1 Introduction
The photocatalytic process is now well known and recognized as an efficient method to remove organic pollutants present in aqueous phase such as pesticides, dyes or in gaseous phase such as volatile organic compounds (VOC) [1]. Several industrial reactors are now commercialized for this type of application [2]. Main researches concerned water treatment or removal of pollutants, but disinfection was few studied. Photocatalytic oxidation as a technique for microbial disinfection was first demonstrated by Matsunaga et al. [3] using TiO2/Pt as a catalyst. Blake [4] and Fujishima [5] provide a review of the application of TiO2 photocatalysis to disinfection. Since 2000, Pulgarin's team thoroughly studied the effects of various parameters such as nature of support, nature of photocatalyst, light power and initial concentration on the photocatalytic efficiency [6–8]. In gas phase, the studies are very scarce. The pioneering work in the field of gas-phase photocatalytic disinfection was done by Goswami et al. [9,10]. He developed a recirculating duct facility to completely inactivate biological contaminants in indoor air. Beside studies on several bacterial strains [11,12], photocatalytic disinfection has also been carried out on microcystin toxins produced by various cyanobacteria (blue-green algae) [13,14]. In 2004, Keller et al. [15] developed and patented [16] a photocatalytic reactor removing more than 99% of bacteria. Very few studies performed tests to prove the efficiency of photocatalytic process to remove viruses. Following the attack of SARS (severe acute respiratory syndrome) virus, Knight [17] studied a system, based on photocatalytic disinfection, to control the spread of infectious microorganisms such as SARS virus on flights.
Herein, we report on fundamental studies on the efficiency of the photocatalytic process to inactivate bacteria. The originality of this work is to better understand the role of bacterial surface. We worked on two Escherichia coli strains, one having more fimbriae, and on two TiO2 photocatalysts (TiO2 Degussa P-25 and TiO2 Millennium PC500) and we used a dialysis membrane to determine the importance of the contact between the bacteria and the photocatalyst. The role of the generated hydrogen peroxide on bacteria inactivation will also be discussed.
Then, we will present the efficiency of the photocatalytic process to inactivate avian influenza virus A/H5N2, model of A/H5N1.
2 Results and discussion
2.1 Efficiency of the photocatalytic process for water disinfection
2.1.1 Comparative efficiencies of P-25 and PC500 TiO2 photocatalysts for the inactivation of two E. coli strains
This study was aimed at understanding if the modification of the bacteria surface by the increase of the number of fimbriae (E. coli PHL1273) could modify the photocatalytic degradability of E. coli. In E. coli PHL1273 strain, a gene coding for a green fluorescent protein has been incorporated in the genome in order to visualise the strain by fluorescence microscopy. This strain also carries the ompR234 mutation which increases the synthesis of curli, a particular type of fimbriae allowing the bacteria to better adhere to abiotic surfaces [18]. These curli or fimbriae should (i) improve the adsorption of bacteria on the photocatalyst and (ii) be directly in contact with the photocatalyst.
In a first approach, TiO2 Degussa P-25, used as a world reference, has been used to study the inactivation of both E. coli strains. Fig. 1 shows the changes in the survival of E. coli (PHL849 and PHL1273) bacteria concentration using TiO2 Degussa P-25. From this result, we can see that the inactivation of both bacterial strains was similar. In our experimental conditions, it was impossible to determine whether the adhesion of E. coli 1273 on TiO2 was increased or not. With respect to the obtained results, the inactivation of both E. coli strains on TiO2 P-25 seems to be similar. However, it is difficult to imagine that the presence of more fimbriae on bacteria does not modify the inactivation. The active species generated at the surface of titania during the irradiation should be more in contact with fimbriae than with the membrane. Thus, the fimbriae should be oxidized in competition with the membrane. This hypothesis is in agreement with the measurement of ammonium cations in solution. Ammonium ions, obtained from the degradation of aminoacids present in fimbriae, were detected only in the case of E. coli PHL1273. In conclusion, the similarity of the two curves suggests that the oxidation of fimbriae does not lead to the inactivation of bacteria during this irradiation time.
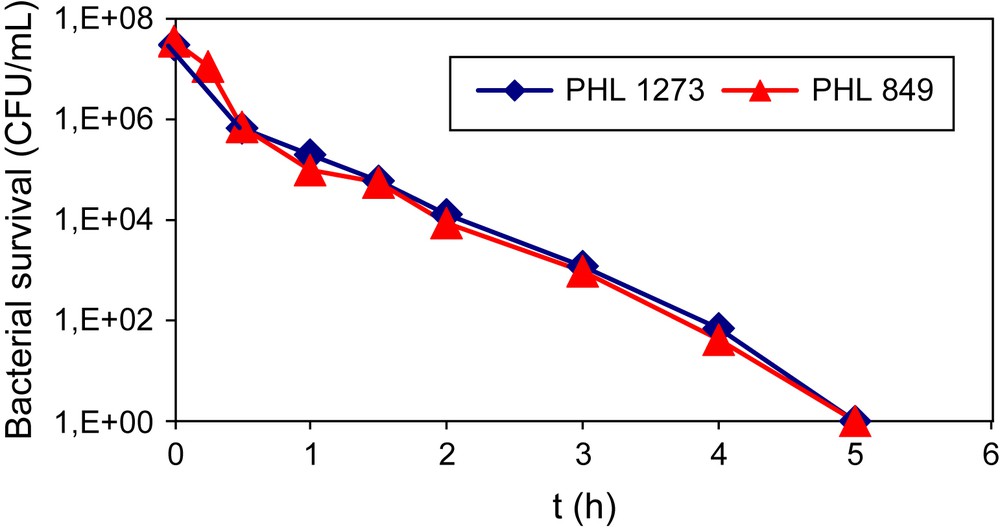
Inactivation of PHL849 and PHL1273 E. coli strains in the presence of TiO2 Degussa P-25.
The second experiment has been performed using TiO2 Millennium PC500 having a higher surface area, a smaller particle size and an isoelectric point (IEP) lower than that of TiO2 P-25 [8]. As seen in Fig. 2, PHL1273 strain, having more fimbriae, was more quickly inactivated than PHL849 strain in the presence of PC500. To explain this result, it can be suggested that the adhesion of PHL1273 on PC500 could be stronger. But how to explain this higher adsorption while no difference is observed with titania P-25? It may be due to the more negative overall charge of PHL1273 compared to that of PHL849 strains. Moreover, if the adhesion of PHL1273 strain on PC500 was really more important than that of PHL849 on the same photocatalyst, it should be considered either that the degradation products (aldehydes, acids, ions…) originating from the degradation of fimbriae participate in the inactivation of the bacteria or that the smaller particles of PC500 penetrate more easily in the membrane of PHL1273. Future studies are programmed to better understand this phenomenon.
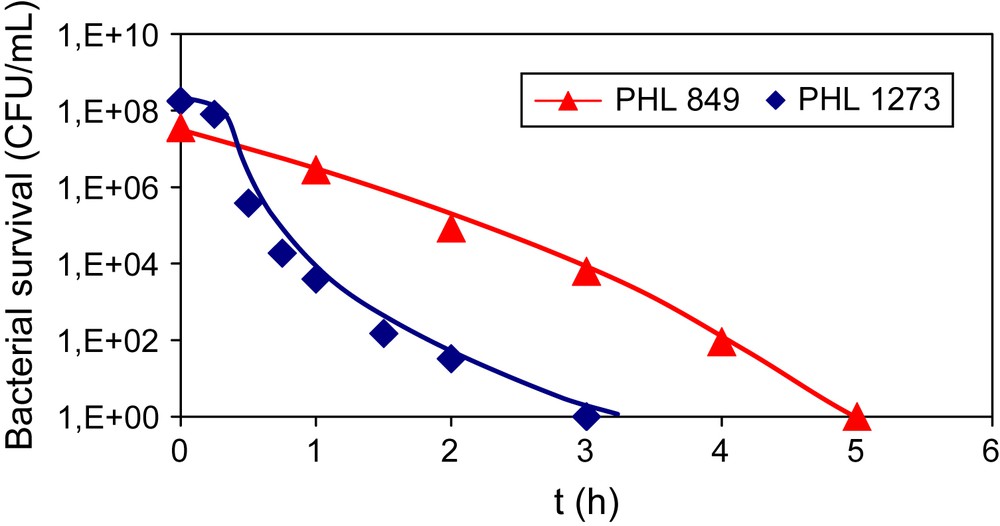
Inactivation of PHL849 and PHL1273 E. coli strains in the presence of Degussa TiO2 P-25.
2.1.2 Importance of the interaction between the bacteria and the semiconductor (TiO2)
This experiment has been performed using TiO2 Degussa P-25. In photocatalytic process, electron (e−)/hole (h+) pairs are generated during the irradiation. These species react, respectively, with O2 and H2O in aqueous phase to form superoxide anion radical (O2−) and hydroxyl radical (OH) as described in Eqs. (1) and (2):
O2 + e− → O2− | (1) |
H2O + h+ → OH + H+ | (2) |
Other secondary reactions can also occur, such as the formation of hydrogen peroxide, considering the reaction between superoxide radical and proton (Eqs. (3)–(5)).
O2− + H+ → HO2 | (3) |
HO2 + O2− → OH + O2 + H2O2 | (4) |
2HO2 → O2 + H2O2 | (5) |
In the case of photocatalytic degradation of organic molecule, it is admitted that active species are mainly OH radicals and, to a lesser extent, HO2 or O2−. H2O2 is not considered as an active species. Moreover, it is admitted that the reaction of this species occurs on the surface of the photocatalyst or in the first layer around the photocatalyst.
Because of the difference between microorganisms and organic compounds and due to the work of some authors on the inactivation of microorganism in the presence of H2O2, the goal of our study is to verify the importance of the interaction between the microorganism and the semiconductor, using a dialysis membrane. The experiments were performed by introducing a suspension of E. coli in a small volume (4 mL), whose envelope was constituted by the dialysis membrane, TiO2 Degussa P-25 photocatalyst being maintained outside the membrane. The measure of E. coli after 5 h of irradiation shows that no inactivation of bacteria was detected (Table 1). The difference observed is negligible. It means that:
- - H2O2 which could be generated during the photocatalysis process is not at the origin of the inactivation observed in the presence of photocatalyst and UV-A light;
- - a contact or the presence of bacteria very close to the irradiated semiconductor is required.
Concentration of E. coli present inside the dialysis membrane before and after 5 h of irradiation of TiO2 Degussa P-25 present outside the membrane
[E. coli] before irradiation | [E. coli] after 5 h of irradiation |
6.2 × 107 CFU/mL | 5.6 × 107 CFU/mL |
2.2 Efficiency of photocatalysis to remove avian influenza virus (A/H5N2) in air
In the second part of the study, the efficiency of the photocatalytic process in air disinfection was studied directly in a pilot reactor manufactured by Buxair, using an important virus aerosol flow, 40 m3/h.
In a preliminary study, we measured the efficiency of photocatalysis against the avian influenza virus. Since the A/H5N1 virus is strongly pathogenic, the tests were performed on the A/H5N2 strain chosen as the conventional research model for the A/H5N1 strain. A reference was made using no irradiation flux. The experimental set is represented in Fig. 6 (see Section 3).
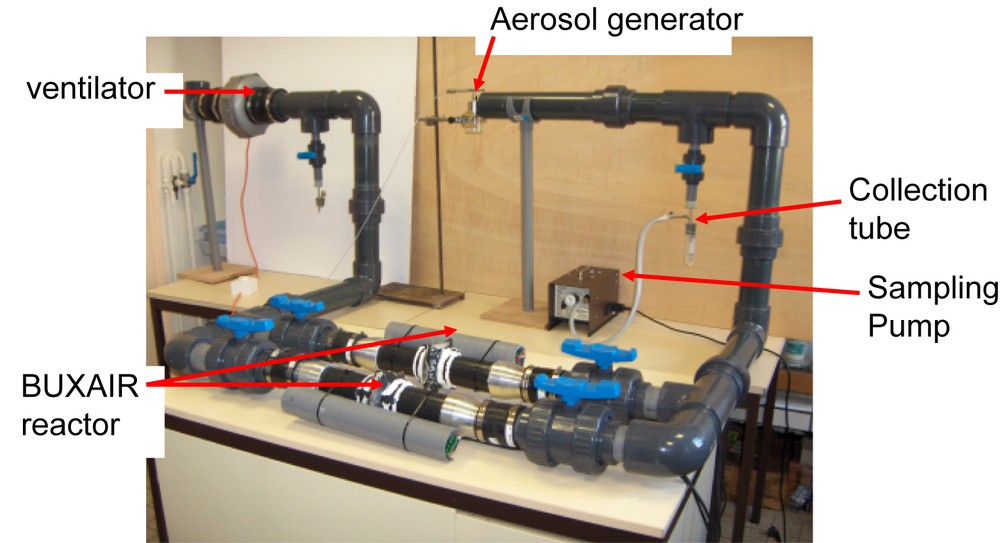
Photograph of experimental device used to test the efficiency of Buxair reactor against the avian influenza virus.
In the first experiment the photoreactor was used in the dark (with the UV-lamp turned off). After 30 min of stabilisation, the first sample was taken at the reactor inlet. The virus flow was sampled for 3 min using a sampling pump. The sampling valve at the reactor inlet was then closed and a sample was taken at the reactor outlet under the same conditions. Eight samples were taken alternatively at the inlet and the outlet and this operation has been repeated four times to check the reproducibility.
In the second experiment, the lamp was turned on. After 30 min of stabilisation, eight samples were taken under the same conditions, as previously described at the inlet and outlet.
Titration results are presented in Fig. 3a and b. Without UV-light, we observe a 0.7 log (or 80%) reduction of the number of viruses. The addition of UV-light led to a complete elimination of viruses titrated at entry up to a 3.1 log.
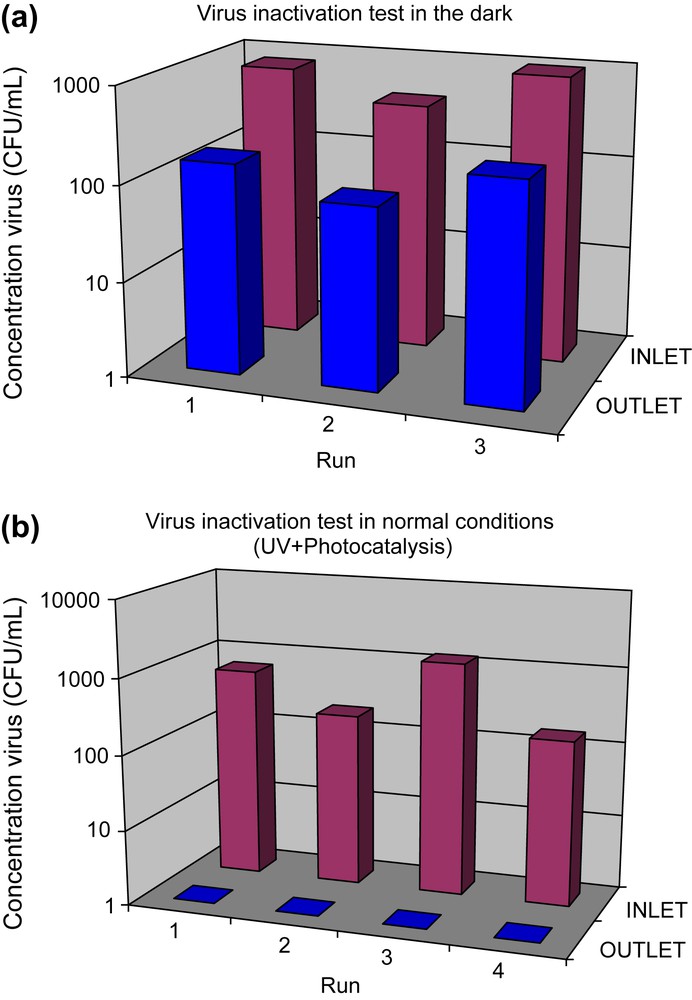
(a) Virus inactivation tested in the Imutube photoreactor in dark conditions. Air flow: 40 m3/h. (b) Virus inactivation tested in the Imutube photoreactor in UV in the presence of photocatalyst.
The test shows that Buxair technology is able to completely eliminate A/H5N2 viruses present in an aerosol titrated at a maximum of 103.2 UFP/mL in normal operating conditions (40 m3/h) and in a single pass.
The study performed on the A/H5N2 strain could serve as a research model for the A/H5N1 strain and shows that avian viruses can be destroyed by Buxair technology. More experiments should now be done to better understand the importance of the type of irradiation used.
2.3 Photocatalytic disinfection versus photocatalytic removal of organic pollutants: are the problematic issues similar?
Several important differences can be underlined, such as size, composition, viabilities and inactivation process.
The size of organic molecules, such as pesticides, volatile organic compounds, and dyes is below 1 nm, while the size of a microorganism is generally higher than 300 nm. For example, taking into account the epifluorescence microscopy measurement, E. coli, often used as model in disinfection, can be considered as a cylinder of 1.5-μm length and of 0.9-μm diameter [19].
The Langmuir adsorption observed in the case of organic pollutants on TiO2 cannot be considered for microorganism owing to its important size. E. coli has a size more than 30 times higher than that of TiO2, so it is the semiconductor which wrapped the microorganism and not the opposite.
The composition of a microorganism is very complex. Fig. 4 represents a Gram(−) bacteria. It is composed of different membranes or layers constituted of different types of organic molecules to protect the cell. For example, E. coli possesses an outer membrane beyond the peptidoglycan layer on which appreciatively 3.5 million molecules of lipopolysaccharide (LPS) are present, which covered about three-fourths of the outer membrane. The thickness of each membrane or layer is also important. Table 2 gives for example the thickness of each membrane [20].
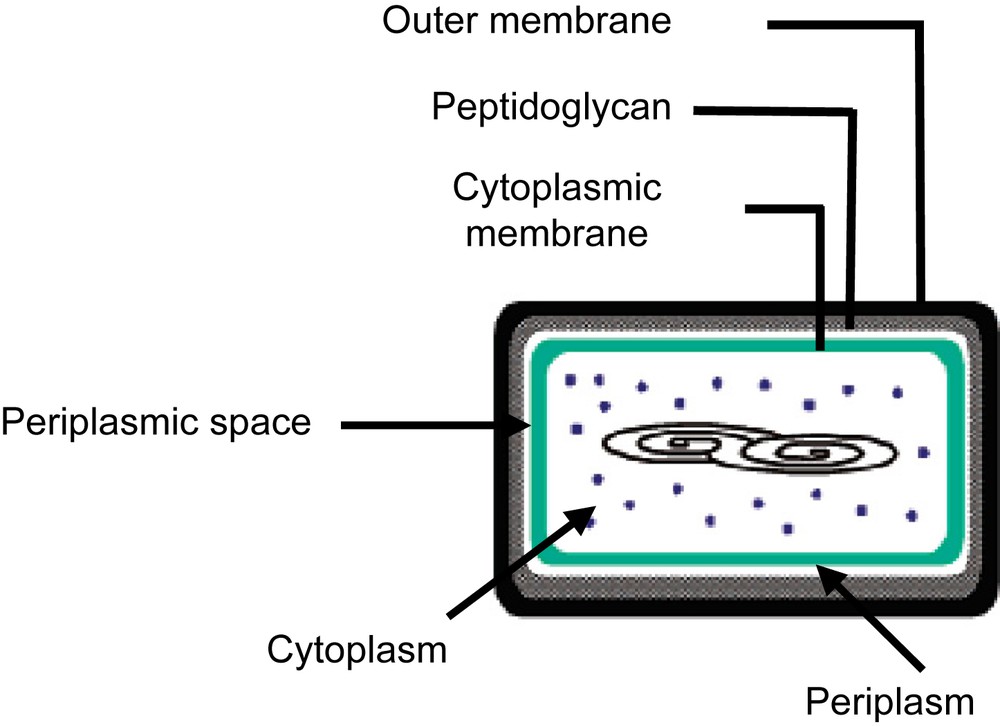
Representation of a Gram(−) bacteria.
Thickness of membrane in Gram(−) bacteria
Outer membrane | Peptidoglycan | Periplasmic space | Cytoplasmic membrane |
75 Å | 25 Å | 75 Å | 75 Å |
Another important difference between organic molecules and microorganisms is the viability of microorganisms. For example, bacteria possess protection and recovery mechanisms to overcome the oxidative stress that involve endogenous proteins such as antioxidant enzymes or DNA repair enzymes. We can underline the presence of enzymes such as Fe-SOD (SOD: superoxide dismutase), Mn-SOD and catalase which protect the cell from oxidative damages by catalysing reactions that prevent the accumulation of O2− and H2O2, or exonuclease and DNA-glycoxylase enzymes which are able to repair DNA lesions resulting from oxidative damages. Moreover, depending on its physiological state, their inactivation will be modified. In other words, when bacteria are in their growing phase, they are easily inactivated. This behaviour can be compared to the resistance of a baby or an adult to the disease.
Another important point to be considered is the way of determining the efficiency of the photocatalytic process. In the case of organic compounds, we follow mainly the disappearance of organic molecules and sometimes the mineralization. Only one modification in the structure of organic molecule leads to its disappearance, while the inactivation of a microorganism does not correspond to only one modification of an organic molecule present in the microorganism. The mechanism of inactivation is not very well understood until now. Matsunaga et al. [3] suggested that its inactivation is due to the oxidation of intracellular co-enzyme A (CoA) which inhibits the cell respiration. Other authors proposed that a significant disorder in the cell permeability (loss of ionic flow control) and decomposition of the cell walls are at the origin of their inactivation [21]. Many questions still remain misunderstood: is cellular death due to a loss of the integrity of the bacterial wall or to the oxidation of intracellular components? [22]
3 Experimental
3.1 Photoreactor and light sources for water disinfection
The reactor (Fig. 5) consisted of a batch of Pyrex cylindrical flasks (100 cm3) inside which 20 mL of E. coli aqueous solution was put into contact with the photocatalyst. The irradiated side of the reactor was the bottom window suprasil disk of 3-cm diameter. The light source was an HPK 125 W Philips mercury lamp, cooled with a water circulation. The irradiation spectrum was cut off below 340 nm using a Corning 0.52 filter. All reactions were carried out at ambient temperature.
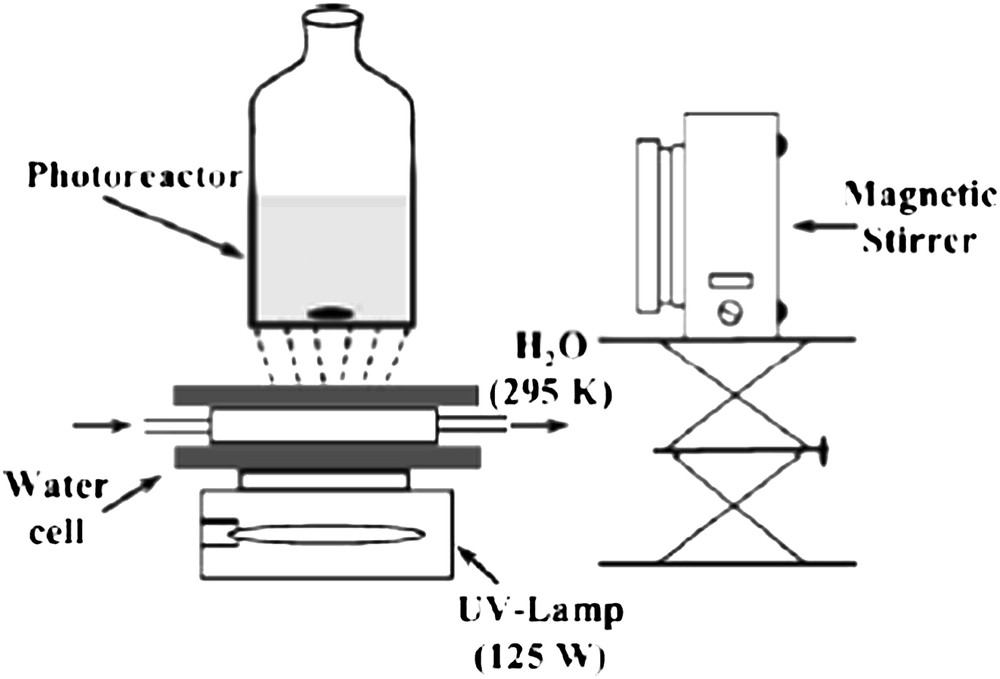
Scheme of the photoreactor used for water disinfection.
3.2 Photocatalysts
The experiments with suspended TiO2 were performed with commercial Degussa P-25 (anatase/rutile = 80/20, SBET = 50 m2 g−1, particle size = 30 nm) and industrial Millennium PC500 from Millennium Inorganic Chemicals (anatase, SBET higher than 300 m2 g−1, particle size ≈ 5 nm).
3.3 Bacterial strain and growth media
The bacterial strains used were E. coli K12 PHL849 and E. coli K12 PHL1273. The bacterial strain E. coli K12 PHL1273 was modified by incorporating a green fluorescent protein (gfp) in DNA and enhancing the expression of the csgA gene encoding curli synthesis (fimbriae for cell adhesion).
Aliquots of the overnight cultures were inoculated into fresh medium and incubated aerobically at 37 °C until the stationary growth phase was reached (15 h). Growth was monitored by optical density (OD) at 600 nm. Bacterial cells were harvested by centrifugation at 500g for 10 min at 4 °C. The bacterial pellet was subsequently washed three times with the Mili-Q water. Cell suspensions were inoculated in the solution in a Pyrex cylindrical reactor to the required cell density corresponding to 107–108 colony forming units per millilitre (CFU/mL). Serial dilutions were prepared if necessary in the solution and 100 μL samples were plated on Plate-Count-Agar (PCA, Merck, Germany). Plates were incubated at 37 °C for 24 h before the bacterial counting was carried out. The detection limit for the procedure employed was 30 CFU/mL.
3.4 Analytical determinations
The formation of ammonium ions was followed using ionic chromatography with a Dionex DX-120 pump and conductivity detector, and a CS 12A (250 mm × 4 mm) column. The flow rate was 1 mL/min, the injection volume was 50 μL and the mobile phase was H2SO4 (22 mmol/L).
3.5 Photoreactor for air disinfection
We used a photocatalytic Buxair reactor using an aerosol flow of about 40 m3/h and 103 CFU/mL. Imutube® manufactured by the Buxair Group consists of a UV-C source and an Emavab® titania photocatalyst (Fig. 6).
4 Conclusion
This work clearly pointed out the important difference between the photocatalytic removal of organic molecules. One has to take into account the size of the microorganisms compared to those of semiconductor and of organic molecules, composition and its viability. The efficiencies of TiO2 Degussa P-25 on the inactivation of K12 PHL849 and K12 PHL1273 E. coli strains were comparable, whereas a more important inactivation of PHL1273 E. coli was obtained on TiO2 Millennium PC500. This effect was the consequence of a better adhesion between the bacteria and the photocatalyst. An experimental study using a dialysis membrane allowed us to conclude that the microorganisms and the photocatalyst surface have to be in contact to observe any positive effect. A preliminary experiment on the photocatalytic disinfection of air contaminated by avian influenza virus A/H5N2 outlines the efficiency of the photocatalytic process for disinfection.
These new results still under development are promising for a new advanced disinfection process.