1 Introduction
Rare-earth half-sandwich complexes are known as highly versatile polymerization precatalysts or catalysts. A Cp∗La[CH(SiMe3)2]2 (Cp∗ = C5Me5) half-lanthanocene was reported for the polymerization of various polar and non-polar monomers in a controlled fashion [1]. Cationic systems based on a (C5Me4SiMe3)Sc(CH2SiMe3)2(THF) half-scandocene were reported for the polymerization and copolymerization of various non-polar monomers, leading notably to unprecedented styrene–ethylene copolymers that incorporate syndiotactic sequences [2]. Another example is given by a Cp∗Nd(BH4)2(THF)2 borohydrido half-neodymocene that leads, after an in situ alkylation, to new stereoregular block [3] and statistical [4] isoprene–styrene copolymers and to the unprecedented polystyrene catalyzed chain growth on a metal [3]. Despite such promising potentialities, polymerization catalysis using mono(cyclopentadienyl) complexes of the rare earths remains poorly explored in comparison to their metallocene homologues, probably due to the difficulties encountered in the synthesis of these compounds. Indeed, rare-earth half-sandwich complexes are generally considered as poorly stable, electronically more unsaturated and sterically opened in comparison with lanthanidocenes, and as a consequence, elaborated synthetic routes have to be used [5]. We recently developed a simple and convenient route toward the formation of borohydrido half-lanthanidocenes, where the cyclopentadienyl ligand is introduced from its neutral protonated form using an alkylmagnesium reagent associated with a trisborohydride precursor [6]. On the one hand, the so-called “borohydride/alkyl route” led è to a new family of bimetallic ionic compounds of the general formula [(CpR)Ln(BH4)3]2[Mg(THF)6] that could be isolated using a one-pot synthesis (Scheme 1, [7]). Combined with dialkylmagnesium, their application to the polymerization of isoprene reveals a highly stereoselective reaction (Scheme 2).

B/A synthesis of the ionic compound.

Polymerization involving the ionic compound obtained by the B/A route (I = isoprene).
We performed on the other hand the polymerization using an in situ generated monoCp neodymium active species by the B/A route in the presence of isoprene and using an additional equivalent of dialkylmagnesium for the alkylation step (Scheme 3). The resulting catalytic properties were similar not only to those observed starting from the isolated bimetallic complex 1a, but also to those starting from the neutral homologue Cp∗Nd(BH4)2(THF)2 (Scheme 4, [6]) obtained by ionic metathesis. This in situ one-pot method enables us to generate very easily sophisticated catalytic systems that are obtained after several synthetic steps, and to evaluate the influence of specific ligands on the catalytic behaviour of half-lanthanidocenes in a simple and convenient way. We report in this frame, after a brief extension to the polymerization of isoprene using lanthanum based half-sandwiches, a similar strategy applied to the polymerization of styrene. Reactions performed via this B/A route with in situ introduction of the ligand starting from HCp∗, HCp and ligands are compared to the polymerization mediated by the complexes 1a, 1b, 2a and 3a. It is shown that the former method not only enables to evaluate the effect of a ligand on the catalytic properties in a simple way, but also leads to a well-controlled polymerization of styrene starting from a common trisborohydride precursor combined with commercial ligands and dialkylmagnesium compound.
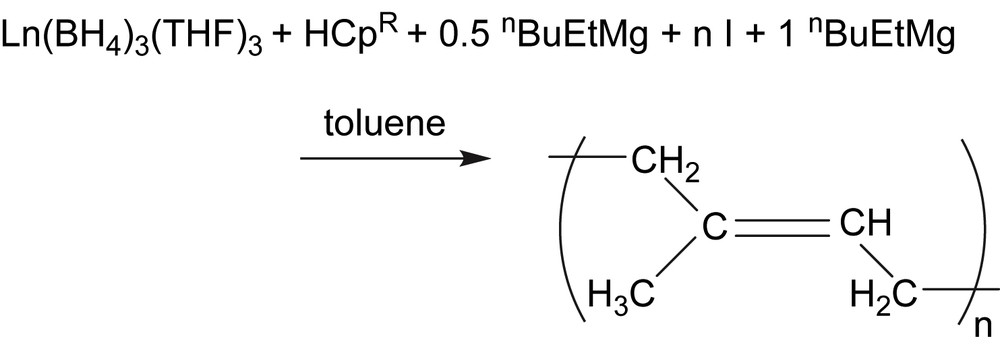
Polymerization by B/A in situ route (I = isoprene).

“Regular” poylmerization starting from the neutral half-lanthanidocene (I = isoprene).
2 Half-lanthanocenes for the polymerization of isoprene
The polymerization of isoprene using complex [(Cp∗)La(BH4)3]2[Mg(THF)6] 1b combined with n-butylethylmagnesium is reported in Table 1, together with the results obtained according to the B/A in situ monoCp generation depicted in Scheme 3. Both entries can be compared to the polymerization of isoprene using the trisborohydride precursor La(BH4)3(THF)3 4b combined with n-butylethylmagnesium [8]. The introduction of the pentamethylcyclopentadienyl ligand using the B/A routes leads to (i) a significant increase of the activity, from 1 to 20 kg/mol/h, (ii) an increase of the trans-selectivity of the reaction, from ca. 93 to 97% and (iii) narrower molecular weight distributions (entries 1 and 2 vs. [8]). Both routes involving a monoCp compound lead to similar activity and selectivity, following the trends observed for neodymium-based compounds [6]. The B/A in situ route is thus a convenient tool to evaluate the role played by the ligand, even if the resulting molecular weights are slightly less controlled (PDI = 1.63 vs. 1.39 for entries 2 and 1, respectively).
Polymerization of isoprene using the lanthanum bimetallic ionic precatalyst 1b and comparison with the in situ generation of the active species
Entry | Precatalyst | Time (h) | Yield (%) | Activity (kg/mol/h) | c (g/mol) | d (g/mol) | PDIe | 1,4-transf (%) | 1,4-cisf (%) | 3,4-f (%) |
1a | 1b | 2 | 60 | 20.4 | 40,800 | 43,100 | 1.39 | 97.6 | 0.5 | 1.9 |
2b | (In situ) | 2 | 58 | 19.6 | 39,400 | 25,300 | 1.63 | 96.7 | 0.3 | 3 |
[8] | 4b | 24 | 25 | 0.7 | 17,000 | 17,300 | 1.84 | 92.8 | 5.3 | 1.9 |
a Reactions conducted at 50 °C with 10−6 mol of 1b or 4b, 1 equiv n-butylethylmagnesium for the alkylation and a [Isoprene]/[La] ratio of 1000 in 1 mL toluene (I = isoprene).
b Reactions conducted at 50 °C with 10−6 mol of 4b, 1 equiv HCp∗, 1.5 equiv n-butylethylmagnesium and a [Isoprene]/[La] ratio of 1000 in 1 mL toluene (Scheme 3).
c Expected molecular weight assuming one growing chain per lanthanum: .
d Number-average molecular weight measured by SEC.
e Polydispersity index measured by SEC .
f Determined by 1H NMR.
3 Half-lanthanidocenes for the polymerization of styrene
3.1 Polymerization starting from ionic bimetallic precatalysts
The polymerization of styrene using complexes 1a, 1b, 2a, and 3a combined with n-butylethylmagnesium according to Scheme 2 is reported in Table 2. All these systems are able to oligomerize/polymerize styrene at 50 °C. The introduction of the pentamethylcyclopentadienyl ligand using this B/A route leads to (i) a decrease in activity and (ii) narrower molecular weight distributions as compared to the trisborohydride precursors Ln(BH4)3(THF)3 (Ln = Nd, 4a and Ln = La, 4b) in the presence of 1 equiv dialkylmagnesium. For neodymium-based catalysts, molecular weights are smaller than expected considering two growing chains per magnesium atom,1 highlighting possible uncontrolled transfer reactions. If the polydispersity is acceptable (PDI < 1.3) in the first step of the polymerization, the molecular weight broadens along the polymerization for higher St/Ln ratios, probably as a consequence of the advanced transfer reactions. The lanthanum-based system leads in turn to a controlled polymerization of styrene independent of the St/Ln ratio (PDI = 1.22, entries 5 and 6 in Table 2) with an activity similar to that of its neodymium homologue (entries 3 and 4 in Table 2). Concerning the ligand effect, a slightly higher activity after 2 h (entries 7 and 9 vs. 3 in Table 2) can be observed using Cp and vs. Cp∗, which suggests an influence of the electron-donating ability rather than steric requirements. 13C NMR spectra in the phenyl ipso carbon region were found to be in accordance with a syndiotactic-rich polystyrene,2 whatever the nature of the ligand. A representative pattern is given in Fig. 1e.
3.2 Polymerization following the B/A in situ pathway
The polymerization of styrene according to Scheme 3 starting from HCp∗, HCp and is reported in Table 3. From the value of the polydispersity indexes (PDI ≤ 1.25 except for for a St/Nd ratio of 440), all these systems are able to oligomerize/polymerize styrene with a well controlled character at 50 °C. The introduction of a cyclopentadienyl ligand, particularly HCp∗ using the “borohydride/alkyl” in situ route leads to (i) a decrease in activity and (ii) narrower molecular weight distributions as compared to the trisborohydride precursors 4a and 4b. It is noteworthy that the PDI remains in the controlled range for higher St/Nd ratio and longer polymerization times (except for ) as opposed to the broadening observed starting from the ionic precursor. The reaction leads moreover to a syndiotactic-rich polystyrene, whatever the nature of the ligand. A representative pattern is given in Fig. 1d. A higher activity after 2 h is observed using HCp and vs. HCp∗, which confirms the trends observed previously. From entry 16, consistent correspondence between experimental and calculated number-average molecular weight is observed with HCp combined with neodymium trisborohydride.
Polymerization of styrene – B/A in situ generation of monoCp complexes
Entrya | Ln | Ligand | St/Ln | Time (h) | Yield (%) | Activity (kg/mol/h) | b (g/mol) | c (g/mol) | PDId |
11 | Nd | HCp∗ | 100 | 2 | 19 | 1 | 1000 | 470 | 1.19 |
[10] | Nd | – | 100 | 2 | 58 | 3.0 | 3000 | 2700 | 1.7 |
12 | Nd | HCp∗ | 440 | 20 | 27 | 0.6 | 6400 | 8900 | 1.14 |
13 | La | HCp∗ | 100 | 2 | 20 | 1 | 1000 | 500 | 1.19 |
[10] | La | – | 100 | 2 | 78 | 4.0 | 4100 | 6000 | 1.7 |
14 | La | HCp∗ | 433 | 20 | 22 | 0.5 | 5000 | 7700 | 1.09 |
15 | Nd | HCp | 100 | 2 | 33 | 1.7 | 1700 | 1000 | 1.20 |
16 | Nd | HCp | 440 | 20 | 68 | 1.6 | 15,500 | 15,700 | 1.25 |
17 | Nd | 100 | 2 | 26 | 1.4 | 1400 | 800 | 1.16 | |
18 | Nd | 440 | 20 | 37 | 0.8 | 8500 | 3750 | 1.75 |
a Reactions conducted at 50 °C with 0.5 mL styrene in 0.5 mL toluene in combination with n-butylethylmagnesium.
b Expected molecular weight assuming two growing chains per magnesium atom: .
c Number-average molecular weight measured by SEC.
d Polydispersity index measured by SEC .
3.3 Comparison with the neutral precatalyst
The polymerization of styrene using the neutral compound Cp∗Nd(BH4)2(THF)2 [3] vs. the ones obtained from the B/A route (ionic and in situ introduction of the ligand) is reported in Table 4. The activities observed are higher and the control over the molecular weight is better using the neutral half-neodymocene. The number-average molecular weights are closer to the theoretical ones considering two growing chains per magnesium atom. In contrast to the pathway involving the ionic bimetallic precatalyst, and in a similar way to the B/A in situ route, the polydispersity remains constant along the polymerization and for higher St/Nd ratios. The selectivity is higher using the neutral compound, as it is possible to quantitate the syndiotacticity (around 85%) from the heptads observed on the 13C NMR pattern (Fig. 1c, [3]).
Comparison between B/A generated and neutral half-neodymocene precatalysts for the polymerization of styrene
Entrya | Route | St/Nd | Time (h) | Conversion (%) | Activity (kg/mol/h) | b (g/mol) | c (g/mol) | PDId |
3 | Ionic | 100 | 2 | 24 | 1.2 | 1200 | 650 | 1.14 |
11 | (In situ) | 100 | 2 | 19 | 1 | 1000 | 470 | 1.19 |
[3] | Neutral | 100 | 2 | 31 | 1.6 | 1600 | 1400 | 1.32 |
4 | Ionic | 429 | 20 | 45 | 1.0 | 10,000 | 7200 | 1.81 |
12 | (In situ) | 440 | 20 | 27 | 0.6 | 6400 | 8900 | 1.14 |
[3] | Neutral | 440 | 20 | 81 | 1.9 | 18,500 | 16,000 | 1.22 |
a Reactions conducted at 50 °C with 0.5 mL styrene in 0.5 mL toluene in combination with one equivalent n-butylethylmagnesium.
b Expected molecular weight assuming two growing chains per magnesium atom: .
c Number-average molecular weight measured by SEC.
d Polydispersity index measured by SEC .
4 Discussion
The extension of the B/A strategy, initially developed for the neodymium-mediated polymerization of isoprene, to the lanthanum-based polymerization of isoprene has been carried out successfully. The B/A approach has been further applied to the half-lanthanidocene-mediated polymerization of styrene. Trends for activity and selectivity obtained using the in situ strategy were found to be in agreement with those observed starting from the isolated ionic half-lanthanide complexes; similar activities for Nd- vs. La-based catalytic systems, a smaller activity for HCp∗ vs. HCp and , and syndiotactic-rich polystyrenes. This confirms the usefulness of such an approach for evaluating the influence of a ligand over the catalytic properties. The B/A in situ route leads moreover to narrow molecular weight distributions (1.09 < PDI < 1.25 except for and a St/Nd ratio of 440); one can thus consider this strategy as a very simple way to polymerize styrene in a controlled fashion starting from a common trisborohydride precursor and a commercial ligand. Such behaviour is, however, not observed starting from the neodymium-based ionic precursors 1a, 2a and 3a, where a broadening is observed for higher St/Nd ratios and longer times. Such a broadening is, interestingly, not observed with the lanthanum compound 1b.
The similar behaviour of the routes involving neutral or ionic monoCp precatalysts observed in the course of the neodymium-mediated polymerization of isoprene was explained on the basis of a dissociation of the ionic complex releasing a BH4− group, leading to the same active species as that of the neutral compound [6]. The possible steps leading to this active species involving two borohydrido groups per metal atom are represented in Scheme 5. Such a dissociation of the ionic form that occurs in the presence of n-butylethylmagnesium and isoprene would thus not occur in the presence of the bulky and less coordinating styrene in the case of neodymium based catalysts since the results are different (vide infra). As a consequence, the active species may have the form of a crowded complex involving three BH4 per metal analogue to (I) in Scheme 5, leading to a poorer control over the molecular weight along the polymerization. A “putative active species” in the presence of an additional [Mg]B ligand may also be considered. A possible explanation is that such active species simply favours an uncontrolled transfer reaction as compared to the “neutral” active species.

Dissociation of the ionic precatalysts after addition of (i) 1 equiv dialkylmagnesium and (ii) isoprene in excess. B represents a BH4 group, and THF molecules are omitted for clarity.
The weak Lewis acidity of the lanthanum element may in turn render this dissociation possible in a styrene surrounding, and lead to an active species that enables a good control of the polymerization.3 The role played by electron density and charge delocalization in lanthanide-mediated polymerizations has been discussed in the case of dienes [11], and notably for neodymium–magnesium bimetallic active species [12].
The smaller activity and selectivity observed for neodymium based B/A approaches vs. the neutral one may be related to the presence of higher quantities of THF in the medium [6], and possibly to the additional BH4− group in the coordination sphere of the metal. This BH4− group may increase the electron density, which would result in a more difficult coordination of styrene.
The results from this study act finally toward the existence of different active species for the polymerization of styrene following the different routes, in contrast to isoprene. This can be related to previous findings showing important differences between the polymerization of isoprene and that of styrene using half-lanthanidocenes combined with dialkylmagnesium: two growing chains per magnesium atom are observed for styrene using Cp∗Nd(BH4)2(THF)2 combined with n-butylethylmagnesium [3], although only one was evidenced in the case of isoprene [8]. Fast and reversible transfer reactions could moreover been achieved in the case of styrene [3], while an excess of magnesium decreases the activity and broadens the molecular weight distribution of the resulting polyisoprene [8].
5 Conclusion
The “borohydride/alkyl” route has been applied successfully to the polymerization of styrene. When the ligand is introduced in situ, this method provides an interesting tool for the assessment of the influence of a ligand on the performance of half-lanthanidocene catalysts. All the systems tested via this approach lead to well-controlled oligomerization/polymerization processes (PDI < 1.2 in most cases). This highlights a simple way to polymerise styrene in a controlled fashion starting from a common trisborohydride precursor combined with a commercial ligand HCpR. The influence of the nature of a ligand on the activity of borohydrido-based catalytic systems could be established, with trends corresponding to those obtained starting from the isolated ionic precursors [(CpR)Ln(BH4)3]2[Mg(THF)6]: . These results suggest an influence of the electron donating ability rather than steric requirements.
6 Experimental section
6.1 Materials
All operations were performed under dry argon using Schlenk techniques. Toluene was dried over sodium/benzophenone and distilled over molecular sieves before use. Styrene and isoprene (99% from Aldrich) were dried over calcium hydride and distilled over molecular sieves. n-Butylethylmagnesium (from Texas Alkyl) and di-n-hexylmagnesium (from Akzo Nobel) were both used as 20 wt.% solutions in heptane. Ln(BH4)3(THF)3 [13] and [(CpR)Ln(BH4)3]2[Mg(THF)6] [7] complexes were synthesized according to literature procedures.
6.2 Polymerisation
6.2.1 Ionic precatalysts
In a glove box, the borohydrido complex was weighed into a vessel. Toluene, styrene and n-butylethylmagnesium were added in the flask, respectively, using syringes. The mixture was magnetically stirred at the reaction temperature for a given time, diluted in toluene and the resulting solution was poured into ethanol, leading to the precipitation of an off-white polymer. Solvents were evaporated under partial vacuum, and the product was dried under vacuum for 48 h.
6.2.2 In situ introduction of the ligand
In a glove box, the borohydrido complex was weighed in a vessel. Ligand, 1/2 equiv of n-butylethylmagnesium, toluene, styrene and 1 equiv of n-butylethylmagnesium were added in the flask in this order using syringes. The procedure described above was employed.
6.3 Measurements
1H spectrum of polyisoprene was recorded on a AC 300 Bruker spectrometer at room temperature in CDCl3. 13C NMR spectra of the polystyrene were recorded on a AC 400 Bruker spectrometer at 380 K and 400 K in tetrachloroethane-d2. The chemical shifts were calibrated using the residual resonances of the solvent. Size exclusion chromatography (SEC) of polystyrenes was performed (i) in THF as eluent at 20 °C using a Waters SIS HPLC-pump, a Waters 410 refractometer and Waters styragel column (HR2, HR3, HR4, HR5E) for soluble low-molecular-weight oligomers resulting from polymerizations conducted with a St/Ln ratio of 100 and (ii) in trichlorobenzene as eluent at 135 °C using a Waters 150C PLUS device with coupled refractometer and viscosity detectors for the other experiments. The number-average molecular weight and polydispersity index were calculated using polystyrene standards.
Acknowledgments
We are very grateful to the Région Nord-Pas-de-Calais (ARCIR Nanocat project). We are also indebted to Marc Bria and Pierre Watkin for NMR studies, Anne-Marie Cazé for GPC measurements and Arnaud Caillier for careful reading of the manuscript.
1 Molecular weight distributions resulting from the polymerization of styrene using the neutral Cp∗Nd(BH4)2(THF)2 compound combined with n-butylethylmagnesium are consistent with the growth of one macromolecular chain per alkyl group, see Ref. [3].
Entrya | Complex (Ln, ligand) | St/Ln | Time (h) | Yield (%) | Activity (kg/mol/h) | b (g/mol) | c (g/mol) | PDId |
3 | 1a (Nd, Cp∗) | 100 | 2 | 24 | 1.2 | 1200 | 650 | 1.14 |
[10] | 4a | 100 | 2 | 58 | 3.0 | 3000 | 2700 | 1.7 |
4 | 1a (Nd, Cp∗) | 429 | 20 | 45 | 1.0 | 10,000 | 7200 | 1.81 |
5 | 1b (La, Cp∗) | 100 | 2 | 18 | 0.9 | 900 | 640 | 1.22 |
[10] | 4b | 100 | 2 | 78 | 4.0 | 4100 | 6000 | 1.7 |
6 | 1b (La, Cp∗) | 440 | 20 | 45 | 1.0 | 10,300 | 13,100 | 1.22 |
7 | 2a (Nd, Cp) | 100 | 2 | 26 | 1.4 | 1400 | 720 | 1.26 |
8 | 2a (Nd, Cp) | 456 | 20 | 42 | 1.0 | 10,000 | 3500 | 2.28 |
9 | 3a | 100 | 2 | 30 | 1.6 | 1600 | 730 | 1.32 |
10 | 3a | 435 | 20 | 39 | 0.9 | 8800 | 3400 | 2.52 |
a Reactions conducted at 50 °C with 0.5 mL styrene in 0.5 mL toluene in combination with n-butylethylmagnesium.
b Expected molecular weight assuming two growing chains per magnesium atom: .
c Number-average molecular weight measured by SEC.
d Polydispersity index measured by SEC .
2 From 13C NMR spectra (Fig. 1), the syndiotacticity of the corresponding polymers did not enable to distinguish the four heptads characteristics of highly syndiotactic polystyrene [9] and to perform a quantitative analysis.
3 The exact nature of the active species involved in the half-lanthanidocene/dialkylmagnesium-mediated polymerization of styrene is still the subject of investigation in our group.