1 Introduction
Nanoscaled materials are a field of growing interest due to the fundamental differences in properties between nanoscale and bulk materials [1]. For this reason, the synthesis of organically functionalized silicon nanoparticles (Si-nps) is currently an area of intense research. However, the great difficulty is the existence of the covalent bonds Si–Si which imply the use of very efficient reducing reagents. Many routes have been developed for preparing organically functionalized Si-np. Two types of strategies have been developed: physical and chemical routes. Access to Si-np by physical routes consists in “top–down” [2] or “bottom–up” [3] strategies. In most cases, a good control of composition and size of the particles was obtained, despite some oxidation of the surface [4–6]. The second strategy consists in solution routes. However, the products have been generally limited to aliphatic surface substituents. For example, alkylated Si-np (Si–C linkage) were synthesized by reduction of chlorosilanes with Na at high pressure and temperature [7] or by reduction of SiCl4 with NaSi [8], Mg2Si [9], or Na naphthalenide [10], followed by reaction with Grignard or alkyl lithium reagents, and by hydrosilylation of 1-alkenes with Si-np–H [11–13]. Alkoxylated Si-np (Si–O–C linkage) have been prepared by reducing SiCl4 with Zintl salts followed by treatment with alcohol [14], through thermal decomposition of diphenyl silane (SiH2Ph2) in supercritical octanol [15], by thermally activated reaction of alcohols with polydisperse Si-np–H [16] and by functionalization via hydrosilylation with 1-alkenes [17]. Recently, hydrogen terminated silicon nanoparticles (Si–H linkage) have been obtained from sodium silicide and ammonium bromide in DME or dioctylether [18,19]. Other routes to silicon nanoparticles involving silicon tetrachloride [20–24] or tetraethoxysilane [25] have also been described. Among the reducing agents employed, C8K appears to be very efficient [26–29]. We have recently reported a new solution route to silicon nanoparticles functionalised at the surface in two steps. The first one consists of the use of potassium incorporated in graphite (C8K) as reducing agent of silicon tetrachloride in THF which leads to chlorinated Si-np and is followed by their reaction with various nucleophile reagents [30]. Unfortunately this route was limited because of a side reaction of the chlorinated Si-np with the solvent (THF) [30]. In this paper we report an improved synthesis of surface functionalized silicon nanoparticles using dimethoxyethane (DME) as solvent and a variety of nucleophiles.
2 Experimental
2.1 Materials and instruments
Analytical grade reactants SiCl4, 2-propanol, 1-butanol, 1-hexadecanol, di(ethyleneglycol)hexyl ether, (3-aminopropyl)trimethoxysilane and 3-[2-(2-aminoethylamino)ethylamino]propyl-trimethoxysilane were purchased from Aldrich and used as received. Reactions were performed under an inert atmosphere of dry argon using standard Schlenk techniques and vacuum lines (10−1 mbar). 1,2-Dimethoxyethane was dried and distilled from Na/benzophenone prior to use. Graphite powder (−20 + 84 mesh, 99.9%) was purchased from Alfa aesar and washed three times with ethanol and diethylether then dried at 120 °C for 8 h under reduced pressure (10−1 mbar).
FTIR spectra were recorded on a Perkin Elmer 1600 series IRFT, spectra of liquids between NaCl pellets and spectra of solids in dilution of 95% dry KBr. A blank KBr pellet was examined before each sample. Liquid-phase NMR spectra were recorded at room temperature on a BRUKER ADVANCE DPX 200 (1H, 13C) spectrometer. Chemical shifts are referenced in ppm. Solid-phase NMR spectra were recorded at room temperature with a BRUKER ADVANCE DPX 300 spectrometer (13C). Elemental analysis was performed at Laboratoire Central d'Analyse du C.N.R.S. in Vernaison. Air sensitive compounds were handled under argon and elemental analysis was done without any contact with air. UV–visible solid spectra were recorded on a UV–visible Perkin Elmer Lambda 14. A blank BaSO4 pellet was examined before each sample. Photoluminescence spectra were recorded on an Aminco SLM 8100. TEM pictures and related SAED were recorded on a 100 kV JEOL JEM-1200EX microscope.
2.2 Synthesis of functionalized Si-np
C8K was prepared according to literature [31] by reacting potassium (7.33 g, 0.19 mol) with graphite powder (18.00 g, 1.5 mol) at 150 °C for 15 min under vigorous stirring in a three-necked, flame-dried, 500-mL round-bottomed flask. The resulting product was a powder with a characteristic gold color. It was then suspended in 150 ml of DME and cooled at 0 °C. A solution of SiCl4 (9.04 g, 0.05 mol) in 50 ml of DME was added (4 times 12.5 ml over a period of 20 min). The gold powder gradually turned black. The suspension was stirred overnight at room temperature and then refluxed for 1 h. Analysis of the liquid phase revealed the presence of traces of unreacted chlorosilane. The chlorine capped Si-np were insoluble in DME and it was impossible to separate them from graphite and KCl. The solvent was removed by heating in an oil bath under reduced pressure (10−1 mbar) in order to eliminate the side products.
The functionalized Si-np D1–D6 were prepared according to the same experimental procedure. The synthesis of D4 is given as an example.
The mixture D0/graphite/KCl previously prepared was suspended in 150 ml of DME. The mixture was cooled to −30 °C. A solution of di(ethylene glycol)hexyl ether (8.48 g, 45 × 10−3 mol) in 40 ml of DME was slowly added. Once the solution returned to room temperature, it was stirred for 30 min and then refluxed for 1 h. Graphite and KCl were removed by filtration. Then the excess of reagent and solvent were removed by heating in an oil bath under reduced pressure (10−1 mbar). An orange oil D4 was isolated.
- D1, reagent: 2-propanol, 2.78 g (46 × 10−3 mol)
- D2, reagent: 1-butanol, 3.20 g (43 × 10−3 mol)
- D3, reagent: 1-hexadecanol, 10.93 g (45 × 10−3 mol)
- D4, reagent: di(ethylene glycol)hexyl ether, 8.48 g (45 × 10−3 mol)
- D5, reagent: (3-aminopropyl)trimethoxysilane, 7.85 g (44 × 10−3 mol)
- D6, reagent: 3-[2-(2-aminoethylamino)ethylamino]propyl-trimethoxysilane, 10.77 g (41 × 10−3 mol)
D1: yield 0.34 g, 10%, orange solid, mp >300 °C (dec), (Found: Si, 41.1; Cl, 1.2; C, 15.1; H, 4.4; Calc. for Si1(C3H7O)0.12: Si, 79.8; C, 12.3; H, 2.4%), (νmax/cm−1): 3380; 2974; 2935; 2251; 2122; 1632; 1466; 1387; 1374; 1052 (br); 876; 619; δC (75 MHz; HPDEC; Me4Si): 26.3; 66.1.
D2: yield 0.27 g, 7%, orange solid, mp >300 °C (dec), (Found: Si, 35.9; Cl, 3.1; C, 29.4; H, 6.2; Calc. for Si1(C4H9O)0.12: Si, 76.2; C, 15.7; H, 2.9%), (νmax/cm−1): 3378; 2962; 2927; 2868; 2248; 2108; 1462; 1376; 1262; 1090 (br); 874; 803; 639; δC (75 MHz; HPDEC; Me4Si): 13.4; 19.2; 22.6; 64.3.
D3: yield 0.36 g, 6%, orange solid, mp >300 °C (dec), (Found: Si, 23.6; Cl, 0.6; C, 42.7; H, 8.2; Calc. for Si1(C16H33O)0.12: Si, 49.1; C, 40.5; H, 7.0%), (νmax/cm−1): 3372; 2923; 2852; 2243; 2114; 1467; 1388; 1378; 1086 (br); 880; 803; 720; 646; 428; δC (75 MHz; HPDEC; Me4Si): 14.2; 23.6; 26.6; 29.8; 32.4; 62.6.
D4: yield 1.90 g, 21%, orange oil, mp >300 °C (dec), (Found: Si, 15.4; Cl, 1.2; C, 56.3; H, 10.1; Calc. for Si1(C10H21O3)0.12: Si, 55.2; C, 28.4; H, 5.0%), (νmax/cm−1): 3482; 2960; 2858; 2739; 2217; 2108; 1461; 1356; 1293; 1250; 1080 (br); 971; 875; 801; 727; 521; 436; δC (75 MHz; CDCl3; Me4Si): 12.4; 22.9; 25.8; 29.8; 32.5; 61.3; 69.9; 70.8; 72.8.
D5: yield 1.56 g, 24%, yellow oil, mp >300 °C (dec), (Found: Si, 21.6; Cl, 3.2; C, 33.6; H, 7.5; N, 5.0; Calc. for Si1(C6H16NO3Si)0.12: Si, 63.5; C, 17.5; H, 3.9; N, 3.4%), (νmax/cm−1): 3384; 2938; 2839; 2128; 1460; 1409; 1248; 1191; 1086; 816; 668; δC (75 MHz; CDCl3; Me4Si): 6.4; 27.1; 44.6; 50.4.
D6: yield 2.51 g, 30%, yellow oil, mp >300 °C (dec), (Found: Si, 16.7; Cl, 2.9; C, 36.3; H, 8.5; N, 9.7; Calc. for Si1(C10H26N3O3Si)0.12: Si, 52.5; C, 24.1; H, 5.2; N, 8.4%), (νmax/cm−1): 3294; 2940; 2840; 1575; 1463; 1411; 1345; 1306; 1192; 1083 (br); 816; 478; δC (75 MHz; CDCl3; Me4Si): 6.8; 22.9; 41.6; 48.7; 50.3; 51.9.
3 Results and discussion
3.1 Synthesis of functionalized silicon nanoparticles
Chlorine-terminated silicon nanoparticles were prepared via the reduction of silicon tetrachloride with potassium-graphite in DME. The ratio SiCl4/C8K employed was 0.28 since we have previously shown that the best results were obtained using this stoichiometry [30]. The Cl-terminated Si-np D0 appeared to be insoluble in DME and the further substitution of Cl atoms was performed directly on the crude reaction mixture without elimination of graphite and KCl (Scheme 1).

(a) Insoluble and impossible to separate from graphite.
However, in order to remove the unreacted chlorosilanes, the solvent was pumped off under vacuum (10−1 mbar). The mixture D0/graphite/KCl was then suspended in DME and treated by an excess of various nucleophilic reagents at −30 °C. The reaction mixture was allowed to warm up slowly to room temperature, stirred for 30 min at this temperature, and then refluxed for 1 h. Graphite and potassium chloride were removed by filtration. The solvent and the unreacted reagents were pumped off under vacuum (10−1 mbar) from the resulting yellow or orange solution, leading either to a solid or to a viscous oil (Scheme 1).
The different nucleophilic reagents were selected in order to obtain a variety of surface types through the formation of Si–C, Si–O, or Si–N bonds with different lengths of chains. In the case of methyl and butyl groups, the compounds D7 and D8 were insoluble materials that could not be separated from graphite and KCl.
The elemental analyses gave Cl/Si ratios close to zero. This suggests that the nucleophilic substitution of Si–Cl functions in D0 was almost complete. However, the ratios C/Si were generally higher than the theoretical values, suggesting that D0 contained more Si–Cl functions than expected, due to an incomplete reduction of SiCl4.
3.2 Spectroscopic characterization
The nanoparticles were obtained as insoluble solids (D1–D3) or waxy solids (D4–D6). In the case of D4–D6, solution 1H NMR spectra were consistent with the presence of the organic groups on the surface of the nanoparticles. This was also supported by FTIR spectroscopy, which showed for all samples (D1–D6) strong peaks in the stretching region 2980–2850 and medium peaks in the bending/scissoring region 1450–1350 cm−1 indicative of the presence of both methylene and methyl groups. These vibrations were attributed to the alkyl chains. In addition, strong vibrations at ∼1050 cm−1 corresponded to the C–O–C or C–O–Si groups. For D5 and D6 the peaks usually attributed to N–H (∼3150 cm−1), C–N (∼1200 cm−1) and Si–N (∼800 cm−1) vibrations were present. The 13C NMR spectra exhibited signals corresponding to the surface organic groups, as illustrated by the spectra of D3 and D4 in Fig. 1.
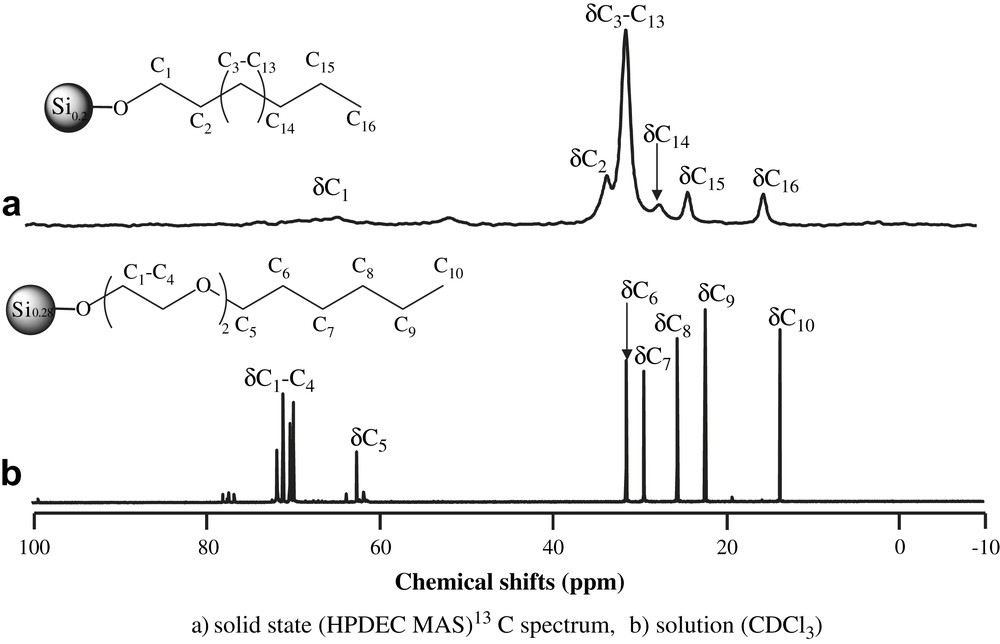
13C NMR spectra of (a) D3a; (b) D4b. (a: solid state (HPDEC MAS) 13C spectrum, b: solution (CDCl3).)
Similarly, the signals expected for each sample, depending on its functionality, could be identified in the respective spectra (Section 2).
3.3 Transmission electron microscopy (TEM)
TEM, HRTEM and EDX were used to confirm the formation of nanoparticles in the case of D1–D3. The Si-nps were deposited on a holy carbon grid via evaporation of a pentane suspension. All the reactions led to nanoparticles that showed similar micrographs (shape and size in all cases). The nanoparticles appeared to be of uniform size but there were large numbers of particles that were aggregated (Fig. 2).
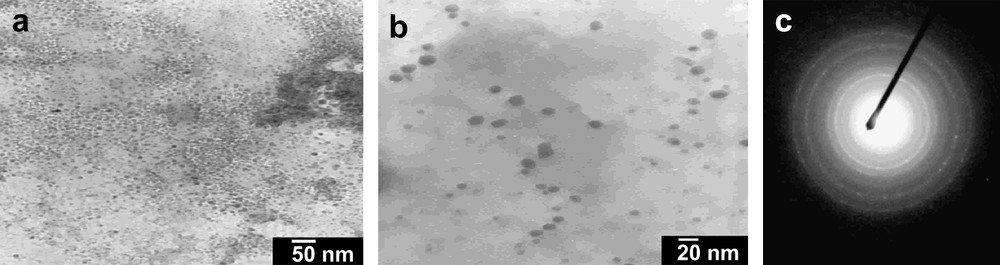
Micrograph of D1 and electronic diffraction.
A large number of homogeneous round-shaped isolated particles were observed and a histogram of the size distribution was constructed based on a batch of 700 nanoparticles. Fig. 3 shows this histogram in the case of D1. The average diameter was 5 nm.
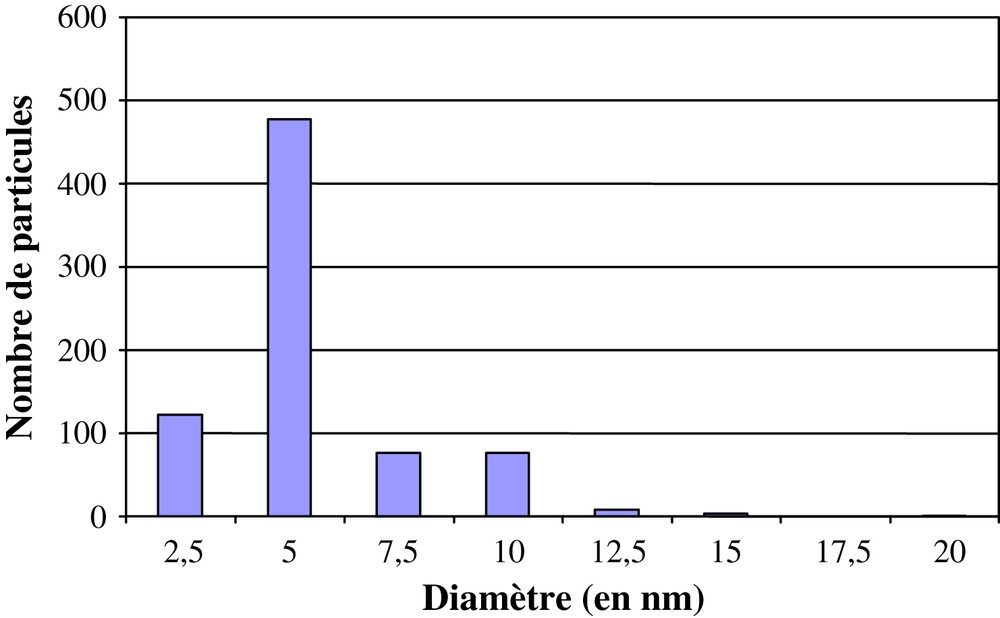
Histogram of particle sizes from a survey of 700 particles of D1 from different regions of the grid.
Selected area electron diffraction (SAED) showed a diffraction pattern with well-defined rings presenting more luminous spots. These were attributed to disordered nanocrystals (Fig. 2). Inter-reticular distances were measured on the first five rings using a gold sample as reference. The experimental values obtained corresponded to the standard values of diamond cubic crystalline silicon from Joint Committee for Powder Diffraction Standard (JCPDS) (Table 1).
Experimental and literature inter-reticular distances
dhkl measured (Å) | dhkl silicon (Å) | {hkl} |
3.16 ± 0.14 | 3.14 | 111 |
1.91 ± 0.05 | 1.92 | 220 |
1.65 ± 0.04 | 1.64 | 311 |
1.25 ± 0.02 | 1.25 | 331 |
1.10 ± 0.02 | 1.11 | 422 |
Fig. 4 gives a HRTEM of a single large nanoparticle (∼8 nm). The diagonal striations corresponding to lattice fringes are clearly visible. The lattice fringes' spacing (0.312 nm) was consistent with the {111} plane of diamond crystalline silicon.

HRTEM image of D1. Dark striated region is an 8 nm silicon nanoparticle with clearly visible lattice fringes.
This was further confirmed by energy dispersive spectroscopy (EDS), which showed that the particles’ core consisted mainly of silicon (90%), with some residual chlorine (∼10%) and potassium (<1%). The presence of chlorine could result from the presence of remaining Si–Cl bonds. This has been already observed in the case of Si-np prepared from SiCl4 and sodium naphthalenide [32]. Despite their electron diffraction pattern in TEM, the nanoparticles exhibited no signal on powder XRD diagrams. This was consistent with particles with a small crystalline core [33] and unstructured peripheral matter. The absence of signal in the XRD diagrams confirmed that graphite or KCl, by-products of the reaction were not present in the samples, or only as traces.
3.4 Optical properties
The optical properties of Si-np were studied by UV–visible and photoluminescence spectroscopies at room temperature. It appeared that only the oily derivatives D5 and D6 exhibited an emission at 400 nm when they were excited at 334 nm, that is, in the range already reported in literature [9,21,24]. The other nanoparticles D1–D4 had no emission. This could originate from the functionalization of silicon nanoparticles that was performed on the crude reaction mixture. This could have an influence on the size of the particles that were extracted from graphite as well as the nature of the functional groups introduced at the surface of the particles: only the Si-nps presenting amino groups exhibited photoluminescence.
4 Conclusion
The solution route reported here allowed us to obtain silicon nanoparticles bearing a large range of surface functionalities. The nature of the substituent and the size of the particles were key factors for their solubility and extraction from graphite. The extracted Si-nps were around 5 nm in size and showed some order at the nanoscale. Photoluminescence was observed with amino substituents only, confirming the role of the surface functionality on the nanoparticles’ optical properties. Further work is in progress to include these Si-nps into oxide matrices in order to obtain a new class of hybrid organic–inorganic materials.
Acknowledgements
The authors thank Dr. Pierre Masri (GES-UM2) for fruitful discussion.