1 Introduction
Blue phases are cubic structures observed in a narrow temperature range between the isotropic and the cholesteric states, found in several pure compounds as well as in many mixtures. Although the blue phase (‘blue flash’) was reported by the very early investigators of liquid crystals at the end of the 19th century, research on the blue phase is of more recent date, probably beginning by the observation of an optically active cubic liquid crystal phase by Saupe [1] in 1969. The directed research towards a clarification of the structure of the blue phase is younger, with a series of fundamental studies beginning to appear around 1980, cf. Refs. [2–12]. Even faceted blue phase crystals were obtained and studied [13,14]. Today we understand that regular periodic lattices of an unusual kind lie behind the blue phases, either interlaced sets of vortex tubes [9] or disclinations lattices [11,12], or more complex geometries [14–16], but still very little is really known about the precise distribution of molecules, as suggested by the diversity of plausible models, except that they probably appear in many varieties from one compound to another.
It is now certified that there are several successive blue phases, one of which is probably amorphous. When progressively decreasing the temperature from the isotropic phase, one observes in turn:
- 1. The blue fog, supposed to be made of very small cholesteric germs, showing a strong rotatory power (also called Blue Phase III, or BP III). Thermal fluctuations (Brownian director motion) are well recognizable in the microscope.
- 2. The blue phases sensu stricto, which are probably cubic, since they are not birefringent and show Bragg reflexions in visible light and in the UV range, compatible with certain cubic systems. Grain boundaries are present and cubic shapes are recognizable in some germs and domains. These have a high rotatory power. One generally admits that there are two different blue phases, a high-temperature one, or blue phase II (BP II) and a low-temperature one, or blue phase I (BP I). These two phases were supposed to be made of either coalescent subunits (BP I), or separated, but ordered subunits (BP II).
- 3. The transition from blue phase I which leads to the cholesteric phase.
We have studied blue phases exhibited in a case where it is possible to observe drops, which resemble the famous Grandjean gouttes à gradins, obtained in smectic and, sometimes, in cholesteric mesophases [17,18]. But there are important differences as will be indicated below, mainly in the discussion. The blue-phase drops can become decorated by a ‘plywood’ pattern at the cholesteric transition. Our purpose is to present these observations, probably useful to elucidate some textures of the blue phase, and also to underline analogies with already described biological materials showing similar plywood architectures.
2 Materials and methods
The compound used in this study was synthesized by Nilsson and coworkers at Chalmers and originally described in Ref. [19]. Its formula is indicated below:
The blue fog and the blue phases were observed between 174 and 175 °C, when the compound was pure. However, even mounted between slide and coverslip, this compound degrades fast chemically at 175 °C and the blue phase average temperature is progressively decreased at temperatures around 160 °C, in a temperature interval as large as 5 °C. The preparations were controlled with a Mettler hot stage FP52 and examined in transmission polarizing microscopy, between crossed linear or circular polarizers.
3 Samples mounted between slide and coverslip
A blue fog appears if the isotropic phase is slowly cooled through the transition temperature. Observed between crossed polarizers, the colour was often greenish and remained unchanged when the stage was rotated. It was hence verified that the fog was not birefringent but presented a strong rotatory power. Fog germs coalesced, giving long arms (Fig. 1a), which also could join here and there. Such long contours are often characteristic of low interfacial energies.

Blue phase preparations in transmission polarizing microscopy, between crossed polarizers. (a) Isotropic phase (i), blue fog (f) and blue phase I and II (BP I and BP II), observed between slide and coverslip. Domains of BP II are larger than those of BP I. (b) Enlarged view of micrograph a, around the star in a (∗), with a +π/2 rotation. Several details of the polycrystal mosaic are visible: walls (w); some of them transform into grain boundaries (gb) and series of stripes are present in numerous domains.
A new temperature decrease produced a polycrystal structure growing from the fog, but direct interfaces also existed with the initial isotropic phase, which was not the fog itself (Fig. 1a). In these polycrystalline mosaics (Fig. 1a and b), the domains were separated by sharp walls in general and presented all the aspects described by Marcus [6]. We also verified in these phases the absence of birefringence and the existence of a very high rotatory power. More or less square germs also were observed (Fig. 2a) and other facetted morphologies at the contact of the fog, as in Fig. 2b.

More or less facetted blue-phase crystals, observed between crossed polars. (a) The fog (f) is in contact with the polycrystalline mosaic of blue phase and a more or less square germ (sq) develops in the fog. The high-temperature blue phase (BP II) shows well-defined domains, whereas much smaller ones correspond to BP I. (b) A possibly facetted extension of blue phase II in the fog (f), and some BP II drops.
Temperature gradients were very likely in such simple preparations and two distinct regions were often recognizable (Fig. 1a). They were supposed to correspond to the two blue phases: BP I showing smaller domains in the vicinity of the cholesteric phase, and BP II with much larger domains often at the contact with the fog (Fig. 1a). Vertical defect lines were viewed as points in BP I and II, but mainly in BP II and were either scattered in domains or aligned along grain boundaries as can be seen in Fig. 1b; we also observed walls which were continued by such grain boundaries.
When the temperature was slowly lowered, sets of stripes appeared mainly in BP I, some of them transforming into subdomains with contrasted colours in polarizing microscopy. These stripes also formed parallel series and probably corresponded to the interaction of the periodic structure with the glass interface, so that the periodicity depended on the obliqueness relative to this interface, with larger stripes when layers were close to horizontality. Many stripes ended along walls, whereas other ones ended at the level of the vertical lines, which might be essentially screw dislocations in a set of horizontal layers of cylinders such as those represented in Ref. [14].
Colours and dimensions of the mosaic domains were the two main characters differentiating these two regions, but we did not control the preparation thickness. Dark blue, clear blue and green were the usual colours of the large domains supposed to belong to BP II, whereas smaller domains present in BP I were yellow, orange, red and even brownish. Some domains were very large, the biggest dimension often exceeding 200 μm, while the dimensions were strongly reduced in BP I, often from 10 to 20 μm. We also often observed examples where these two regions were not so clearly separated, neither by the domain dimensions, nor by their colours observed in polarizing microscopy.
The optical rotation in domains showed a strong wavelength dependence. Using a white illuminating source and a monochromator, measurement of the dispersion was possible when the wavelength was different enough from the region of rotatory power inversion, which corresponded to the Bragg reflection of circularly polarized light. At such wavelengths we found an excellent contrast of the appropriately oriented domains, and also of certain stripes and defects. Our accuracy, however, was not high enough to allow an indexation of the crystallographic planes.
The cholesteric phase was observed very regularly at lower temperatures, and at the transition from the blue phase to the cholesteric one. The cholesteric twist-layers (or constant director surfaces) were generally horizontal, forming a planar texture with a uniform blue–green tint when examined between crossed polars, without any colour change when the stage was rotated. The orientation of these layers was easily evidenced by small shifts of the coverslip, and then turned oblique or vertical, with a birefringence clearly demonstrated.
To summarize these observations in the polarizing microscope, let us recall that a progressive temperature decrease from the isotropic phase of this material mounted between slide and coverslip showed successively: a grey or greenish ‘fog’, and later on, a mosaic phase often blue or green, with walls and defects and, finally at a lower temperature, a phase with more contrasted colours, green, yellow or red for instance, with smaller domains, before the passage to the cholesteric phase.
4 Free-surface drops attached to the glass interface
A very small quantity of isotropic phase was stretched at the slide surface (with a coverslip edge), in order to obtain a set of small ‘free-surface drops’, with two interfaces, one with the glass and the other one with the air. The contact with air probably accelerated chemical degradation and the transition temperature rapidly got lower than 170 °C. The presence of fog could not be ascertained in these small droplets, and the blue phase possibly nucleated from the initial isotropic liquid, along the air or the glassy interface.
Concentric lines – in the topological sense – appeared in the blue phase growing in such drops and were well resolved in the microscope, all together simultaneously, so that a focus change was not required to see all the lines (Fig. 3a). They strongly resembled the contours of steps described in certain smectic drops (Fig. 3b), and also in cholesteric liquids, the well-known gouttes à gradins or stepped drops, first studied by Grandjean [17,18]. However, the concentric lines of the blue-phase drops were very different from the level lines of stepped drops, since they all lie at the level of the glass boundary, or in its close vicinity, as will be confirmed in the discussion. This means that, instead of horizontal twist-layers forming steps at the air interface, we have slightly curved twist-layers which superpose and meet the glass boundary along the contour lines (Fig. 4). A stepped structure is not excluded at the air interface, but we did not observe it in our material. Since these drops were very thin, the refractive effect was small, and a good focus was obtained for all the concentric lines at the glass boundary. These lines often showed alternating contrast, leading to easily distinguished odd and even lines, or pairs of lines (Fig. 3b).
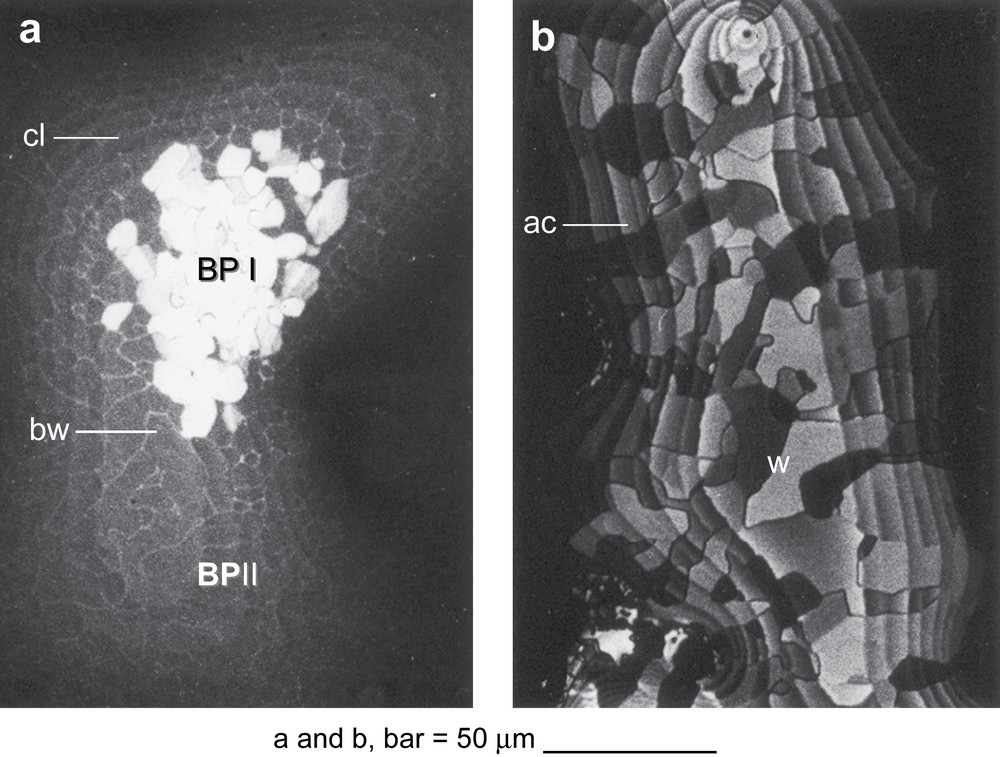
Free-surface drops showing topologically concentric contour lines. (a) These contour lines (cl) resemble level lines, but are likely to lie at the glass contact. This is the high temperature blue phase (BP II), with brilliant walls (bw) showing clear divisions into domains. In the center of the drop the white domains correspond to an overexposed view of the growing blue phase I (BP I), the overexposure being necessary to have a good contrast in BP II. (b) Free-surface drop of blue phase I, with similar concentric lines, resembling steps and showing alternating contrast. These lines are also supposed to lie at the glass interface. Walls are present and limit domains showing different contrast.

Representation of a blue-phase drop considered in two perpendicular planes, one vertical (a) and one horizontal (b). (a) In the vertical section, the drop is attached to a coverslip (cs) and presents a layered structure (L str) close to horizontality, but slightly curved, so that layers end obliquely at the vitreous interface (vi). The layered structure (L str) has been represented by continuous lines alternating with dotted lines. Each continuous line corresponds to a cylinder of double twist in longitudinal view, whereas each point represents a cross-section of such a cylinder (as recalled in Fig. 7b). Actually there are three perpendicular directions of such cylinders, but the vertical ones were omitted in this representation for the sake of clarity. The dashed line corresponds to the interface (LC-a) between the drop of liquid crystal and air, but the arrangement of the liquid crystalline structure remains unknown at this level. (b) Idealized representation of decorations observed in the vicinity of the glass interface (vi), at the end of the transition from the blue phase I to the cholesteric liquid. Vertical dashed lines indicate the correspondence between figures (a) and (b): the vertical section in (a) lies along mn in the horizontal representation along the glass interface (b). Striations align within concentric bands, along two alternating directions at right angle (or close to). Compare with the micrograph of Fig. 5b where the pattern is much less regular.
The high-temperature phase BP II was very dark in these free-surface drops, when examined between crossed polarizers, but the concentric lines were clearly recognizable, and also a set of brilliant walls separating domains (Fig. 3a). We suppose that these drops corresponded to BP II, the high-temperature blue phase, but with some hesitation, since they remained extremely dark, without any sharp contrast differentiating them from the black background. The blue or green domains of BP II, observed between slide and coverslip, and even the blue fog, were much better differentiated from the isotropic liquid, but the sample thickness was also much larger than in the free-surface drops.
When BP I nucleated in these dark drops, the light was suddenly reestablished, as shown in the center of the drop of Fig. 3a. Rapidly all the drop transformed into BP I, and domains limited by sharp walls formed a mosaic system completely independent from the concentric lines (Fig. 3b). The walls which appeared to be uniformly green in BP II drops were maintained at the transition to BP I, with some contour transformations. The contrast of these walls and concentric lines was then strongly reinforced (Fig. 3b). The drops became highly coloured at the transition, orange initially, red later, and even brown, when the temperature was further decreased. These drops never showed screw dislocations, neither at low temperature, nor in the high-temperature phases (BP I and BP II).
Walls formed a mosaic in each drop of blue phase and moved slightly, as could be seen from comparisons between successive views, when the temperature was modified. The domains presented different contrasts, changing abruptly along walls, but contour lines crossed walls without significant distortions (Fig. 3b).
A schematic interpretation is proposed along a vertical section in Fig. 4a and in projection onto the horizontal plane of the preparation in Fig. 4b. In the drop the lattice planes formed by the blue phase are not flat and stacked horizontally as in usual stepped drops, but curved, so that the superposed twist tubes come to end at the glass interface.
The transition to the cholesteric phase was followed in these drops (Fig. 5a). Parallel and equidistant stripes formed probably in the vicinity of the glass boundary and later in the bulk and were well resolved in polarizing microscopy (Fig. 5b). However, these drops were very thin with 10–20 lattice planes, the whole thickness being possibly less than 5 μm, so that the precise level of the observed stripes remained unknown. The orientation of stripes changed abruptly along certain walls. When the temperature was decreased, a second set of stripes appeared, superimposing or not on the preceeding one, at an angle of 90°, or along a somewhat different direction. The first array of stripes then vanishes. A third set of stripes appears in turn, and eventually a fourth one. The successive sets of stripes made angles close to 90°, so that the whole structure was reminiscent of that found in plywoods. An example of the successive directions of stripes in a domain is illustrated in Fig. 6.

Transition to the cholesteric phase observed in free-surface drops of blue phase I (crossed polarizers). (a) At the beginning of the transition, equidistant stripes are visible and there are shifts of director orientation across some walls between the adjacent domains. (b) When the transition to cholesteric phase has been completed, the cholesteric layers are mainly horizontal, but corrugated with the folds highly contrasted in polarization microscopy. The orientation of folds probably align along crystallographic directions which preexisted in the blue phase at the air interface. Concentric zones are seen with folds showing two alternating orientations, roughly parallel to the two edges of the micrograph.

Drawing of the evolution of a contour-decorated drop at the blue phase – cholesteric transition. (a) Series of contour lines just before the transition. (b) First parallel stripes showing orientation 1. (c) New stripes 2 appear, superimposing on direction 1 and finally replacing it. (d) Stripes of direction 3 form and superimpose on those of direction 2. The contour lines become sinuous. (e) The phase becomes highly birefringent and the folds form, following direction 4 which is close to direction 2. (f) Schema recapitulating the successive orientations within this domain.
Let us recall that a plywood consists of thin sheets of wood stuck together, with the fibers of adjacent layers at right angle to each other, as shown in Fig. 7a. When the cholesteric transition is accomplished, one observes a distorted texture easily obtained from a planar texture. One can say, in a first approximation, that the twist-layers are horizontal, but they are more or less corrugated, and the long axes of the parallel folds are aligned along the last series of stripes observed during the transition.

Some models. (a) Schematic representation of a ‘plywood’ structure, and the observed patterns arising from a succession of steps, with an oblique section, indicating the origin of the ‘herring-bone’ patterns. (b) Representation of the blue phase model proposed by Meiboom et al. [9], supposed to correspond to BP II. A triply orthogonal arrangement of cholesteric tubes leads to a simple cubic orientational lattice. Each tube is cholesteric and the tubes touch laterally. When considering only horizontal tubes, one recognizes a directional structure which is close to that of a plywood. (c) Alternative arrangement of the vortex tubes in BP I (redrawn after Ref. [13]). (d) Arrangement of the helical fibrils, lying horizontally in the skin of certain sea-worms. The dotted circles correspond to the vertical cytoplasmic extension, often accompanied by some vertical fibrils (after Ref. [24]).
Some free-surface drops were prepared to lie on a rubbed glass surface, an ancient technique used to align nematics and other liquid crystals along a preferred direction on a glass slide and to obtain liquid monocrystals. One then observed, just at the end of the transition to the cholesteric phase, a remarkable pattern decorating the concentric bands. We suppose that the twist-layers close to the transition interface became corrugated and the resulting folds observed between two successive concentric lines were aligned along a constant direction, either parallel or perpendicular to the rubbing direction. The orientation changed by a right angle (or close to) at each successive step (Fig. 5b). These patterns were not visible in all drops. The best observations were obtained with very flat drops, showing large domains. The presence of walls, which were not the contour lines, did not facilitate the observations. When lines limiting the steps run at 45° with respect to the rubbing axis, this arrangement forms the so-called ‘herring-bone patterns’, their origin being indicated in Fig. 7a.
Such pictures strongly suggest that the cholesteric transition decorates an initial structure comparable to that of a plywood, as shown below in the discussion. The cholesteric structure obtained seems to be a pseudomorph of the initial blue phase. If such decorated droplets are slowly reheated, the complete story is reobserved, the process appearing to be reversible, but let us say at first view. The successive sets of stripes which appeared at a given place reappeared, following the opposite order in time, but some walls limiting domains show slight transformations, which are not fully reversible during these transitions.
5 Discussion
The concentric lines of blue-phase drops strongly resemble the ‘pairs of steps’ observed by Pieranski et al. [14], in the facet of BP I crystals lying along the glass interface, and particularly the ‘pair of steps’ associated with a screw dislocation. These precise observations of paired lines present at the glass interface and similar to our paired concentric lines in free-surface BP drops were a convincing argument for us to think that they were also lying at the glass interface, so that these blue-phase drops were not stepped in the classical meaning of the term when applied to liquid crystals. Pieranski et al. also observed steps, on the opposite horizontal facet, forming a spiral contour, around the emerging screw dislocation, at the interface with the isotropic liquid, this being different from our interface with air. We did not observe any screw dislocations in our free-surface drops of blue phase. Meiboom et al. [9] in 1981 described one model of blue phase, indicating that other possibilities could exist. They proposed to “interlace space with sets of tubes, so constructed that the director points along the tube axis in the center, and rotates about the radial direction, as one moves out, making an angle of 45° on the surface of the tube. If, for example, sets of such tubes are placed with their axes along three orthogonal directions, so that mutually orthogonal tubes touch, but do not intersect, we obtain a simple cubic structure…”. We have illustrated such an arrangement in Fig. 7b. It can be seen that the director, a unit vector n, makes 90° turns between any two orthogonal tube sets. This model does not indicate the distribution of molecules between the tubes. However, several authors considered this question, finding that the molecules have to arrange around defect lines, −1/2 disclinations, which run along the four ternary axes of this cubic structure, corresponding to the four long diagonals of the cube [11,12]. The core of these disclinations is either continuous or discontinuous, along segments which alternate at the centers and at the vertices of each cubic cell. As quoted above, one has other plausible models, for BP I and BP II, and for instance a variant of the tube arrangement is represented in Fig. 7c, which could represent BP I, whereas Fig. 7b would better correspond to BP II.
At the transition from BP I to the cholesteric phase, complex phenomena occur as can be seen from Fig. 5. There are three sets of lattice twist tubes which are mutually orthogonal in BP I and we suppose that the cholesteric twist-layers form from the preexisting twist in one direction, the horizontal one in our preparations. In our drops described above, the cholesteric twist-layers arrange almost horizontally as the twist producing the stepped aspect of the blue-phase drop. The parallel stripes which appear in the first steps of the transition to the cholesteric phase do not resemble cholesteric layers. They might be due as quoted above to a regular corrugation of layers, but this is not demonstrated. Such corrugations are known in cholesterics and could occur in the blue phase, since the cholesteric pitch differs from the thickness of the corresponding four twist tubes in the blue phase.
Now, let us simplify the models: consider only the horizontal tubes (or threads, or fibrils…) aligned along two orthogonal directions, and forget the vertical tubes and the cholesteric structure within tubes. One has something which is reminiscent of a ‘plywood’, where layers of wood fibers are added in successive orthogonal directions. Such layers observed obliquely, along steps, form the pattern described above, in the free-surface drop (Fig. 7a).
It is striking to find biological structures very close to this plywood model. For instance collagen is a triple helix associating three long polypeptide chains, of equal length, a biopolymer which often assembles into fibrous systems, showing a cholesteric geometry, as in certain connective tissues and in fibrous matrices of bones [20,21]. One also finds plywood structures in skeletal tissues, with superposed layers of aligned fibrils, alternating along two perpendicular orientations [22]. Oblique sections of such structures reproduce the patterns observed in blue-phase drops, at the cholesteric transition. Studies of collagen distribution in the skin of certain worms [23,24] show other examples of structures very close to the model due to Meiboom et al. [9], but there are some differences with respect to that model:
- a. Vertical fibrils are absent, or strongly reduced, or even replaced by a thin cytoplasmic extensions (Fig. 7d).
- b. Each bundle shows only a very slight cylindrical twist, limited to a maximum angle of 30°, instead of the angle of 90° in each vortex of blue phase models.
- c. The fibrous matrices are not fluid in biological systems.
In fish scales, one also often [25] observes a three-dimensional arrangement of collagen bundles similar to that of Fig. 7a, with similar differences from blue phases.
In short, this study shows the existence of highly structured drops of blue phases, different from usual stepped drops in liquid crystals. At the glass interface, these drops can be decorated at the cholesteric transition, and the observed patterns are strongly reminiscent of a ‘plywood’ structure, which was not only invented by men, but used very long before by organisms, in many examples of tissues. Blue phases could intervene as a morphogenetic factor in many biological analogues of liquid crystals, especially for biopolymers such as collagen, which self-assemble into cholesteric phases, which further stabilize into cholesteric-like, or plywood-like fibrous matrices, and even into triply orthogonal networks [25].