1 Introduction
β-Functionalized enamine derivatives are useful precursors in synthesis as they combine nucleophilicity of the enamine and electrophilicity of the enone motives. Such derivatives are key intermediates for the synthesis of biologically active compounds such as α- [1] and β-aminoacids [2], alkaloids [3], peptides [4]; and heterocycles [5] including 1,4-dihydropyridines [6], pyrroles, oxazoles, pyridinones, quinolines, dibenzodiazepines, tetrahydrobenzoxazines, tetronic acids, azasteroids,(1H)-pyridin-2-one, pyrazolo[1,5-a] pyrimidine and isoxazole derivatives have also been prepared from enaminones [5,7]. They exhibit a wide range of biological activities for various diseases and have found applications as anti-inflammatory [8], anti-tumor [8a,9], anti-convulsant agents [8a,10], antibacterial [11]… However, the synthesis of β-enaminones or β-aminoacrylates had received only little attention until 2000s. These compounds can be obtained via either addition of ester or amide enolates to nitriles (method A) [12], to tosyl imines (method B) [13], and to imidoyl halides (method C) [14], addition of enamines [15] or ketimines [16] to activated carboxylic acid derivatives (method D) (Scheme 1) or Michael addition of amines to ynones [1,17]. A one-pot sequential process, described by Choudhury et al., consisting of nucleophilic substitution of the lithiated acetylides with Weinreb amides followed by a Michael reaction of the extruded N-methoxy-N-methylamine after quenching with saturated NH4Cl, provided also stereoselectively β-enaminoketones in high yields [18].
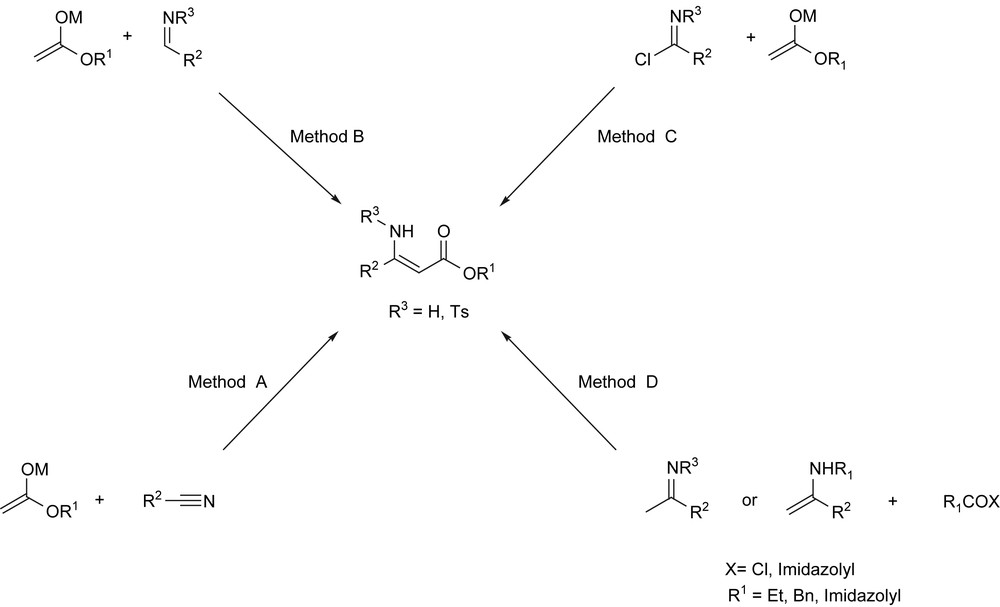
Due to the wide range of utility in pharmaceutical industry, the amination of β-dicarbonyl compounds to form enamine derivatives has become an important transformation, and several methods have been developed for the synthesis of these compounds. One of the most straightforward routes to β-enaminones involves the direct condensation of β-dicarbonyl compounds with amines in refluxing aromatic hydrocarbons with azeotropic removal of water (Scheme 2) [19,20].

Alternatively, primary amines also react with β-dicarbonyl compounds in water to give enaminones [21]. Some improved procedures have been reported using Al2O3 [22], SiO2 [23], K10-montmorillonite [24], NaAuCl4 [25], microwaves [26], iodine [27], [EtNH3][NO3] [28], sulfonated zirconia [29], and orthosilicate in acetic acid [30]. However, many of these methods have some drawbacks: low selectivity (more specifically, transformation of the β-ketoester into β-ketoamide), unsatisfactory yields, harsh conditions. Therefore, due to the importance of these derivatives in organic chemistry, the development of more efficient procedures was desired.
Lewis acid-catalyzed reaction are now of great interest because of their unique reactivity and selectivity under mild conditions [31]. Recently, ZnClO4·6H2O [32], Bi(TFA)3 in water [33a] or molten salt [33b], cerium salts [34,35], CoCl2 [36], ruthenium catalyst [37], ZrCl4 [38], LaCl3·7H2O [39], Yb(OTf)3 [40], Er(OTf)3 [41], and In(OTf)3 [42] were found to be very active for the synthesis of β-enaminones or β-aminoacrylates.
As a part of our research directed toward the preparation of optically active amine compounds via enantioselective hydrogenation [2,43] and cascade transformations catalyzed by Lewis acid [6a], we investigated the use of an efficient Lewis acid catalyst for the synthesis of N-substituted β-aminoacrylates 3 via condensation of primary amines 2 with dicarbonyl derivatives 1 (Scheme 3). We report here that iron(III) chloride is an attractive Lewis acid catalyst for the straightforward synthesis of β-aminoesters under mild conditions.

2 Results and discussion
We have recently shown that Zn(OAc)2·2H2O is a very effective Lewis acid catalyst for the formation of β-enaminoketones and esters from primary amines [44], and that the condensation of secondary amines with β-diketones and β-ketoesters to form β-enaminoketones and esters can be efficiently performed in good to excellent yields under solvent-free conditions in the presence of Lewis acids (such as CeCl3·7H2O, FeCl3·6H2O or Zn(OAc)2·2H2O) [45]. In recent years, iron(III) chloride [46] has emerged as a powerful Lewis acid catalyst and performs many useful organic transformations under mild reaction conditions. Moreover, iron salts are usually non-toxic and very abundant on earth, and consequently among the most inexpensive, easy to handle, and environmentally friendly metal derivatives.
We thus tested the activity of iron salts (5 mol%) for the direct formation of aminoacrylate from benzylamine (1.5 equiv) and methyl acetoacetate (1 equiv) in dichloromethane at room temperature in the presence of magnesium sulfate. The corresponding enamine 3a was isolated in 92% yield after purification by chromatography on a short silica gel column (Scheme 4).

To demonstrate the generality of this method, we next investigated the scope of this reaction under optimized conditions and the results are summarized in Table 1. Thus, a variety of amines (2a–d), including primary, benzylic, and aniline, were condensed with various β-dicarbonyl compounds (1a–e) to produce a range of β-enaminoesters. In general, for primary and benzylic amines or aniline, the condensation reactions usually afforded the corresponding β-enaminoesters in over 80% yield (Table 1). With iso-propylamine, the yield was usually slightly lower (>75%), but remained acceptable.
Iron-catalyzed condensation of amines with β-ketoestersa
Dicarbonyl compound | Amine | Compound | Temperature (°C) | Yield (%) |
1a | 2a | 3a | r.t. | 92 |
1a | 2b | 3b | r.t. | 96 |
1a | 2c | 3c | r.t. | 86 |
1a | 2d | 3d | r.t. | 76 |
1b | 2a | 3e | r.t. | 81 |
1b | 2b | 3f | r.t. | 86 |
1b | 2c | 3g | r.t. | 80 |
1b | 2d | 3h | r.t. | 71 |
1c | 2a | 3i | r.t. | 92 |
1c | 2b | 3j | r.t. | 90 |
1c | 2c | 3k | r.t. | 84 |
1c | 2d | 3l | r.t. | 88 |
1d | 2a | 3m | Reflux | 98 |
1d | 2b | 3n | Reflux | 98 |
1d | 2c | 3o | Reflux | 95 |
1d | 2d | 3p | Reflux | 61 |
1e | 2a | 3q | Reflux | 90 |
1e | 2b | 3r | Reflux | 77 |
a Conditions: 0.75 mmol of amine, 0.5 mmol of ketoester 1, iron(III) chloride (5 mol%) in 5 mL of CH2Cl2.
When 1,2-diaminoethane was used as the amine, 2 equiv of methyl acetoacetate were required to give the product with two enaminoester groups (Scheme 5). Then, compound 3s was isolated in 96% yield as only one stereoisomer.

It is also worth to note that this iron catalyst provided higher yields than our previous zinc acetate catalyst, it provided the enamino derivatives in milder reaction conditions (Table 2), and the reactions could be carried out without MgSO4 as drying reagent [44]. Moreover, it competes with all the more expensive and previously reported Lewis acid catalyst [31–41,43].
Comparison between iron- and zinc-catalyzed condensation of primary amines with β-ketoestersa
Compounds | FeCl3·6H2O (%) | Zn(O2CCH3)2·2H2O/MgSO4 (%) |
92 | 78 | |
98 | 90 | |
90 | 53 | |
86 (r.t.) | 57 (reflux) |
a Conditions: 0.75 mmol of amine, 0.5 mmol of ketoester 1, iron(III) chloride or hydrated zinc(II) acetate (5 mol%)/dried MgSO4 (10 mol%) in 5 mL of CH2Cl2.
The method was chemoselective as amine attacked only at the ketone carbonyl. The (Z)-selectivity in the products derived from acylic diketones and β-ketoesters was secured by intramolecular hydrogen bonding. Thus, by 1H NMR analysis of compound 3, only the signal for CH3 at 1.90 ppm was observed, no signal was obtained at lower field (i.e. >2.2 ppm), and the –NH– group appeared in the high-field region (8.6–10.2 ppm).
The reaction proceeded very cleanly without the formation of any by-products, except water. Because the reaction can be performed using a solvent-free procedure, at the end of the reaction, the crude mixture can be directly eluted over a chromatographic column to obtain the pure product, avoiding any tedious work up.
In conclusion, we have shown that FeCl3·6H2O, an inexpensive and non-toxic iron salt, was able to catalyze condensation of alkyl or aryl amines with β-ketoesters, in good to excellent yields, providing a straightforward and selective route to β-N-substituted aminoacrylate derivatives.
3 Experimental section
1H NMR and 13C NMR spectra were taken on 200 MHz Bruker AC 200 spectrometers. Chemical shifts are reported in ppm referenced to the residual proton resonances of the solvents. Mass spectra (MS) were obtained on GC–MS Hewlett–Packard HP 5971 apparatus. Thin-layer chromatography (TLC) was performed on Merck silica gel 60 F254. Silica gel Merck Geduran SI (40–63 μm) was used for column chromatography.
3.1 General procedure for the preparation of aminoacrylates
To a dichloromethane solution (5 mL/mmol), were added successively (under argon) FeCl3·6H2O (5 mol%), the dicarbonyl derivative 1 (1 equiv), and finally the amine 2. The reaction mixture was stirred at room temperature or under reflux until complete conversion of the ketoester 1 is detected by TLC analysis. The solution was then directly poured at the top of a silica gel column and eluted with a (7/3) heptane/ethyl acetate mixture.
All the N-substituted aminoacrylates were fully characterized with 1H ad 13C NMR. Spectroscopic data of 3c [36], 3d [42], 3g [42], and 3n [36,42,44] have previously been reported and our data were in accordance with those reported.
3.1.1 Methyl 3-(benzylamino)-but-2-enoate (3a)
1H NMR spectrum (200.13 MHz, CDCl3) δ ppm: 1.95 (s, 1H), 3.66 (s, 3H), 4.46 (d, J = 6.4 Hz, 2H), 4.65 (s, 1H), 7.3–7.45 (m, 5H), 8.96 (s, 1H). 13C NMR spectrum (50.3 MHz, CDCl3) δ ppm: 19.8, 47.2, 50.6, 83.2, 127.1 (2C), 127.8, 129.2 (2C), 139.2, 162.4, 171.3. IR (cm−1): 1658, 1600.
HRMS calculated for C12H15NO2: 205.11028, found: 205.1099.
3.1.2 Methyl 3-anilino-but-2-enoate (3b)
1H NMR spectrum (200.13 MHz, CDCl3) δ ppm: 2.09 (s, 3H), 3.71 (s, 3H), 4.70 (s, 1H), 7.09–7.18 (m, 3H), 7.29–7.40 (m, 2H). 13C NMR spectrum (50.3 MHz, CDCl3) δ ppm: 20.8, 50.7, 86.0, 125.0, 125.4, 129.5, 139.7, 159.3, 171.2.
HRMS calculated for C11H13NO2: 191.09463, found: 191.0951.
3.1.3 Methyl 3-(butylamino)-but-2-enoate (3c)
1H NMR spectrum (200.13 MHz, CDCl3) δ ppm: 0.95 (t, J = 7.2 Hz, 3H), 1.47 (m, 2H), 1.58 (m, 2H), 1.93 (s, 3H), 3.22 (q, J = 6.5 Hz, 2H), 3.64 (s, 3H), 4.45 (s, 1H). 13C NMR spectrum (50.3 MHz, CDCl3) δ ppm: 14.2, 19.8, 20.4, 32.9, 43.1, 50.3, 81.7, 162.6, 171.4.
3.1.4 Methyl 3-(iso-propylamino)-but-2-enoate (3d)
1H NMR spectrum (200.13 MHz, CDCl3) δ ppm: 1.22 (d, J = 6.4 Hz, 6H), 1.96 (s, 3H), 3.63 (s, 3H), 3.71 (m, 1H), 4.41 (s, 1H), 8.52 (s, 1H). 13C NMR spectrum (50.3 MHz, CDCl3) δ ppm: 19.6, 24.5, 44.9, 50.3, 81.8, 161.4, 171.3.
3.1.5 Ethyl 3-(benzylamino)-but-2-enoate (3e)
1H NMR spectrum (200.13 MHz, CDCl3) δ ppm: 1.28 (t, J = 7.1 Hz, 3H), 1.94 (s, 3H), 4.12 (q, J = 7.1 Hz, 2H), 4.45 (d, J = 6.4 Hz, 2H), 4.55 (s, 1H), 7.27–7.36 (m, 5H), 8.97 (s, 1H). 13C NMR spectrum (50.3 MHz, CDCl3) δ ppm: 15.1, 19.8, 47.2, 58.8, 83.6, 127.1, 127.8, 129.2, 139.3, 162.3, 171.0.
HRMS calculated for C13H17NO2: 219.12593, found: 219.1244.
3.1.6 Ethyl 3-anilino-but-2-enoate (3f)
1H NMR spectrum (200.13 MHz, CDCl3) δ ppm: 1.21 (t, 3H, J = 6.4 Hz), 1.99 (s, 3H), 4.10 (q, 2H, J = 6.4 Hz), 4.78 (s, 1H), 7.10 (m, 5H), 10.35 (s, 1H, NH). 13C NMR spectrum (50.3 MHz, CDCl3) δ ppm: 14.6, 20.4, 58.7, 86.1, 124.4 (2C), 124.9, 129.3 (2C), 139.4, 159.0, 170.4.
HRMS calculated for C12H15NO2: 205.11028, found: 205.1118.
3.1.7 Ethyl 3-(butylamino)-but-2-enoate (3g)
1H NMR spectrum (200.13 MHz, CDCl3) δ ppm: 0.95 (t, J = 7.1 Hz, 3H), 1.26 (t, J = 7.1 Hz, 3H), 1.53 (m, 4H), 1.93 (s, 1H), 3.22 (q, J = 6.5 Hz, 2H), 4.10 (q, J = 7.1 Hz, 2H), 4.44 (s, 1H), 8.55 (s, 1H). 13C NMR spectrum (50.3 MHz, CDCl3) δ ppm: 14.1, 15.0, 19.7, 20.4, 32.8, 43.1, 58.6, 82.1, 162.3, 171.0.
3.1.8 Ethyl 3-(iso-propylamino)-but-2-enoate (3h)
1H NMR spectrum (200.13 MHz, CDCl3) δ ppm: 1.22 (d, J = 6.4 Hz, 6H), 1.28 (t, J = 7.2 Hz, 3H), 1.95 (s, 3H), 3.67 (m, 1H), 4.10 (q, J = 7.1 Hz, 2H), 4.40 (s, 1H), 8.47 (s, 1H). 13C NMR spectrum (50.3 MHz, CDCl3) δ ppm: 15.12, 19.67, 24.56, 44.88, 58.63, 82.12, 161.36, 171.38.
3.1.9 tert-Butyl 3-(benzylamino)-but-2-enoate (3i)
1H NMR spectrum (200.13 MHz, CDCl3) δ ppm: 1.50 (s, 9H), 1.90 (s, 3H), 4.45 (d, J = 6.6 Hz, 2H), 4.93 (s, 1H), 7.24–7.39 (m, 5H), 8.92 (m, 1H). 13C NMR spectrum (50.3 MHz, CDCl3) δ ppm: 19.8, 29.1, 47.2, 78.4, 85.3, 127.2, 127.7, 129.2, 139.5, 161.0, 171.1.
HRMS calculated for C15H21NO2: 247.15723, found: 247.1577.
3.1.10 tert-Butyl 3-anilino-but-2-enoate (3j)
1H NMR spectrum (200.13 MHz, CDCl3) δ ppm: 1.56 (s, 9H), 2.00 (s, 3H), 4.70 (s, 1H), 7.08–7.37 (m, 5H), 10.45 (s, 1H). 13C NMR spectrum (50.3 MHz, CDCl3) δ ppm: 20.7, 29.1, 78.9, 88.4, 124.6, 125.1, 129.4, 140.0, 158.4, 170.8.
HRMS calculated for C14H19NO2: 233.14158, found: 233.1407.
3.1.11 tert-Butyl 3-(butylamino)-but-2-enoate (3k)
1H NMR spectrum (200.13 MHz, CDCl3) δ ppm: 0.94 (t, J = 7.2 Hz, 3H), 1.47 (s, 9H), 1.49 (m, 4H), 1.89 (s, 3H), 3.18 (q, J = 6.7 Hz, 2H), 4.37 (s, 1H), 8.47 (s, 1H). 13C NMR spectrum (50.3 MHz, CDCl3) δ ppm: 14.2, 19.8, 20.5, 29.1, 33.1, 46.2, 78.1, 83.8, 161.7, 171.2.
3.1.12 tert-Butyl 3-(iso-propylamino)-but-2-enoate (3l)
1H NMR spectrum (200.13 MHz, CDCl3) δ ppm: 1.21 (d, J = 6.4 Hz, 6H), 1.47 (s, 9H), 1.91 (s, 3H), 3.66 (m, 1H), 4.33 (s, 1H), 8.40 (s, 1H). 13C NMR spectrum (50.3 MHz, CDCl3) δ ppm: 19.5, 24.5, 29.1, 44.7, 77.9, 83.9, 160.3, 170.95.
3.1.13 Ethyl 2-benzylamino-cyclopent-1-ene-1-carboxylate (3m)
1H NMR spectrum (200.13 MHz, CDCl3) δ ppm: 1.26 (t, 3H, J = 7.1 Hz), 1.78 (quint, 2H, J = 7.7 Hz), 2.53 (m, 4H), 4.15 (q, 2H, J = 7.1 Hz), 4.35 (d, 2H, J = 6.3 Hz), 7.15–7.40 (m, 5H), 7.84 (br s, 1H (NH)). 13C NMR spectrum (50.3 MHz, CDCl3) δ ppm: 14.4, 20.5, 28.8, 31.6, 47.9, 53.2, 93.0, 126.3 (2C), 126.8, 128.3 (2C), 138.9, 164.1, 168.0.
HRMS calculated for C15H19NO2: 245.14158, found: 245.1409.
3.1.14 Ethyl 2-anilino-cyclopent-1-ene-1-carboxylate (3n)
1H NMR spectrum (200.13 MHz, CDCl3) δ ppm: 1.34 (t, J = 7.1 Hz, 3H), 1.90 (m, 2H), 2.6 (t, J = 7.2 Hz, 2H), 2.83 (t, J = 7.5 Hz, 2H), 4.25 (q, J = 7.1 Hz, 2H), 7.02–7.34 (m, 5H), 9.62 (s, 1H). 13C NMR spectrum (50.3 MHz, CDCl3) δ ppm: 15.2, 22.3, 29.2, 34.1, 59.4, 98.1, 121.1, 123.5, 129.6, 141.2, 160.9, 168.9.
3.1.15 Ethyl 2-(butylamino)-cyclopent-1-ene-1-carboxylate (3o)
1H NMR spectrum (200.13 MHz, CDCl3) δ ppm: 0.94 (t, J = 7 Hz, 3H), 1.28 (t, J = 7.1 Hz, 3H), 1.37–1.57 (m, 4H), 1.83 (m, 2H), 2.56 (t, J = 7 Hz, 4H), 3.20 (q, J = 6.5 Hz, 2H), 4.16 (q, J = 7.1 Hz, 2H), 7.41 (s, 1H). 13C NMR spectrum (50.3 MHz, CDCl3) δ ppm: 14.0, 14.3, 15.2, 20.3, 29.4, 32.4, 33.4, 44.8, 58.8, 92.5, 165.4, 169.0.
3.1.16 Ethyl 3-(iso-propylamino)-cyclopent-1-ene-1-carboxylate (3p)
1H NMR spectrum (200.13 MHz, CDCl3) δ ppm: 1.20 (d, J = 6.4 Hz, 6H), 1.28 (t, J = 7.1 Hz, 3H), 1.83 (m, 2H), 2.55 (m, 4H), 3.57 (m, 1H), 4.12 (q, J = 7.1 Hz, 2H), 7.37 (s, 1H). 13C NMR spectrum (50.3 MHz, CDCl3) δ ppm: 15.2, 21.7, 24.8, 29.3, 32.3, 46.7, 58.8, 92.4, 164.4, 169.0.
3.1.17 Ethyl 3-(benzylamino)-3-phenyl-acrylate (3q)
1H NMR spectrum (200.13 MHz, CDCl3) δ ppm: 1.28 (t, 3H, J = 7.1), 4.21 (q, 2H, J = 7.1 Hz), 4.30 (d, 2H, J = 6.5 Hz), 4.70 (s, 1H), 7.29 (m, 10H), 8.91 (br s, 1H, NH). 13C NMR spectrum (50.3 MHz, CDCl3) δ ppm: 14.5, 48.3, 58.8, 86.2, 126.8 (2C), 127.1, 127.8 (2C), 128.3 (2C), 129.0 (2C), 129.2, 136.0, 139.2, 164.7, 170.3.
HRMS calculated for C18H19NO2: 281.14158, found: 281.1408.
3.1.18 Ethyl 3-(anilino)-3-phenyl-acrylate (3r)
1H NMR spectrum (200.13 MHz, CDCl3) δ ppm: 1.35 (t, J = 7.2 Hz, 3H), 4.26 (q, J = 7.1 Hz, 2H), 5.03 (s, 1H), 6.69 (d, J = 7.8 Hz, 2H), 6.90–6.97 (m, 1H), 7.11 (t, J = 7.6 Hz, 2H), 7.27–7.40 (m, 5H). 13C NMR spectrum (50.3 MHz, CDCl3) δ ppm: 15.0, 59.8, 91.6, 122.6, 123.4, 128.7, 128.9, 129.1, 129.9, 136.4, 140.8, 159.5, 170.6.
HRMS calculated for C17H17NO2: 267.12593, found: 267.1259.
3.1.19 (2Z,2′Z)-Dimethyl 3,3′-(ethane-1,2-diylbis(azanediyl))-dibut-2-enoate (3s)
1H NMR spectrum (200.13 MHz, CDCl3) δ ppm: 1.93 (s, 6H), 3.36 (q, J = 6.2 Hz, 4H), 3.63 (s, 6H), 4.51 (s, 2H), 8.64 (s, 1H). 13C NMR spectrum (50.3 MHz, CDCl3) δ ppm: 19.7, 44.2, 50.5, 83.6, 161.8, 171.3.
HRMS calculated for C12H20N2O4: 256.14231, found: 256.1420.
Acknowledgements
The authors wish to warmly acknowledge the Ministry of Education and Research of the Algerian government for a doctoral grant to H.H.