen, rate of heating 2.5 °C/min. The numbers correspond to the samples described in Table 1.
Dividing equation 1 by equation 2 we obtain:
(3) |
The unknown parameter may be thus eliminated and relative values of chemiluminescence intensity may be used for semiquantitative comparison of sample stability.
The above approximation is based on the assumption that the rates of oxidation of the same material (cellulose) are quite comparable at very high temperatures regardless of the presence of any additive. The transformation of experimental lines from Fig. 1 according to eq. (3) is seen in Fig. 2. Magnesium carbonate and MMMC (methoxy magnesium methyl carbonate) (lines 2 and 4) increase the stability of cellulose at low temperatures significantly. Less efficient seems to be calcium carbonate (line 3), while Whatman paper treated with HCl (line 5) degrades faster than reference sample of Whatman (line 1).

The data from Fig. 1 plotted in relative units of chemiluminescence intensity (Irel) and semilogarithmic coordinates. The numbers correspond to samples described in Table 1.
Fig. 3 shows that the presence of calcium has practically no effect on absolute values of chemiluminescence intensity; the respective lines are, however, slightly shifted to higher temperatures. The pronounced effect of magnesium ions on chemiluminescence intensity is limited exclusively to samples being initially at room temperature under alkaline conditions. When the pH of the original sample approaches 7, the chemiluminescence intensity at 220 °C becomes comparable to that of the reference sample of Whatman (Fig. 4), even for magnesium-containing samples.
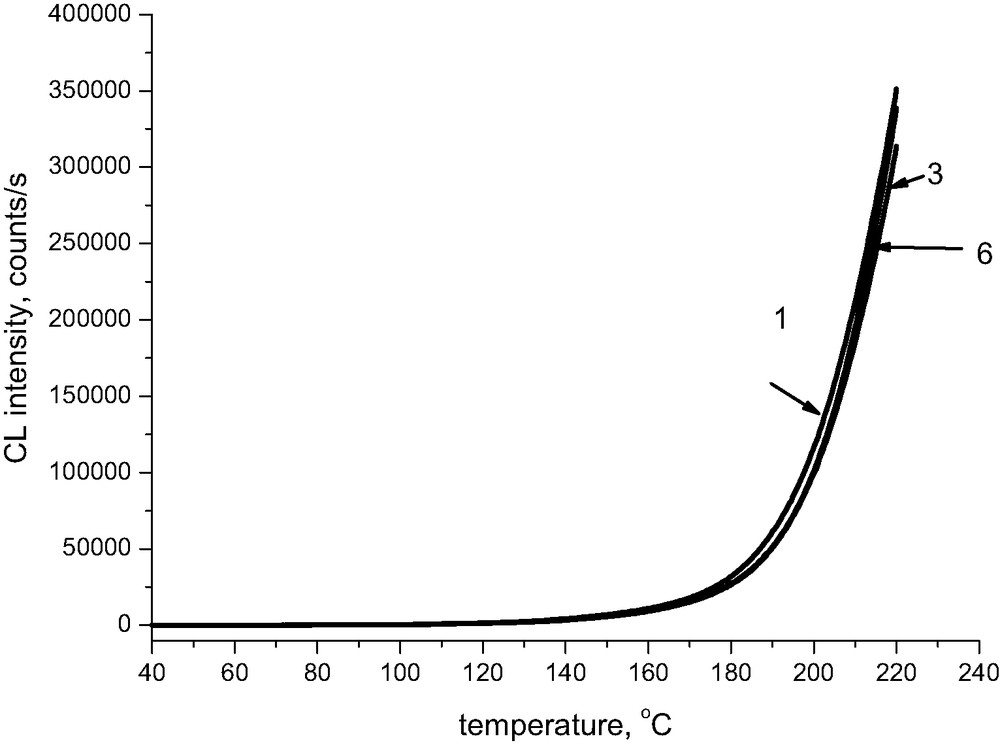
Chemiluminescence from cellulose pulp treated with calcium carbonate,oxygen, the rate of heating 2.5 °C/min. The numbers correspond to the Table 1.
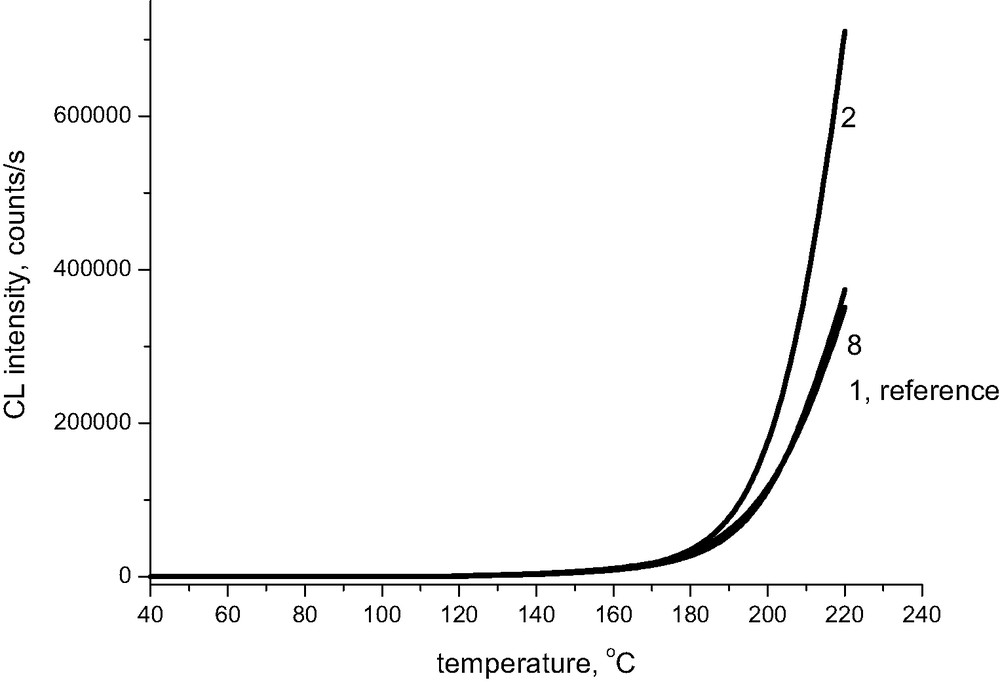
Chemiluminescence from cellulose pulp treated with magnesium carbonate in oxygen atmosphere, the rate of heating 2.5 °C/min. Line 2 corresponds to pH 9.8 and line 8 to pH 7.2 (See Table 1).
The interpretation of the above observation is based on the assumption of electron transfer interaction between glycosidic oxygen linking glucopyranosyl units of cellulose and gaseous oxygen (Scheme 1). This process may compete with the attack of protons on glycosidic bonds in acid medium, which leads subsequently to hydrolysis (Scheme 2). Interaction of oxygen with glycosidic bonds is much more probable in alkaline medium where protons are absent. At the same time, magnesium ions may promote the electron transfer between a potential electron donor and an electron acceptor [3,4]. However, what happens in samples when we approach the high-temperature region is not clear. Consistent with the pronounced chemiluminescence intensity, the effect of magnesium ions and initial alkaline conditions remains apparently preserved.
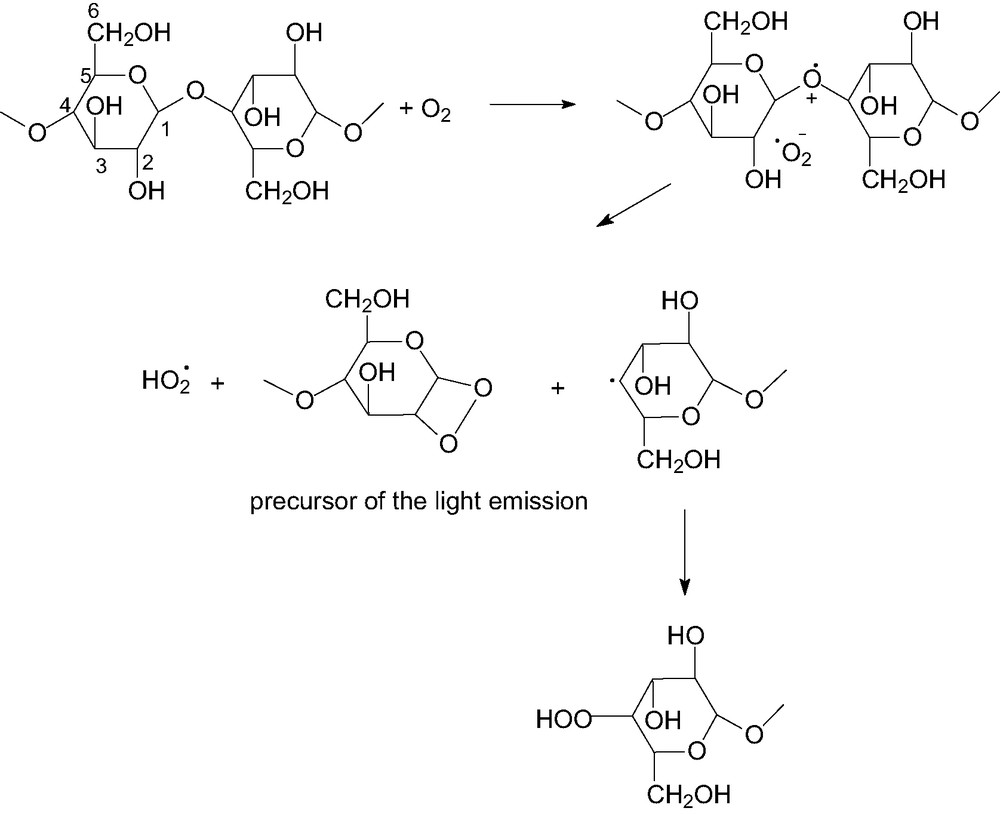
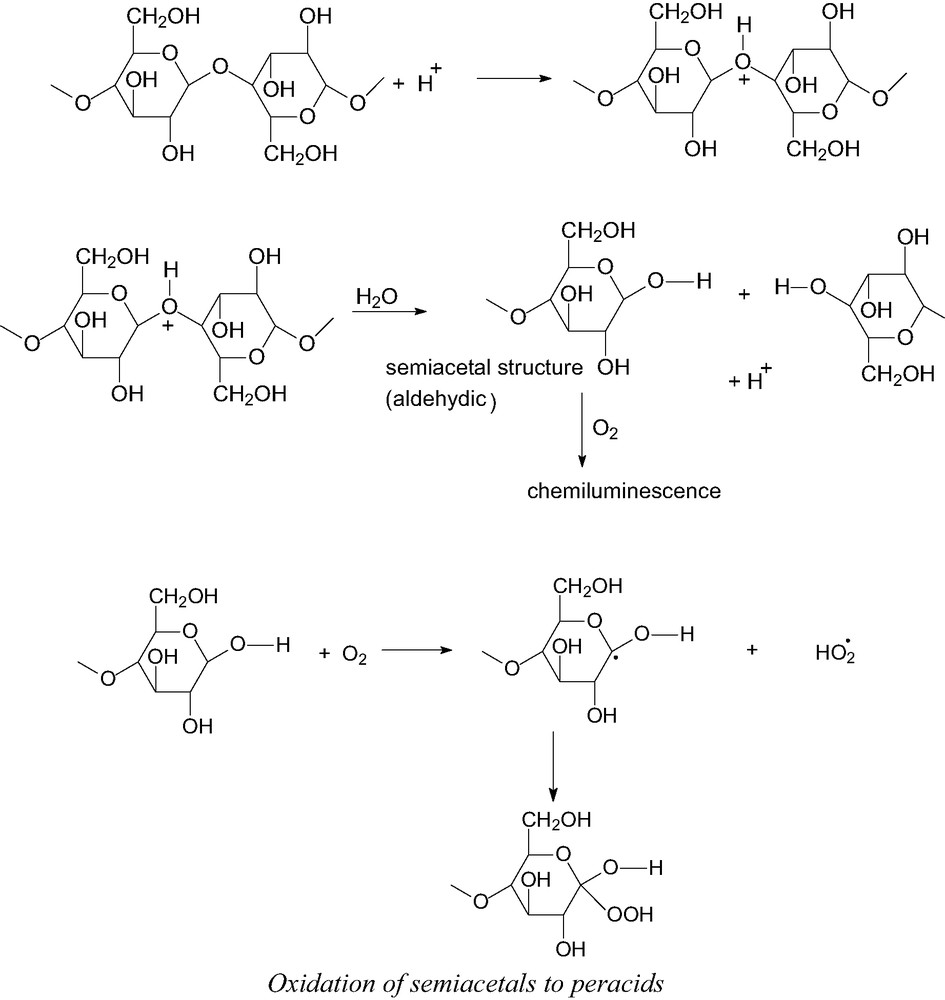
Oxidation of semiacetals to peracids.
Cation radical on glycosidic oxygen and superoxo anion radical are mutually attached; however, the hydroxyl group in position 2 is slightly acid so that the formation of dioxetanes, source of the light emission, may easily occur at higher temperatures. Dioxetanes are unstable and decompose into the excited triplet of aldehydic groups, which in turn yields intense light emission. In parallel, hydroperoxides are formed, which may provide additional chemiluminescence during their decomposition. In such a case, the elementary step responsible for the light emission may be the disproportionation reaction of hydroxyl and alkyloxyl radicals, giving water and excited triplet carbonyl groups. Decomposition of the above peroxidic intermediates may explain the formation of the whole spectrum of products from the oxidation of cellulose occurring at moderate temperature and in the presence of water.
Acid papers give a much more pronounced chemiluminescence signal at temperatures close to ambient when compared with reference cellulose sample (Fig. 5). This is in accord with the assumption that acid catalyzed scission of glycosidic 1,4 bonds in the presence of water in paper at lower temperatures gives terminal semiacetal (aldehydic) groups, which are subsequently oxidized and provide the light emission (see Scheme 2). Semiacetals, similarly to aldehydes, have reducing properties and react with oxygen to give carboxylic acids. The oxidation probably occurs via peracids or hydroperoxides and has a free-radical mechanism leading to chemiluminescence from recombination of peroxyl radicals. One can thus see that the rate of main chain scissions of cellulose and chemiluminescence due to its oxidation should thus be mutually related [1].
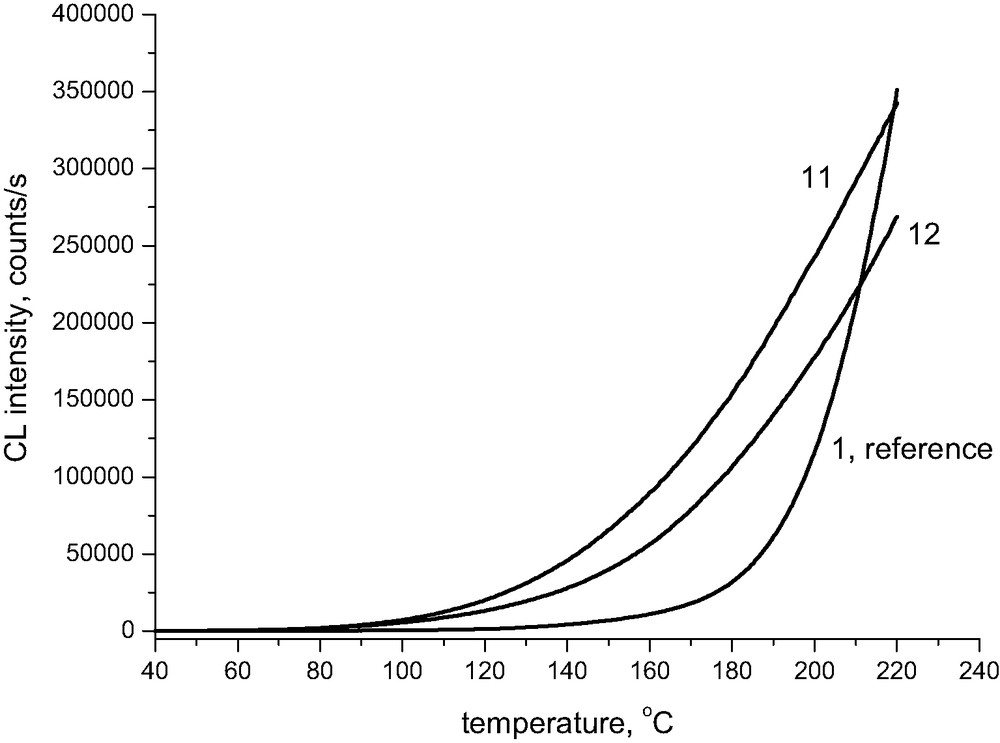
Chemiluminescence from cellulose pulp (line 1) and from acid paper (Slavošovce) before (line 11) and after 24 days of accelerated ageing at 105 °C (line 12), The chemiluminescence measurements were performed in oxygen atmosphere, the rate of heating 2.5 °C/min. The numbers correspond to Table 1.
Fig. 6 shows the comparison of degradation of cellulose-containing magnesium carbonate examined by chemiluminescence and by DSC. While the chemiluminescence method indicates the significantly lower stability at rather low temperatures of a sample having pH = 7.2 when compared to that having pH above 9, DSC confirms the difference existing at considerably higher temperatures when the sample is already charred. It is, however, of interest that the pattern of instability is maintained until high temperatures where it is revealed even by DSC.

Comparison of stability of cellulose pulp containing Mg(HCO3)2 having different pH at room temperature. The left-hand axis is related to non-isothermal chemiluminescence, the right-hand one to DSC runs. The numbers correspond to the samples described in Table 1.
The chemiluminescence signal from oxidized Whatman cellulose depends on the concentration of oxygen in the surrounding medium. From the study of other polymers, it is known that the main contribution to light emission probably comes from peroxyl radicals, which in their recombinations provide carbonyl groups in an excited triplet state. From a simple experiment with stepwise dilution of oxygen with nitrogen (Figs. 7, 8) we may see that the intensity of the light emission is proportional to the square root of oxygen concentration. This may be an indication that oxygen participates more in initiation than in propagation of oxidation reaction. This is the main difference when compared, e.g., with oxidation of polyolefins. At the same time, comparing the levels of chemiluminescence intensities and thus the rates of oxidation of Whatman cellulose for oxygen, air, and nitrogen, the following ratio of respective rate constants may be estimated (Scheme 3, Fig. 8).

Continuous changes of the intensity of the chemiluminescence signal with the concentration of oxygen in the surrounding atmosphere, 180 °C, cellulose pulp. Numbers are % vol. of oxygen in the mixture with nitrogen.

Plot of quasi-stationary levels of chemiluminescence intensity from Whatman cellulose at 180 °C vs. the square root of oxygen concentration in surrounding gas.

Replacement of oxygen by nitrogen leads to the expected reduction of the light intensity. However, when we replace nitrogen with oxygen, the signal jumps to a considerably higher value than that corresponding to the stationary level. The latter is subsequently reached through a certain decay (Fig. 9). The sharp increase of the chemiluminescence signal is proportional to the time during which the sample is placed under inert atmosphere. This observation has two alternative explanations. In an inert atmosphere, by direct scission of 1,4 glycosidic bonds in cellulose, there are accummulated aldehydes (semiacetals). When switching to oxygen, aldehydes are consumed in a fast oxidation until their stationary level is restored. George [5] explains the similar observation made during thermal treatment of nylon 6,6 as a transfer from a certain level of alkyl radicals to the same level of peroxyl radicals when oxygen is admitted, which have significantly higher recombination rate constants. This is then reflected in a decay from an initial higher intensity to a lower stationary intensity.
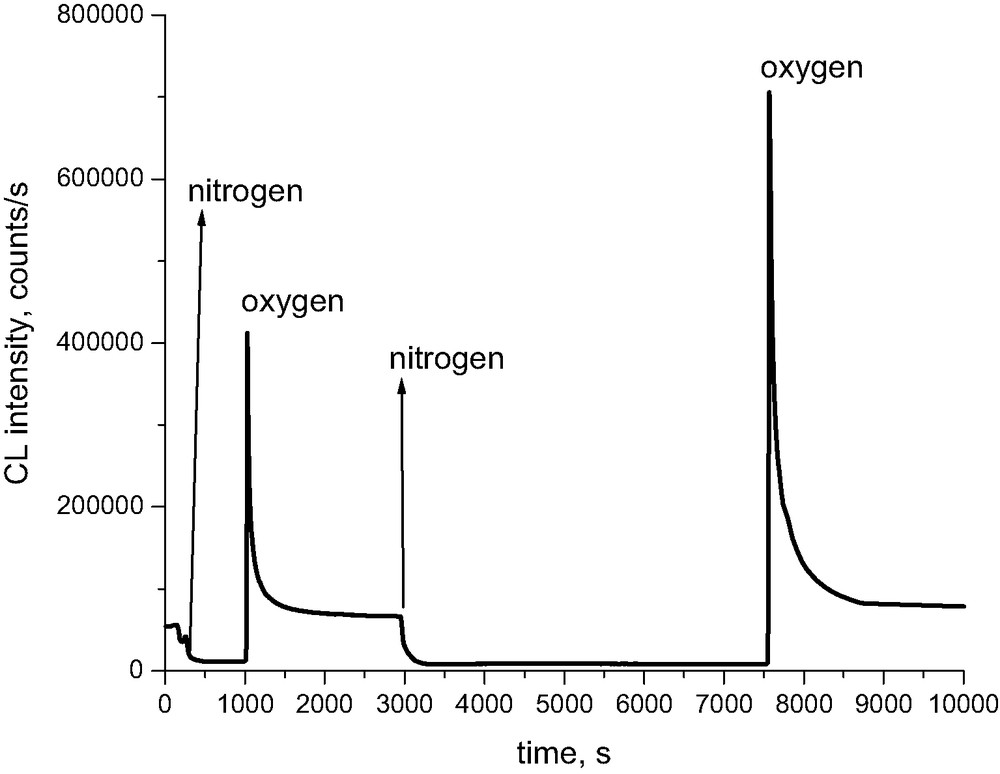
Relaxation experiments of abrupt changes of surrounding gas for Whatman cellulose containing Mg(HCO3)2 at 180 °C.
3.1 Kinetic analysis of chemiluminescence runs and the relation with polymerization degree changes from simple model of the degradation process
The relative comparison of oxidizability of different samples based on relative values of chemiluminescence intensity does not seem to be sufficient when we want to compare the results with the data from other methods. For such purpose some model should be used and implemented into the output of respective method.
The kinetic model of the cellulose degradation that has been the subject of numerous papers [6,7] is quite simple. The polymerisation degree (DP) is understood here as the ratio of concentration of monomer units (N) and polymer molecules (i) as follows:
(4) |
The concentration of cellulose molecules during degradation increases with time. Provided that the process of chain scissions is of zero order (), the concentration i of macromolecules changes with time as follows:
(5) |
(6) |
(7) |
(8) |
Equation (7) may be found in the literature as the Ekenstam equation [7]. The plots of vs time give a slope equal to . However, it is more practical to use the equation (8), which gives the rate constant of magnitude s−1 and enables a direct comparison between samples of different initial DP.
To develop some methodology of classification of data from chemiluminescence, we have to understand the reactions leading to the light emission. As it was already outlined by equation (1), chemiluminescence intensity generally should be related to the rate of sample oxidation. However, it depends on a set of parameters like geometry of the sample, temperature, oxygen concentration, the concentration of potential emitters, such as carbonyl groups in the sample, morphology and water content, etc. In the first approximation, we have, therefore, assumed that:
(9) |
According to eq. (8) and for non-isothermal conditions we have:
(10) |
Here T is the temperature, A and E are the pre-exponential factor and the activation energy, respectively and is the linear rate of sample heating.
After integration of eq. (10) and back substitution into eq. (9), we finally obtain for non-isothermal conditions:
(11) |
We may notice that while the process of chain scission is of zero order, the chemiluminescence runs formally correspond to the second-order scheme.
Provided that the process of light emission corresponds to several initiation events (i = 3), we have:
(12) |
Here Pi is the proportionality constant including the corresponding terms from the eq. (11), Ai and Ei are the pre-exponential factor and the activation energy of the respective components of the initiation event. An averaged value of the rate constant of paper degradation may be calculated from the rate constants of each component of the initiation event at a given temperature.
The averaged rate constant kav for three components of initiation (i = 3) leading to the light emission is defined as:
(13) |
Here k1/i0, k2/i0 and k3/i0 are rate constants at a given temperature determined from the corresponding Arrhenius parameters for each component of the initiating process, respectively. The first is related to a faster process, the second to a slower process of cellulose degradation, while the third was introduced formally to obtain a fit of eq. (12) to experimental runs as close as possible. This enables to approximate the rate constants at low temperatures, which is of interest for preservation of paper-based historical documents. While in paper [1] we have discussed the values of the corresponding activation energies and rates constants of the faster and slower process, here we focus on simple evaluation of an average half-life of respective sample at some temperature that is of interest for prognosis of the remaining service life of paper-based material.
From the averaged rate constant, the average half-life of samples from Table 1 may be determined for a given temperature as follows:
(14) |
The values of τ for temperatures from 20 to 105 °C are summarized in Table 2.
Half-lives of samples 1–12
Sample no | 20 °C | 20 °C | 60 °C | 90 °C | 105 °C |
Days | Years | Days | Days | Days | |
1 | 11 184 | 30.6 | 253 | 25a | 9 |
2 | 40 593 | 111.2 | 843 | 80 | 28 |
3 | 23 208 | 63.6 | 415 | 36 | 12 |
4 | 85 406 | 234.0 | 1 528 | 133 | 45 |
5 | 7 080 | 19.4 | 184 | 20 | 8 |
6 | 4 407 | 12.1 | 165 | 22 | 9 |
7 | 23 879 | 65.4 | 563 | 58 | 21 |
8 | 12 859 | 35.2 | 308 | 32 | 12 |
9 | 3 606 | 9.9 | 128 | 16 | 7 |
10 | 1 997 | 5.5 | 94 | 15 | 6 |
11 | 217 | 0.6 | 10 | 1.5 | 0.6 |
12 | 96 | 0.3 | 7 | 1.3 | 0.6 |
a From DP measurements of pure Whatman at 90 °C in air, the half-life estimated from the lower slope of the graph DPo/DP vs. time is 57 days.
We have to emphasize that the above numbers correspond to oxygen atmosphere. Looking at Fig. 2, we see that the relative comparison of the stabilities of samples 1–5 correspond well with the quantitative data in Table 2. The most stable are samples containing MMMC or magnesium carbonate (sample 2, 4, and 7), relatively efficient in stabilization is also calcium carbonate; however, when pH shifts to lower values, the stability significantly decreases (samples 8, 9 and 10). The less stable is the acid paper containing lignin (samples 11 and 12). The effect of pre-degradation on further lowering of paper stability becomes distinct at low temperatures. The verification of lower-temperature data from the above Table 2 is not possible at the moment, those at higher temperatures correspond quite well with, e.g., the rate constants determined from the decay of polymerization degree [1]. The comparison of rate constants of degradation from polymerization degree and from non-isothermal chemiluminescence experiments [1] is from this viewpoint very promising and the chemiluminescence method may be highly recommended for an easy estimation of the residual quality of paper samples.
4 Conclusions
Chemiluminescence accompanying the oxidation of Whatman cellulose as well as other papers corresponds well to the degradation and reduction of their molar mass. The method appears to be suitable for the fast estimation of the remaining service-life of paper-based materials.
Acknowledgements
The authors gratefully acknowledge the support of the European Community, 5th Framework Energy, Environment and Sustainable Development programme, contract no. EVK4-CT-2000-00038 (PAPYLUM). The work is the sole responsibility of the authors and does not represent the opinion of the Community. The Community is not responsible for any use that might be made of the data appearing herein.
A partial financial support was also provided by Grant Agency VEGA (projects No. 2/3053/23 and 2/5109/25 and by the project KNIHA-SK from the Ministry of Education of the Slovak Republic.