1 Introduction
Benzene is a notorious pollutant emitted from various urban and industrial activities, and has high toxicity and carcinogenic properties. One recent report showed that benzene, even at a low level of 1 ppm, can reduce blood cell counts and cause hematotoxicity in factory workers [1]. Therefore, efficient treatment to benzene from environment is mostly desirable, but it is a difficult challenge.
Several advanced oxidation technologies have been studied for volatile organic compounds' (VOCs) removal, among which the photocatalytic oxidation over TiO2 appears to be mostly attractive [2–8]. Many VOCs, such as formaldehyde, acetone, benzene and toluene, can be efficiently oxidized and mineralized into CO2 and H2O over TiO2 when the catalyst is excited by UV (ultraviolet) light irradiation. However, the catalyst is often rapidly deactivated, especially during aromatics oxidation, due to accumulation of less-reactive by-products on the photocatalyst surface [6]. Addition of a small quantity of water vapor to the system could slightly meliorate the catalyst's durability [7,8], but such a low inherent conversion is not significant for application. Loading of noble metal (Pt, Pd, and Rh) on TiO2, on the other hand, can enhance photooxidation efficiency of benzene in humidified air stream [2,9,10]. We have recently also demonstrated that introduction of trace amounts of H2 [11] into the reaction system could greatly increase benzene photooxidation efficiency and stability of Pt/TiO2. However, this method is unpractical for environmental application due to its high danger and cost. All the photocatalysts developed so far for benzene removal are mainly the TiO2-based ones. It may be a good strategy to extend the research using other classes of photocatalysts for improvement of the system efficiency.
In this work, we report a novel photocatalyst, In(OH)3, which is highly photoactive and stable for gas-phase removal of benzene under UV irradiation, even in dry O2 atmosphere. Both the conversion and mineralization of benzene are notably accelerated, as compared to TiO2 of Degussa P25.
2 Experimental section
2.1 Preparation of In(OH)3
In(OH)3 was prepared by hydrolysis of In(NO3)3 (0.05 M) in an aqueous solution of ammonium (12%) at room temperature. When the solution pH reached about 8, the white precipitate was collected, and washed thoroughly with distilled water till the total concentration of ionic species was lower than 10 ppm. The resulting slurry was then dispersed into water under ultrasound radiation until a transparent sol was formed. Finally, the sol was dried at 60 °C, followed by thermal treatment at 120 °C for 16 h.
2.2 Activity test
The photocatalytic oxidation experiment of benzene was conducted in a tubular quartz microreactor operating in a continuous-flow mode. The reactor was surrounded by four 8-W fluorescent UV bulbs (TUV 8W/G4 T5, Philips, wavelength 254 nm). The catalyst loading was 0.5 g (50–70 mesh). Unless otherwise mentioned, the reaction temperature was ca. 120 °C, which results from the light source without controlling. Certain amount of saturated benzene vapor (at 273.15 K) was introduced into the reactor along with a continuous O2 gas stream. Prior to irradiation, adsorption of benzene on the photocatalyst reached equilibrium. The concentrations of benzene and product CO2 were analyzed by an on-line gas chromatograph (HP 6890), equipped with a thermal conductivity detector, a flame ionization detector, and a Porapak R column.
2.3 Characterization
X-ray diffraction patterns (XRD) were collected using a Bruker D8 Advance X-ray diffractometer (Cu Kα, 1.5418 Å). UV–vis diffuse reflectance spectra (DRS) were recorded on a UV–vis–NIR spectrometer (Varian Cary 500 Scan, BaSO4 as a background reference). Infrared spectra (IR) were recorded on a Nicolet 670 FTIR spectrophotometer. TEM image of the sample was recorded on a JEOL model JEM 2010 EX instrument at an acceleration voltage of 200 kV. Nitrogen adsorption and desorption isotherms of the samples were measured on a Coulter Omnisorp 100 CX system. X-ray photoelectron spectrum (XPS) analysis was performed with a Quantum 2000 spectrometer (American physical electronic Co.) using Al Kα X-ray beam (1486.6 eV). All the samples were degassed in vacuum until the pressure is lower than 10−8 Torr. Binding energies were calibrated for charging effect in reference to the C1s peak, which was assumed to have a binding energy of 284.8 eV.
3 Results and discussion
3.1 Photocatalytic properties of In(OH)3
Fig. 1 shows photodegradation of benzene over In(OH)3 and P25 TiO2 under 254 nm UV light irradiation. For P25 TiO2, the initial conversion of benzene is 9.5%, and the amount of product CO2 is 180 ppm, corresponding to 26% of mineralization of benzene. However, during benzene photooxidation, it suffers from rapid deactivation so that the conversion of benzene decreases from 9.5% to 0% after 300 min, and the color of the catalyst changes from white to brown. The quick deactivation is related to the large amount of the carbonaceous deposits, as discussed later. The deactivated TiO2 cannot be regenerated even it is calcined under oxygen atmosphere at 200 °C for 5 h. In contrast to P25 TiO2, In(OH)3 presents a much higher steady-state photodegradation activity (from 200 to 800 min) for benzene under the same conditions. After 300 min of the photoreaction, the conversion of benzene and the mineralization rate over In(OH)3 achieve the maximum, 33.4% and 56%, respectively, while the P25 TiO2 is deactivated completely. For In(OH)3, the gradual increasing conversion rate of benzene in the range 0–300 min may be resulting from the increasing temperature of the reaction system under UV light irradiation. After 800 min of reaction, In(OH)3 also suffers from a gradual deactivation, but after 30 h of reaction it remains 25% of conversion and 40% of mineralization rate of benzene. These results show that In(OH)3 has not only higher photocatalytic activity and stronger photocatalytic mineralization power for benzene, but also better durability of photoactivity.

Photocatalytic oxidation conversion (A) and production of CO2 (B) on the catalyst (a) In(OH)3, (b) TiO2, respectively, in dry O2; curve (c) was a control experiment with In(OH)3 without irradiation, and (d) was a control experiment without In(OH)3 under 254-nm irradiation. Benzene: 1300 ± 40 ppm; flow rate: 20 cm3 min−1; catalyst: 0.50 g; temperature: 120 °C.
Two control experiments, either without the In(OH)3 photocatalyst or without UV light, were performed to prove the need for photocatalyst and UV light during the photodegradation of benzene. As shown in Fig. 1(c) and (d), negligible photoactivity for benzene is presented, showing that benzene cannot be photodecomposed by UV light, or by the photocatalyst alone. It was concluded that the concurrence of photocatalyst and UV light is necessary for the photodegradation of benzene.
Activity stability of the In(OH)3 can be further ameliorated by adding water vapor to the reactant gas. As shown in Fig. 2(B), the benzene photooxidation reaction reaches a steady state after 6 h in the presence of water vapor, and no notable deactivation is observed during the photoreaction.

Photocatalytic oxidation of benzene and CO2 formation on In(OH)3 with (B) or without (A) water vapor in the same concentration of benzene. Benzene: 1800 ± 50 ppm; flow rate: 20 cm3 min−1; catalyst: 0.50 g.
3.2 Spectral characterizations of the catalyst before and after benzene photooxidation
As shown in Fig. 3, as-synthesized In(OH)3 is a white solid with cubic crystallites. Some colorful by-products are formed from benzene oxidation on the catalyst, as evidenced by UV–vis DRS (Fig. 4). The reflectance percentage is decreased for both the catalysts after photoreaction, which is due to the deposits of by-products. Based on the magnitude of such decreased reflectance, the relative amount of the by-products on TiO2 is more than that on In(OH)3 after the reaction. It is also evidenced by the FTIR spectra in Fig. 5. The absorption in the range 1200–1800 cm−1 appears obviously on TiO2. The band at 1483 cm−1 and in the region of 1260–1410 cm−1 could be assigned to be the signals of benzene derivatives and the corresponding aliphatic C–H bending vibration. Two weak bands shown in spectrum (A) at 1688 and 1711 cm−1 indicate the formation of CO bonding, implying the occurrence of the attack by the activated oxygen species or hydroxyl radical (OH) upon the carbon structures [12,13]. However, such change is not observed for In(OH)3. Spectrum (B) presents some bands related to the absorbed water and hydroxyl groups [14] in the range of 1300–1800 cm−1.

TEM images of indium hydroxide.

UV–vis DRS spectra of In(OH)3 (A) and TiO2(B) samples. (a) Fresh samples as reference; (b) samples after the photooxidation of benzene for 30 h and 15 h over In(OH)3 and TiO2, respectively.

FTIR spectra of TiO2 (A) and In(OH)3 (B). The spectra were recorded (a) before and (b) after photooxidation of benzene for 30 h (In(OH)3) and 6 h (TiO2) in dry O2.
The XPS spectrum (Fig. 6) and the XRD plot (Fig. 7) show that no obvious change in the oxidation state of In3+ (In3d5/2, 444.7 eV; In3d3/2, 452.2 eV [15]) and the crystal phases of the In(OH)3 sample occurs after the photoreaction, indicating that the IR and DRS spectral changes observed above are exactly due to carbonaceous by-products formed from benzene oxidation.
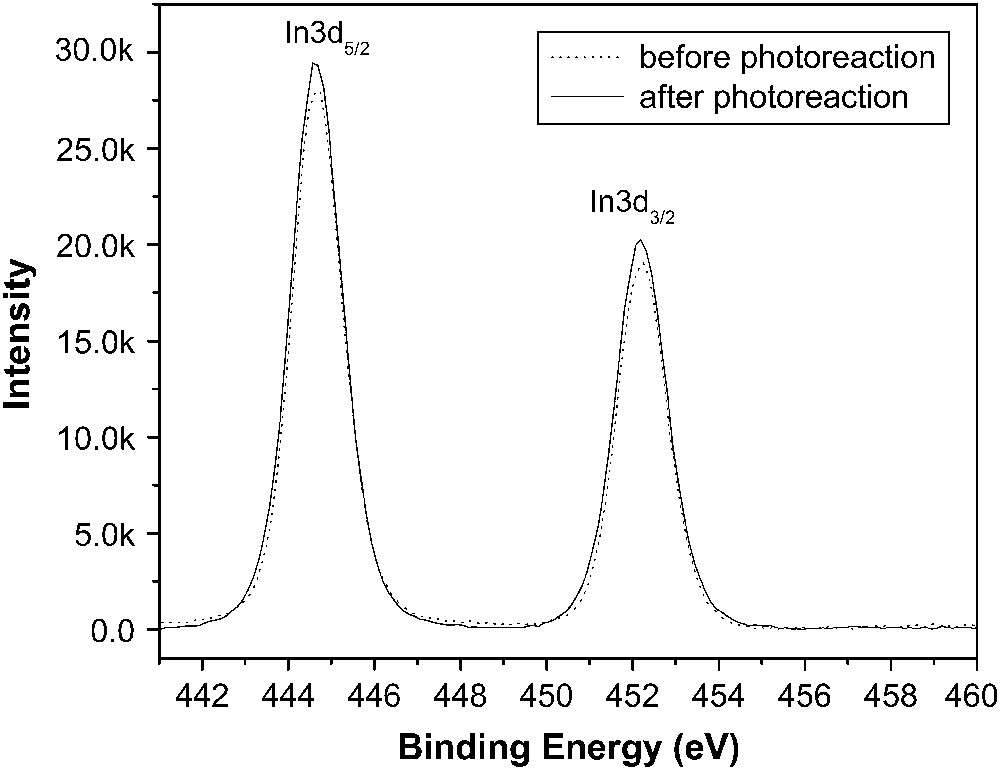
XPS spectra of In(OH)3 before and after benzene photoreaction for 30 h.
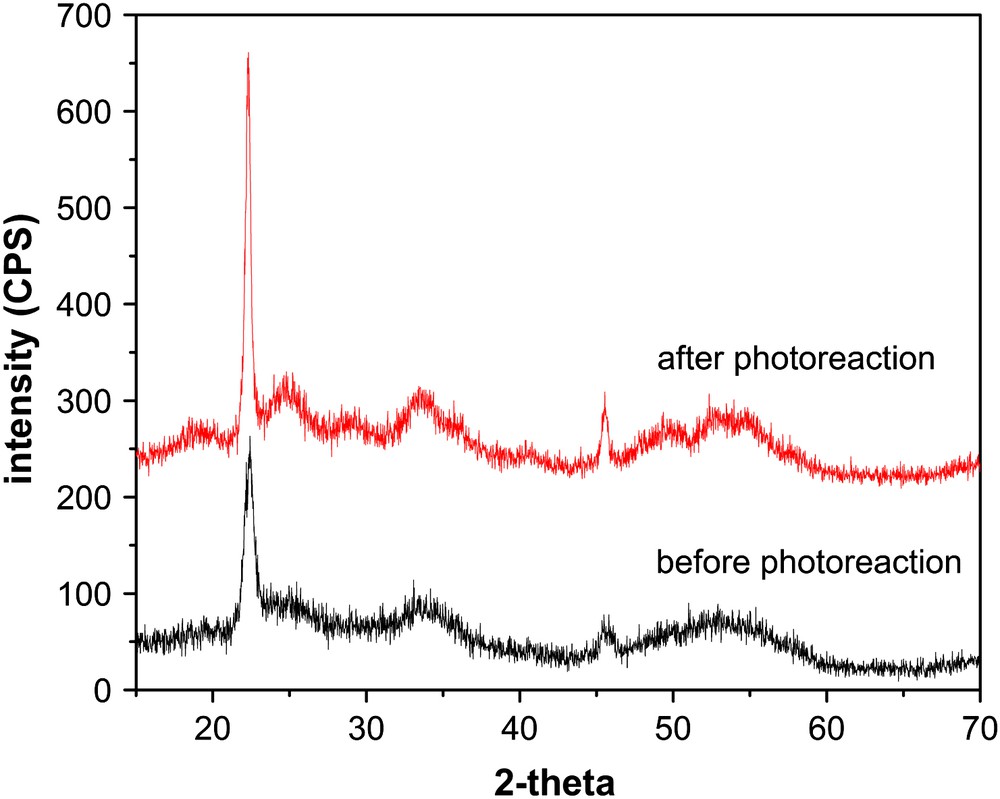
XRD patterns of In(OH)3 before and after benzene photooxidation for 30 h.
A number of studies showed that indium hydroxide is a typical semiconductor with 5.15 eV of wide band gap [16,17], and hence it can be excited exclusively by 254-nm UV light irradiation. Several factors can contribute to the observed difference in photoactivity between the two catalysts. First of all, the catalysts have different spectral properties, which would affect photon harvesting and thus the photocatalytic efficiency for benzene oxidation. When the reaction is performed with UV-light cut-off at 365 nm, no photooxidation of benzene is observed with In(OH)3, but the reaction is still efficient with TiO2. Secondly, direct hole oxidation and hydroxyl radical (OH) attack have been proposed as the reaction pathways for gas-phase photodegradation of benzene over TiO2 [4]. Einaga and co-workers have demonstrated that during benzene photooxidation the OH− groups on TiO2 surface are consumed, which can be regenerated by water vapor for sustainment of the catalyst activity [8]. In this respect, the excess OH− groups preserved by In(OH)3 would be beneficial for benzene photooxidation. Thirdly, the fate of both photogenerated holes and electrons are vital, once the semiconductor is excited by UV light. The reactivity of such photogenerated carriers would be different from one catalyst to another toward surface species, such as benzene, O2, and water. Since In(OH)3 has a larger band gap than that of TiO2, the photogenerated holes may have more positive potentials than those of TiO2 for direct hole oxidation of benzene or for generation of OH radicals to attack aromatic rings. Moreover, In(OH)3 has a higher surface area than TiO2 (the values of the surface areas are 123.8 m2 g−1 and 50 m2 g−1, respectively). This serves as another reason that In(OH)3 is superior to TiO2 as a photocatalyst for benzene degradation. The detailed mechanism is under further investigation.
4 Conclusions
In(OH)3 semiconductor exhibits high photoactivity and stability toward oxidation of benzene under UV irradiation. The maximal conversion of benzene and mineralization rate achieved are 33.4% and 56%, respectively, and are much higher than those obtained with TiO2 (9.5% and 24%). The change in spectra of the two catalysts before and after benzene photooxidation reveals that the carbonaceous by-product formed from photoactive process is uneasily deposited on the surface of In(OH)3, while it is easily deposited on TiO2. The strong photooxidation power of benzene can be attributed to the high hole oxidation potential and carbon deposits formed uneasily on the catalyst surface. The catalyst is expected to apply for other VOCs' degradation by molecular oxygen under UV irradiation.
Acknowledgments
This work was financially supported by the National Natural Science Foundation of China (20537010, 20573020, 20673020, and 20373011), the National Key Basic Research Special Foundation, China (2004CCA07100), and the Natural Science Foundation of Fujian, China (2003F004).