1 Introduction
Single-Molecule Device is the ultimate frontier of Nanosciences (until the jump to single atom device), but it requires the need to “hold” the molecule in a reproducible way. Self-assembly has been proposed as a principle to control the order at the molecular scale, and Self-Assembled Monolayer (SAM) was found as an elegant way to orient as well as to address electrically a molecular component. The ever-growing field of SAM covers a large range of topics in surface sciences, from the simple modification of surface properties, the introduction of some specific interaction to the control of electron exchanges between a solution and an electrode, with applications such as surface patterning, cell culture, sensors… [1]. They have also been proposed as a way to study molecular wires, keeping in mind that, since self-assembly is not unimolecular intrinsically, one can doubt of “how much” the results obtained are significant of the behaviour of a single molecule (tightly bound in the SAM's structure).
Following the pioneering work of Creager's [2–6] and Chidsey's teams [7,8], we have attempted to use redox active SAM in order to screen candidate structures for molecular wires, taking advantage of the quantum description of the heterogeneous electron transfer (HET) rate constant. Indeed, the rate constant of this elementary act being derived from the Fermi's Golden rule (case of weakly interacting redox sites with a metallic electrode), one can find, in the pre-exponential factor, a coupling matrix element describing the tunnelling interaction of the localized redox site with the electrode through what separates them. Thus, if a molecular wire is used to graft the redox centre to an electrode, one should be able to extract from the HET rate constant its transparency to tunnelling electrons [2,9,10]. If one omit the synthesis of the molecular wires in itself, the main difficulty of such a study lies in the preparation of the SAM, since a monokinetic response (i.e. a homogeneous spreading) of the diluted component is sought. Furthermore, the electrochemical technique imposes a minimum signal-to-noise ratio (i.e. a sufficient amount of electroactive species), and is limited by the electrochemical cell's time constant, which depends on the working electrode's area. Thus some groups are deriving results from pure monolayers, made of the electroactive component only [11].
Most of the data collected on conjugated molecular wires are based on ferrocene- [2,7–9,12] or organic donor- [13,14] containing compounds. In the past we have compared the relative kinetic properties of an alkane thiol carrying a cyclometallated ruthenium complex [Ru(bpy)2(ppR)]+ (bpy: 2,2′-bipyridine; ppX: 2-(2′-pyridyl)-4-R-phen-1-yl, R: NHC(O)(CH2)10SH) to published data on an analogous ferrocene containing alkane thiol [15]. The aim was to check the possibility of using such a ruthenium redox centre, the chemistry of which is rather rich, as an alternative to ferrocene. We have been able to show that its cationic charge improves the solubility of rigid molecular wires [16].
In the present study we wish to report our observations about the electrochemical response of mixed ternary monolayers, containing the ferrocenylketoalkanethiol FcC(O)(CH2)10SH and the ruthenated complex [Ru(bpy)2(pp-NHC(O)(CH2)10SH)]+, diluted in ω-hydroxyundecanethiol and adsorbed on a gold ball electrode (Scheme 1), by impedance spectroscopy.
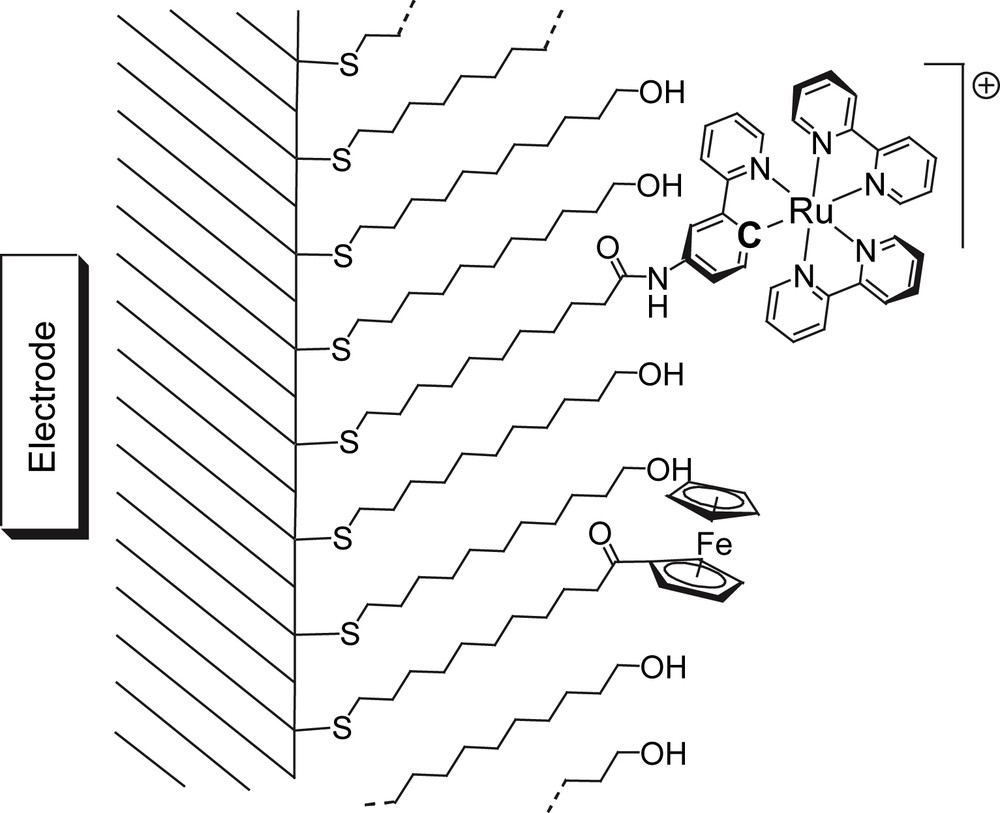
A pictorial view of a self-assembled monolayer made of ω-mercaptoundecanol and containing the ferrocene and the ruthenated thiol derivatives.
2 Experimental
2.1 Set-up
Electrochemical experiments were performed under argon using a single-compartment cell (ca. 4 mL of electrolyte solution). The supporting electrolyte was a 1 M aqueous NaClO4. The cell was housed in a homemade Faraday cage to reduce stray electrical noise. Measurements were performed in a three-electrode mode with an Ag/AgCl/KCl reference electrode (Radiometer-Sodimel S.A.) and a Pt wire auxiliary electrode. The working electrode was a gold ball obtained by melting the end of a clean gold wire with an O2/C2H2 flame into a ca. 500 μm in diameter ball. (Diameter was measured by means of a binocular magnifying glass.) Once covered with the SAM, of the working electrode was positioned as close as possible from the reference electrode with only the ball part immersed in the electrolyte solution. Acquisition of electrochemical data was done using an AUTOLAB PGSTAT 100 potentiostat/frequency response analyser system, under computer control by FRA2 software package (ECO CHEMIE BV). The ac voltammograms were recorded in a stepped mode, one point being acquired every 10 mV, using a rms amplitude (EAC) of 10 mV.
2.2 SAM preparation
Stock solutions of electroactive species (0.1 mM) and ω-mercaptoundecanol (1 mM) were prepared in ethanol. Coating mixtures, obtained by mixing appropriate volumes of the three mother solutions so as to get a final volume of 1–2 mL, were degassed by Ar-bubbling for 15 mn. SAM was obtained by immersing the freshly melted gold ball electrode in the coating solution for 15 min, then chemically annealed in a 1 mM degassed ethanolic solution of ω-mercaptoundecanol for various times. The prepared electrodes were then briefly rinsed with ethanol and connected to the electrochemical cell as described above.
2.3 Chemicals
Ruthenium compound was available as a PF6− salt from a previous study [15]. Ferrocene derivative [13] was prepared by Friedel–Craft acylation of ferrocene (ω-bromoundecanoylchloride/AlCl3/CH2Cl2) followed by a Br-to-SH interconversion sequence (refluxing with thiourea in EtOH then hydrolysis of the S-alkylthiouronium by aqueous KOH) according to known procedures [17]. Solvents for electrochemistry were of analytical grade and used as received.
3 Data analysis by impedance spectroscopy
Impedance spectroscopy offers a direct access to several quantitative parameters of interest when studying electroactive Self-Assembled Monolayers, but it is less known than cyclic voltammetry [18] or chronoamperometry [11]. It relies on the combination of two voltage components, a slow DC one and an superimposed AC one of small amplitude: the current response to this voltage combination contains the impedance Z of the cell. Practically, in the stepped mode, after a EDC potential jump of a 10 mV increment, the intensity is recorded for several frequencies f of the superimposed alternative voltage (amplitude EAC). As usual, the typical signal is a bell-shaped curve centred on the redox potential of the active species embedded in the SAM. The peak intensity Ipeak depends on the surface coverage Γ of the electroactive species. At low frequencies and for small amplitude of the superimposed alternative voltage EAC, a simple relation can be derived (Eq. (1)) where n is the number of electron exchanged, Ntot is the number of active redox sites, and F is the Faraday constant [2].
(1) |
Furthermore, Ipeak is frequency dependent: above a certain cut-off frequency, the signal vanishes totally, as the heterogeneous electron transfer is unable to keep up with the very fast potential changes. Thus, Creager and co-workers [6] have proposed an alternative representation to the Nyquist one commonly used in classical impedance spectroscopy. Indeed, by plotting Ipeak/Ibackground against log f, a sigmoid curve is obtained, showing clearly the cut-off phenomena. Such a behaviour can be simply modelled, since in the case of a SAM, the faradaic process, reflecting the adsorption of a solution anion on the SAM upon oxidation of a molecular wire, is limited by the electron transfer itself as the supporting electrolyte concentration is very high (1 M) (Schemes 2).
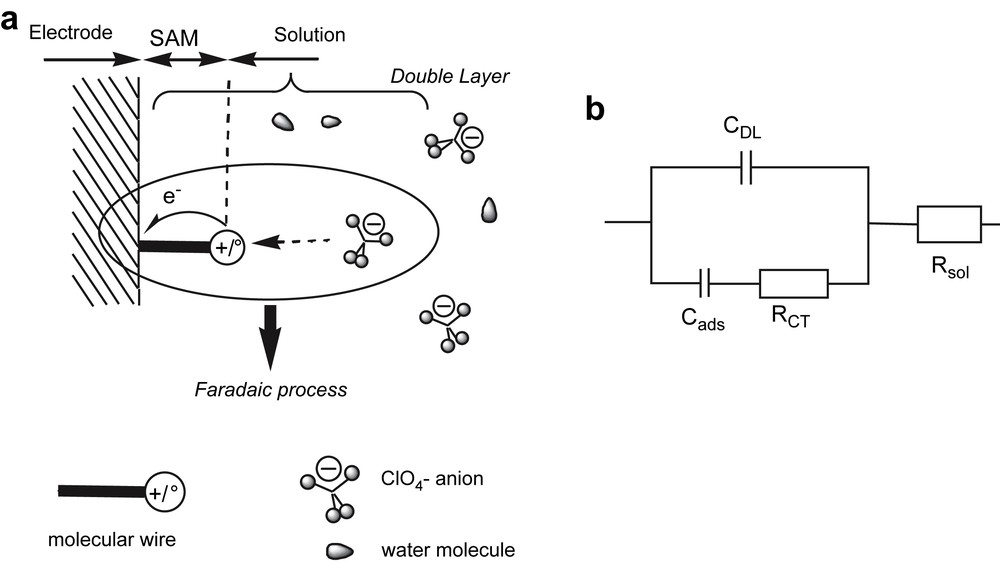
(a) Electrochemical processes at the SAM-covered electrode; (b) the Randles' equivalent circuit used to model all processes at the electrode.
Hence the Randles' equivalent circuit is made only of resistors and capacitors: RCT, charge transfer resistance; Cads, adsorption capacitance; CDL, double layer capacitance; Rsol is the solution resistance. One can show that, for E = E0, Cads, RCT and the rate constant k0 are expressed by Eq. (2), where A is the working electrode area.
(2) |
When one recognizes that Ipeak/Ibackground is also the absolute value of the ratio Y/Y0, where Y is the admittance at E = E0, and Y0 = (2πf)CDL the admittance for E = E0 if no faradaic process is active (background admittance), a simple expression can be derived (Eq. (3)) that leads to the desired curve shape:
(3) |
Thus, only two independent parameters are used to fit the sigmoid curve: k0 and ρ = 1 + Cads/CDL. The quality of the fit depends strongly on the assumption that only one rate constant depicts the faradaic process. Consequently, impedance spectroscopy with the Creager representation offers an easy way to assay the quality of a Self-Assembled Monolayer as one can extract the coverage and, if the annealing is correct, the rate constant.
4 Results and discussion
The different redox active thiol are characterized by well-separated redox potential values, and while the Ru(III)/Ru(II) couple was of 0.3 V vs. KCl/AgCl/Ag [15], the Fc+/Fc couple was found more anodic, at 0.5 V vs. Ag/AgCl. In order to measure a reproducible HET rate constant, we have adopted a two-step protocol for the SAM preparation, involving a short exposure of the clean gold surface to the mixture of thiols, followed by chemical annealing by the inert thiol component. Indeed, it is known that redox active species, once included in a SAM can present a large variation of the kinetic response mostly due to local microenvironments. Among the most common sources of deviation for the rate constant are redox active thiol molecules located at grain boundaries of SAM's nanocrystalline domains [7,19]. As all thiols in these disordered zones are also rapidly exchanged under a condition similar to the SAM formation's one, treatment of the mixed monolayer with an “inert” thiol ethanolic solution provides a way to strip these defective redox active molecules off the grain boundaries. From our previous work on the ruthenated molecule, a contact of at least 4.5 h was found necessary to get data fitting with a single rate constant, within the 20% uncertainty adopted in the literature. This is in contrast with the ferrocene-containing molecule that necessitated annealing time of more than 10 h to reach a similar situation. For both compounds, the surface coverage was in the 10−11 mol/cm2 range.
A typical set of voltammograms for the case of the three-component SAM is given in Fig. 1. The variable frequency voltammograms were acquired over a 0.1 V to 0.7 V (vs. KCl/AgCl/Ag) EDC potential range, with a typical experience time of about one hour. No particular feature due to the mixing of the two electroactive species was observed as the redox couples remained centred on E0 values identical to those observed for single electroactive co-component SAM (Fig. 1).
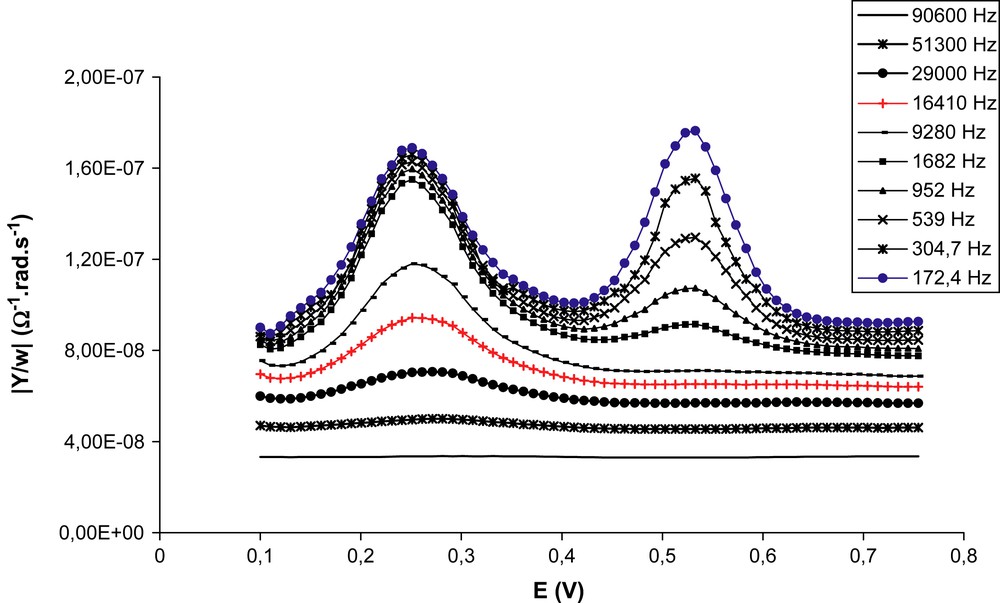
Normalized admittance curves vs. potential for the 2.5/1/30 Fc/Ru/thiol ratio for coating mixture (ΓFc = 1.4 × 10−11 mol/cm2, ΓRu = 1.3 × 10−11 mol/cm2).
By comparing the voltammograms recorded at various frequencies, one can catch qualitatively at a glance the large difference in rate constant for the HET k0. While the ruthenium centred signal persisted at rather high frequencies, the ferrocene one vanished much faster. Impedance spectroscopy on binary SAM have given quantitative values for k0(Ru) and k0(Fc), respectively, 45,000 ± 9000 s−1 [15] and 1400 ± 280 s−1, the second rate constant being very close to Lambert et al.'s result one [13]. Since both molecular wires studied are made of 10 methylene units, this difference was related to the redox sites' reorganisation energies [15]. In the case of the ternary monolayer, a similar difference in the order of magnitude is again observed (Fig. 2).
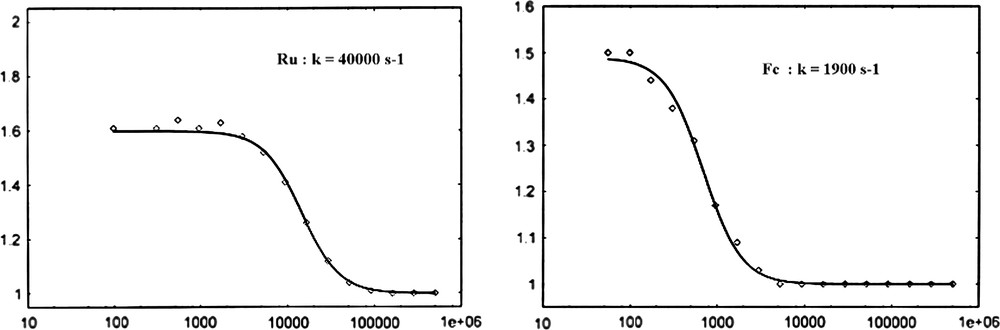
Sigmoid curves Ipeak/Ibackground as a function of the frequency f (Hz) for two redox active components [coating mixture ratio: 2.5/1/30 Fc/Ru/thiol] (ρ(Ru) = 1.6; ρ(Fc) = 1.5).
The most striking result was obtained upon varying the compositions of the ternary SAM. Surface coverage and rate constant for each species were determined in a set of experiments of comparable conditions. Results are summarized in Table 1.
Ternary SAM preparation data (annealing time, composition of the coating mixture), surface coverages and observed rate constants
Entry | Annealing time (h) | Coating solution ratio Fc/Ru/thiol | ΓFc × 1011 (mol cm2) | ΓRu × 1011 (mol cm2) | ΓFc/ΓRu | k0(Ru) × 103 (s−1) | k0(Fc) × 103 (s−1)b |
1 | 13.5 | 2.5/1/60 | 0.55 | 1 | 0.55 | 44 | 1.8 ± 0.35 |
2 | 16.5 | 2.5/1/30 | 1.4 | 1.3 | 1.07 | 40 | 1.9 ± 0.4 |
3 | 15 | 2.5/1/10 | 3.1 | 1.3 | 2.4 | 43 | 2.2 ± 0.44 |
4 | 15 | 2.5/1/5 | 5 | 2.7 | 1.9 | 47 | 3.3 ± 0.65 |
5a | 4.5 | 10/1/5 | 9.6 | 2.2 | 4.4 | 49 | 5.0 ± 1 |
a Entry 5 describes an attempt to reach a very high ferrocene coverage.
b The 20% uncertainty is given.
The first four entries show kinetic results obtained from various coating solution ratio: while the relative initial amount of ferrocene-to-ruthenium compounds was held constant, these species were diluted in decreasing quantities of inert thiol. The annealing time was chosen according to what was determined to extract reliable rate constant for the ferrocene compound. While the final surface coverage for the ruthenated molecule remained remarkably unsensitive to the composition of the coating mixture, this is not the case for the ferrocenyl one, and various ΓFc/ΓRu could be achieved. As expected, the HET rate constant k0(Ru) for the ruthenated molecule, was found to be relatively steady, its fluctuation staying within the error bar (ca. ±9 × 103 s−1). However, this was not the case for the ferrocene-containing compound the rate constant was found to depend on the ΓFc/ΓRu ratio.
No satisfactory model could be found to explain this result. The high supporting electrolyte concentration (NaClO4, 1 M) prevents any significant electrostatic interaction, as one expects the positive charges generated by the oxidation of ruthenium centres to be very quickly screened. Furthermore, the inert thiol, and both molecular wires have similar bridging parts, i.e. methylene units, which ensure not only good mutual solubility, but also similar coupling matrix elements. In a recent report it was found that no significant changes of the apparent rate constant could be detected upon varying the supporting thiol's structure. However, an important feature is the presence of oxidized ruthenium sites at the vicinity of the ferrocenyl moieties when the voltage reaches the ferrocene redox potential. Thus a possible cross-talk between the adsorbed species can be proposed: the ruthenium (III) site would act as a relay for the heterogeneous electron transfer, modifying the apparent rate constant (Scheme 3).

Hypothesis of electron transfer from the Fc unit to the electrode mediated by the Ru(III) sites.
Due to the large k0(Ru) value, this hopping mechanism would then be limited only by the distances between redox sites.
5 Conclusion
In this simple study we have confirmed the importance of the self-assembled monolayers ripening in narrowing the rate constant distribution in order to tend toward a quasi-monokinetic response for the embedded redox active species. This was achieved by partial substitution of the redox-active species by the inert component, to the detriment of the total signal to be recorded. This technique was found to be successful even for a ternary SAM containing multiple redox-active components, structurally close enough so that no pure domain formation was expected. Concerning the kinetics of the redox processes in such a structure, the most remarkable result is the dependence of one rate constant, the smallest, with the surface coverage of the species responsible for the fastest process. No satisfactory explanation was found, but a mechanism based on a mediated electron transfer seems to be a reasonable track to follow. To reach such a goal, other pairs of electroactive species should be tested, using also other electrochemical techniques. If this is confirmed, it should be taken into account when designing multi-component SAM for a higher integration level.
Acknowledgements
The authors thank Prof. J.-P. Launay, Dr. J. Bonvoisin and Prof. C. Amatore for fruitful discussions, Mrs. C. Viala for recording NMR spectra. This work was supported by the CNRS, the “Ministère de l'Éducation nationale, de la Recherche et de l'Enseignement supérieur”, and was partly funded by the 5th IST–FET Nanomol European Network.