1 Introduction
Heavy metals often found in wastewater from electroplating, metal finishing and preparation, tannery, etc. are of considerable concern as these elements are known to be highly toxic to people and environment. If these metal ions are directly discharged into the sewage systems, they may seriously damage the operation of biological treatment and make the activated sludge unsuitable for application to agricultural land [1].
Traditional methods of metal removal such as activated carbon adsorption or ion exchange, because of high capital cost, makes its use not much attractive. Therefore there is a need to develop low cost and abundant materials that can sorb metals effectively. The literature reports frequently new sorbents, such as inorganic (calcined coke, natural zeolites, fly ash, blast-furnace slag), living micro-organisms (bacteria, fungi, algae), cellulosic materials (sawdust, coconut husk, palm fibber, linseed straw), which till today aren't suitable for large commercial applications [2–4].
As an alternative sorbent for scavenging heavy metals from solutions, we present now the volcanic lava ash, a siliceous material released into the atmosphere from volcanoes in quantity which is estimated up to 150 million tons per year. In Sicily, Haulett and Zettwoog [5] observed that the normal activity of the Mount Etna volcano is characterized by more or less continuous emission of lava ash up to 300 tons per day. The effect of this flying material on groundwater, rainwater, agricultural products is important, as well as on human health, since the harmful components of these inhaled particles (heavy metals, acid gases) may create damage in the lungs [6,7].
The present study aims at characterizing the sorptive capabilities of the lava ash released from the Mount Etna volcano through isothermal tests, pH effects, solid–solute ratio, initial metal concentration.
2 Materials and methods
Lava ash was collected near the active submittal craters of the Mt. Etna volcano, at about 3000 m above the sea level. The granulometric fraction of 0.3–0.5 mm, the more abundant in that area (about 84%, w/w), was selected. To eliminate sorbed gases and soluble elements, it was washed with distilled water (1:1, w/w) and then dried at 110 °C. Its elemental composition, shown in Table 1, was measured by a Genesis ICP-MS spectrometer by Fisons Instruments Ltd. Dissolution of the lava ash samples was carried out with a microwave digestion system using 20 mL of a mixture of ultrapure acids (HF:HNO3:HClO4 = 5:1:1) added to 0.5 g of sample. Data were assessed for accuracy and precision using a rigorous control system including reagent blanks, triplicate samples, and certified international reference materials.
Main elemental components of the Mount Etna lava ash
Element | Concentration (mg g−1) | Element | Concentration (mg kg−1) |
Al | 168 | Ag | 0.1 |
Ca | 10.8 | As | 132 |
Fe | 77.9 | Cr | 24.9 |
K | 33.4 | Cd | <1 |
Mg | 53.1 | Cs | 0.6 |
Mn | 1.42 | Cu | 106 |
Na | 59.8 | Pb | <2 |
Si | 155 | Sb | <2 |
V | 0.15 | Zn | 160 |
The density of this volcanic fraction was 2.65 g cm−3. In 10 g L−1 aqueous mixtures with pH ranging from 3.0 to 8.0 the lava ash released in solution under 1% of its components.
All chemicals used were of analytical reagent grade. To prepare both mixtures and solutions, pure water first deionized and then doubly distilled was used (electric conductivity: 0.04 μS cm−1). The standard metal ion solutions were prepared by dissolving the relative metal nitrate salt in pure water. The amount of metal ion in solution was determined also with a ICP-MS spectrometer). Each mixture was analysed in triplicate. The detection limit was calculated as three times the standard deviation of the blank.
To test the system at equilibrium, the following parameters were used: sorption efficiency of the system (% Rem) indicating the percentage of removed metal ions relative to the initial amount, and sorption capacity of the substrate (Cs), which is the metal ion amount sorbed from unitary solid mass (mmol kg−1). These parameters have been calculated as indicated below:
2.1 Batch experiments
The sorption test was realised by adding a known quantity of volcanic ash into metal ion solutions. The temperature was constant (20 ± 1 °C) during experiments. After some contact time, the mixture was centrifuged and the supernatant was analysed for the studied ions. The amount of metal ions sorbed by the lava ash was calculated by mass balance. In our balanced systems a centrifugation time of 3 min at 35,000 g assured an excellent recovery of the solid phase (about 99.7%). The analytical precision, determined as percentage relative standard deviation for ten consecutive measurements, was in the order of 2.0%.
During all the experiments at constant pH, the value was adjusted (pHadj), as it was varying more than ±0.1 unit, by small additions of 0.1 M HCl or 0.1 M NaOH aqueous solution. In order to calculate the degree of metal ions recovered at each value of both pHadj and initial metal ion concentration, blank experiments were carried out according to the batch test methodology, without adding sorbent. Sorption data of each test were corrected subtracting the equivalent blank experiment.
2.1.1 Effect of contact time
The experiments were carried out by preparing a 10.0 g L−1 mixture of lava ash in 0.1 mM metal ion solution. The pHadj was 6.0 ± 0.1. After mixing, aliquots (3 ml) were withdrawn for 12 h at 5-min intervals in the beginning, at 15-min intervals in the second part of the experiment, at 60-min intervals in the last part. The total volume of the solution withdrawn for analysis was about 3% of the initial reacting mixture. Equilibrium in the system, that is a decrease of the solute concentration lower than 2% per hour, was achieved already after a 90-min contact time. According to analytical problems of the system and requirements of industrial scale process, a contact time of 2 h was used for our tests.
2.1.2 pH effect on metal sorption
At pH values next to 8.0, the studied metal ions plunge down visibly into solution. Therefore nine 0.1 mM metal ion solutions, each at different pHadj values varying from 3.0 to 7.0 with 0.5 unit intervals, were prepared. The volcanic substrate (1.0 g) was added to 100 mL of the metal ion solutions and the mixtures were stirred for 2 h. After centrifugation, the supernatant was analysed for metal ions in solution.
2.1.3 Effect of solid–solute ratio (Rss)
The sorption efficiency (% Rem) improved with increasing solid–solute ratio (Rss = p/Ci kg mol−1). For all our tests an Rss value of 100 was found to assure a sorption efficiency varying from 20 to 80%, which was found optimal for the analytical conditions.
2.1.4 Sorption isotherm
For the single-element tests the sorption isotherms of each ion were obtained by increasing the initial concentration up to 5.0 mM. Mixtures of 10 g L−1 lava ash were used. Four series of experiments were carried out, one for each ion according to the standard batch test methodology described earlier. The pHadj was the pHopt determined as reported previously in Section 3.1.1.
2.1.5 Multi-element test
For the multi-element test, 10 g L−1 mixtures of lava ash in mixed solutions of three ions (Cu2+, Cd2+, Cr3+) were used. The initial concentrations of each ion in the three series of experiments were 0.025, 0.05 and 0.1 mM. The suspensions were stirred for 2 h and the centrifuged supernatants were analysed for metal ion in solution, as reported above for the single-element test.
The pHadj value was 7.0 ± 0.1.
2.2 Desorption experiments
Leaching tests by water saturated with CO2 and aqueous solution of acetic acid were carried out. Exhaust lava ash samples, resulting from multi-element sorption experiments, were used.
2.2.1 Test by water saturated with CO2
Desorption experiments were carried out by using pure water saturated with CO2 with an initial pH value of 4.6 ± 0.1. A 10-g sample of exhaust lava ash was placed into 0.2 L of leaching solution. After shaking for 24 h at 20 ± 1 °C, the mixture was filtered by 0.45 μm Millipore filter and the eluate analysed for metal ion concentration.
2.2.2 Test by aqueous solution of acetic acid
These experiments were carried out by using a 0.5 M acetic acid aqueous solution. A 10-g sample of exhaust lava ash was placed into 0.2 L of leaching acetic acid solution. The pH was adjusted to 5.0 ± 0.1 during the experiments by small additions of 0.5 M acetic acid aqueous solution. After shaking for 24 h at 20 ± 1 °C, the mixture was filtered by 0.45-μm Millipore filter; the metal ion content in the eluate was determined by ICP-MS spectrometry.
3 Results and discussion
The lava ash is a material of complex structure and heterogeneous composition. Therefore the sorption term has been preferred here, according to Farley [8], idea which relates them to the whole processes of sorbate transport from solution to the solid phase, the adsorption term referring limitedly to the formation of the covering surface monolayer proposed by the Langmuir theory. The efficiency to remove metal ions from solutions and to immobilize them has been determined by batch tests, as reported previously in Section 2.1.1.
3.1 Batch experiments
Various experimental parameters have been tried to verify their influence on the sorption process.
3.1.1 pH effect
In Fig. 1 pH is plotted vs. time parameter of mixtures prepared with different contents of lava ash (5, 50, 250, 500 and 1000 g) in 1 L of reagent grade water.
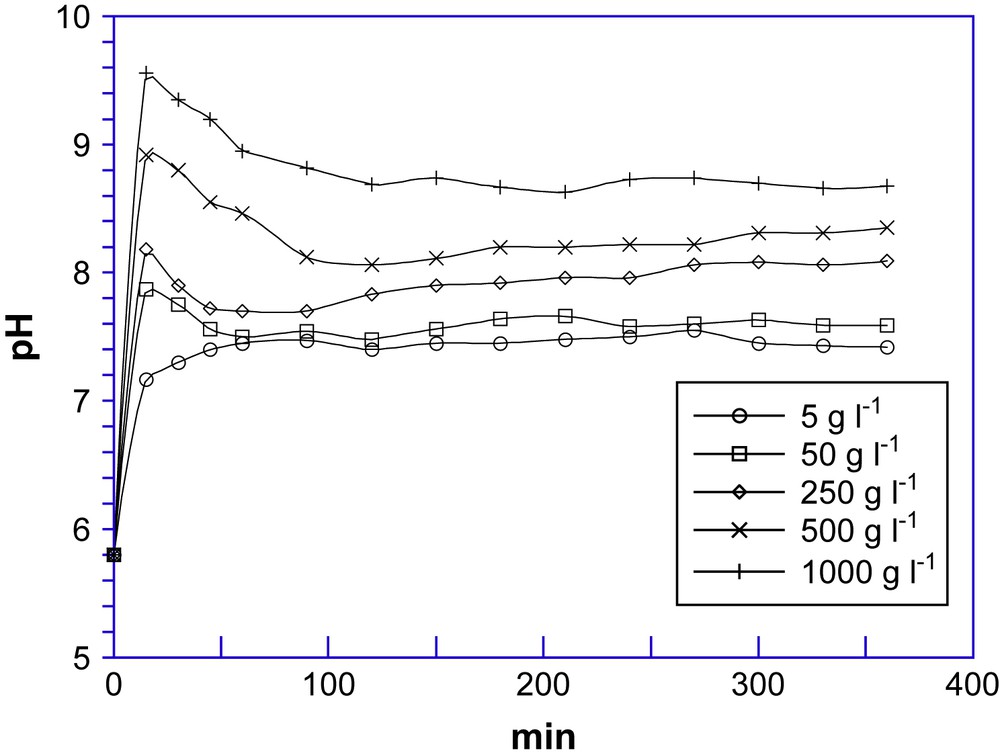
Effect on pH of various lava ash mixtures.
In the first minutes, the pH of the mixtures rises quickly due to both a fast and large dissolution of alkaline compounds (mainly hydrolysis of metal oxides and alumino-silicates) and a release into solution of hydroxyl ions from the oxo groups (MxO) present on the surface of sorbent as reported here:
MxO + H2O ⇄ [MxOH]+ + OH− | (1) |
The following slow decrease in pH may be correlated with the buffering power of the solid phase by progressive acid–base interactions, in agreement with Eq. (1). The pH final values are alkaline values, depending on the lava ash content in mixture.
As solution acidity influences the sorption process which is the result of competing phenomena (hydrolysis and precipitation of interactive ionic species, protonation and deprotonation of substratum, etc.), sorptive experiments at constant pH (pHadj) have been carried out.
Fig. 2a shows the sorption efficiency for Cd2+, Cu2+ and Cr3+ initial ion solutions (Ci = 0.1 mM) at varying pHadj values. One observes the classical bell curve which defines the range where sorption is remarkable or poor or void (sorption edge). The removal of all metal ions is negligible at very low pH values and it is becoming optimal over a limit value of pH (pHopt), typical for each ionic species at the same water concentration. A graphic method to estimate the pHopt value is shown in Fig. 2b: it is the pH intercept of the intersection point of the two tangents at the flex point.

(a) Percent removal of Cd, Cu and Cr ion species as a function of pHadj. (b) Graphic method to estimate the pHopt value.
At the initial concentration of 0.1 mM, the Cu ions showed a sorption efficiency larger than Cd and Cr ions.
To explain this trend, the following assumption is made. At low pH values, when the system is well buffered, the oxo groups (MxO) of the adsorptive sites bind largely H+ protons:
MxO + H+ ⇌ [MxOH]+ | (2) |
However, at moderate pH values, other ionic species in solution are binding, according not only to the mass law but also to their electrophilic power, polarizability, electronegativity, electron affinity, chemical coordination characteristics, solvatation, etc.
MxO + Me(H2O)ny+ ⇌ [MxOMe(H2O)n−m]y+ + mH2O | (3) |
Then θ ionic species can be sorbed effectively only at its own pHopt value, when equilibrium (3) is prevailing against (2).
On account of the highly polarized character of the surface active groups (oxides of silicon, aluminium, iron, alkaline metals), the lava ash could be supposed as a Pearson Hard Base [9] and then, according to the Hard or Soft Acids and Bases (HSAB) theory, it should show a greater affinity towards hard ions. In the scale proposed by Pearson [10], the values of absolute hardness (η) for Cr3+, Cd2+ and Cu2+ ions are 10.3, 9.1 and 8.27, respectively.
These η values show a good linear relationship with the corresponding pHopt values of 6.0 for Cr3+, 6.5 for Cd2+ and 6.8 for Cu2+ ions assessed here.
This fitting is very interesting and worthy to be reported here, but beyond the aims and scope of this study. We are investigating further on this finding.
3.1.2 Thermodynamic factors
Fig. 3 shows the sorption capacity (Cs = g kg−1) of the lava ash with increasing initial metal ion concentration (Ci) up to about 5 mM. In the mixtures, the lava ash concentration was 10 g L−1 and the pHadj value was the pHopt of each ion.
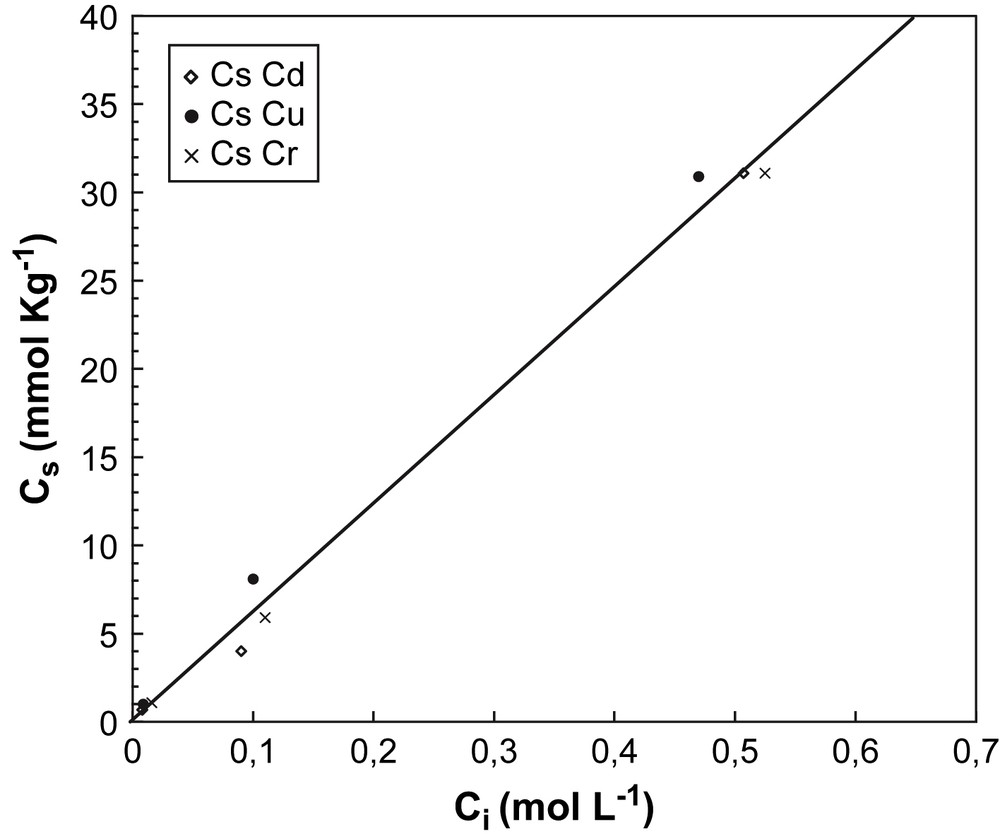
Amount of metal ions sorbed by the unitary mass of Cd, Cr, Cu (Cs = mmol kg−1) vs. the initial solute concentration (Ci = mol L−1).
In the studied concentration range, the Cs values fitted almost linearly with the Ci values and a second stage of sorption (multilayer sorption) was not evident.
The effect of the initial solid–solute ratio (Rss) on sorption efficiency at the pHopt value of each ion species is shown in Fig. 4. The trend of the curves for Cd2+, Cu2+, Cr3+ ions confirms the different affinities of the sorbent towards the three ions. Sorption efficiency increases at higher values of the solid/solute ratio, because the number of free active sites is greater.
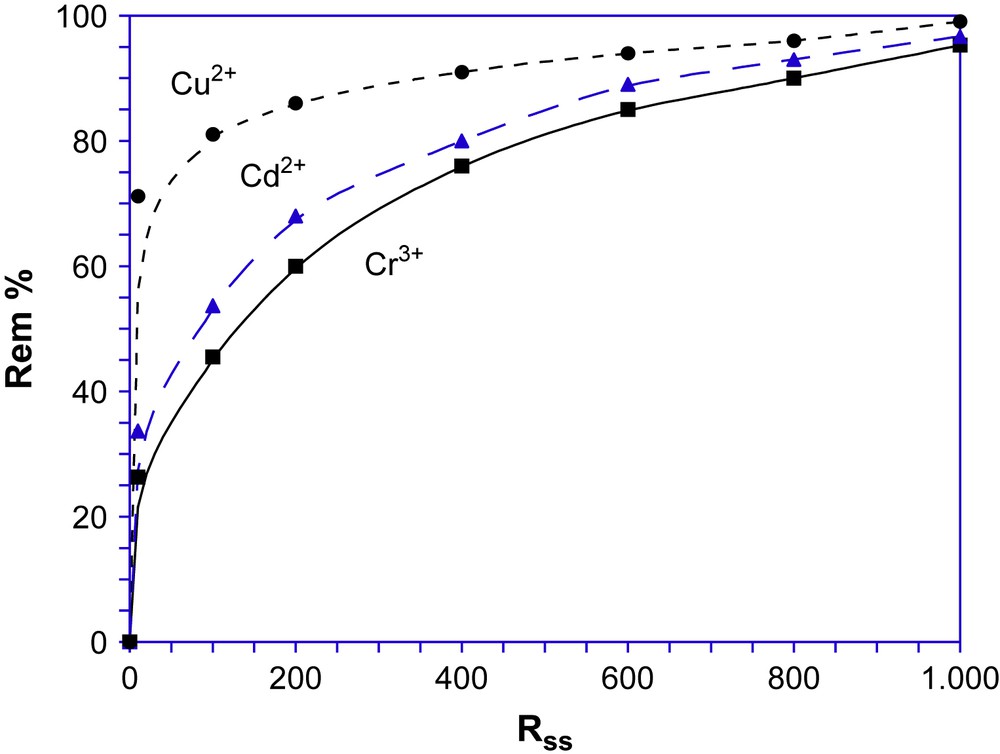
Sorption efficiency (Rem %) vs. the initial solid–solute ratio (Rss = kg mol−1).
3.1.3 Sorption isotherm
Between the applied sorption models (Langmuir, Freundlich, Kolbe), the isothermal data from the pHopt test showed a good correlation only with the Freundlich isotherm (Cs = KF Ce1/n), as is seen in Fig. 5. The relative values of KF (sorption capacity), 1/n (sorption intensity), and r2 (correlation coefficient) found for Cd2+, Cu2+ and Cr3+ ions at pHopt values are listed in Table 2.
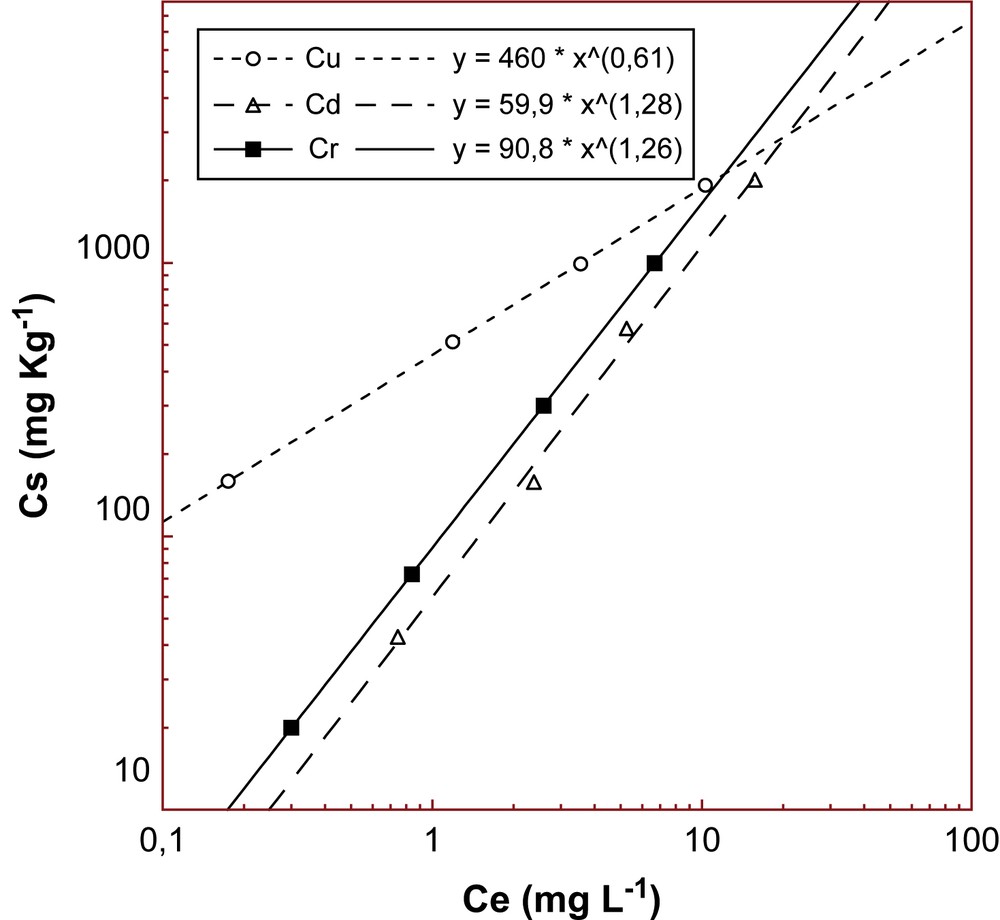
Freundlich sorption fitting curves.
Parameters calculated by the linearized form of the Freundlich isotherm
Element | pHopt | KF | 1/n | r2 |
Cd2+ | 6.5 | 59.9 | 1.28 | 0.998 |
Cr3+ | 6.0 | 90.8 | 1.26 | 0.999 |
Cu2+ | 6.8 | 460 | 0.61 | 0.999 |
3.1.4 Multi-element test
To verify the sorption efficiency of the process in mixed solutions of different ions (Cd2+, Cu2+ and Cr3+), multi-element experiments were performed. A pHadj value of 7.0 ± 0.1 was used, because at this value a satisfactory removal of all ions had been observed (Fig. 2).
Table 3 shows that, according to the single-element test data plotted in Fig. 4, Cu ions are sorbed preferably rather than Cd ions and Cr ions. Besides, on increasing initial metal ion concentration (Ci), the sorption capacity (Cs) improved but the sorption efficiency (% Rem) decreased because the active sites were saturated. All the analytical results, particularly at low initial metal ion concentration, were found comparable with those from single-element tests. Then no competitive but only selective effects were working.
Multi-element sorption tests at different initial concentrations of each metal ion at pH 6 with a solid suspension of 10 g L−1
Element | Ci = 0.1 mmol L−1 | Ci = 0.05 mmol L−1 | Ci = 0.025 mmol L−1 | |||
Cs (mmol kg−1) | Rem (%) | Cs (mmol kg−1) | Rem (%) | Cs (mmol kg−1) | Rem (%) | |
Cadmium | 6.81 | 68.1 | 4.52 | 90.4 | 2.40 | 96.1 |
Copper | 8.84 | 84.4 | 4.93 | 98.7 | 2.49 | 99.5 |
Chromium | 4.93 | 49.3 | 3.45 | 69.1 | 2.01 | 80.6 |
Total | 20.28 | 45.6 | 12.90 | 86.0 | 6.90 | 92.0 |
3.2 Desorption experiments
To check whether metal ion can be desorbed from the exhaust lava ash, two tests were carried out to simulate the leaching as well by rainwater (test by water saturated with carbon dioxide) as by acid percolates (test by aqueous acetic acid). Table 4 reports the metal concentration in the exhaust lava ash sample in the beginning of the experiment (Cs) and in both final eluates (Cdes).
Leaching test by CO2 or acetic acid aqueous solution
Element | Cs (mmol kg−1) | Cdes | |
CO2 (mg L−1) | CH3COOH (mg L−1) | ||
Cadmium | 765 | 0.12 | 2.94 |
508 | 0.04 | 1.44 | |
270 | <0.01 | 0.68 | |
Copper | 543 | 0.07 | 1.55 |
313 | <0.01 | 1.03 | |
158 | <0.01 | 0.68 | |
Chromium | 256 | 0.11 | 1.09 |
179 | 0.04 | 0.68 | |
104 | <0.01 | 0.33 |
Findings indicate the low reversibility of the sorption process at the pH values of both tests. Leaching by an acetic acid solution (pHadj = 5.0 ± 0.1) is more effective than by CO2-saturated water. In this last test, the concentrations of copper and total chromium were under the limit level fixed by the European legislation for wastewaters [11] in order to protect the aquatic environment.
4 Conclusions
This study shows the effectiveness of volcanic ash to remove metal ions from aqueous solutions. The efficiency of the sorption process appears to be correlated to various experimental parameters and particularly to the pH of the medium.
The low cost, the optimal property of settling, the good stability in aqueous solution, the effective sorption capacity suggest that this material could be certainly considered for an economic treatment system of effluents.
Today the Mount Etna lava ash is considered as a polluting product for its impact on different environmental sectors (air, water, ground, economic resources, environmental hygiene). Then, the possibility of using it effectively as a sorbent in depolluting processes appears interesting and of notable importance, as well technological as economic.