The design and synthesis of new ligands will continue to be one of the pillars sustaining the field of modern coordination chemistry as it faces ambitious challenges, such as the search for multifunctional materials and molecules, or the advancement of fundamental scientific knowledge through the synthesis and study of targeted systems. Since the emergence of the field of metallo-supramolecular chemistry, several families of organic molecules have been studied and expanded for their use as ligands within intricate coordination ‘architectures’. One of the largest and best known such families is that of oligo-pyridyl (or other N-heterocycles) molecules [1], although other important categories exist and continue to grow. Among these are poly-catechols [2], multi-porphyrins [3] or poly-β-diketones [4,5]. We have designed and prepared a family of bis-β-diketone ligands that include also phenol groups in their molecules [6,7], so as to dispose arrays of adjacent O-based chelating pockets aimed at stimulating the assembly of linear chains of closely spaced paramagnetic metals. One of the aims of this work is to study the magnetic properties associated to these targeted and sometimes original molecular metallic arrangements. This effort has fructified in the preparation of a large series of coordination clusters, all based on linear metallic arrays, with nuclearities ranging from 2 to 4 [8–11]. In some cases, the aggregation of these clusters has lead to the formation of larger molecules or coordination polymers [12,13]. The ligand H4L, shown in Scheme 1 (top), was prepared to induce the formation of linear tetranuclear clusters disposed as two pairs of interacting metals (Scheme 1, bottom). Previous work revealed that the use of acetates as metal salts in front of this ligand provided a base capable of removing the protons from the β-diketones, but not strong enough to react with the protons of the phenol groups. Thus, a series of dinuclear complexes, exhibiting the coordination mode shown in Scheme 1 (middle) were obtained and characterized with Mn(II), Co(II), Ni(II) and Cu(II) [14]. However, in order to prepare the targeted tetranuclear clusters, a stronger base needs to be employed in the reaction. We report here the first example of one such cluster, consisting of the expected [Ni–Ni⋯Ni–Ni] moiety as assembled by the action of two fully deprotonated ligands, L4−.
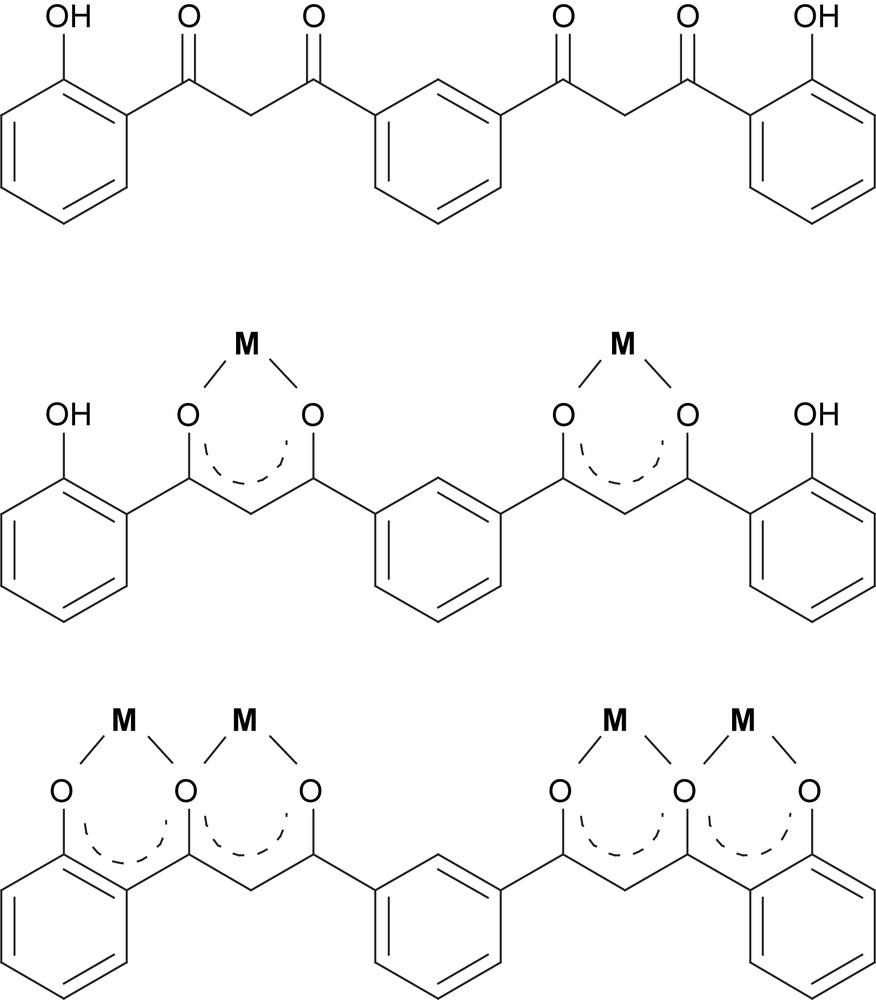
(Top) structure of H4L; (middle) representation of the coordination mode μ-(H2L)2−; (bottom) representation of the coordination mode μ4-(L)4−.
The reaction in pyridine of two equivalents of Ni(ClO4)2 with H4L, previously deprotonated in solution with four equivalents of the organic hydroxide (NnBu4)OH, leads to a solution that, upon layering with MeOH, produces crystals of the complex [Ni4L2(py)6] (1)1. The reaction can be described with the chemical equation (1).
4Ni(ClO4)2 + 2H4L + 8(NnBu4)OH + 6py → [Ni4L2(py)6] + 8(NnBu4)ClO4 + 8H2O | (1) |
The structure of 12 (Fig. 1) consists of a neutral centro-symmetric [Ni4L2(py)6] complex of four Ni(II) ions coordinated equatorially by two μ4:η6-L4− ligands disposed opposite to each other. Each ligand accommodates four metals within four pair-wise adjacent coordination pockets, formed by two β-diketonate and two phenolate groups. The metals are therefore distributed in two equivalent pairs which are maintained separated from each other within the molecule. These metals are not contained in one unique vector but instead within two parallel directions. A total of six pyridine ligands are occupying axial positions of the Ni(II) metals. Interestingly, the coordination geometries of the metals within the Ni2 pairs are dissimilar; the metals located at both ends of the [Ni4L2(py)6] unit are square pyramidal (thus, five-coordinate) whereas the inner metals are six-coordinate, exhibiting octahedral geometry. Inspection of the structure suggests that geometric constraints of the L4− ligand, together with steric encumbrance is the reason why one Ni(II) per pair does not take a second molecule from the solvent to adopt this ion's most common octahedral geometry, as is observed in some dinuclear complexes of the type [Ni2(L′)2(py)4] (H2L′ are 1,3,5-triketone ligands) [15,16]. Indeed, the ligand L4− does not seem to have the capacity to accommodate within one plane the equatorial planes around the NiII ions of each Ni2 pair. Instead, these planes (least square planes of the four oxygen atoms around the metal) form a mutual angle of 5.1(5)°, which blocks the entry to one of the axial ligands (see space-filling diagram in Fig. 2). Within each Ni pair, the equatorial O-atoms around Ni2 and both metals (a Ni2O4 fragment) are nearly within a plane, whereas the remaining two O-atoms from the square pyramidal Ni(II) center are away from that plane. The ligands, in turn, display a sinusoidal conformation (Fig. 1). The Ni⋯Ni distance within the Ni2O2 pairs is 3.098(2) Å. In between pairs, the shortest intramolecular metal–metal distance is 7.279(3) Å. Other metric parameters are summarized in the caption of Fig. 1.
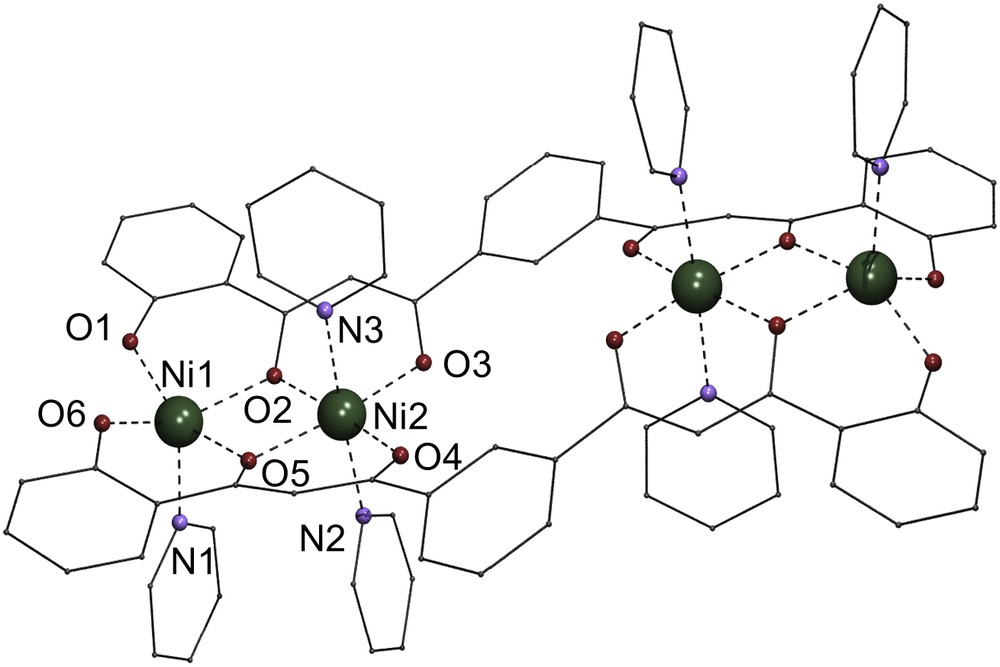
PovRay labeled representation of the molecular structure of the complex [Ni4L2(py)6] (1), emphasizing the intended [Ni–Ni⋯Ni–Ni] topology of metals as held by two L4− ligands. Selected distances (or ranges) (Å) and angles (°); Ni1–O, 1.921(7)–2.073(6); Ni1–N1, 2.027(8); Ni2–O, 1.989(6)–2.045(6); Ni2–N2, 2.080(7) Ni2–N3, 2.119(8); Ni1⋯Ni2, 3.098(2); Ni2⋯Ni2a, 7.279(3); Ni1–O2–Ni2, 98.9(2); Ni1–O5–Ni2, 97.6(2).
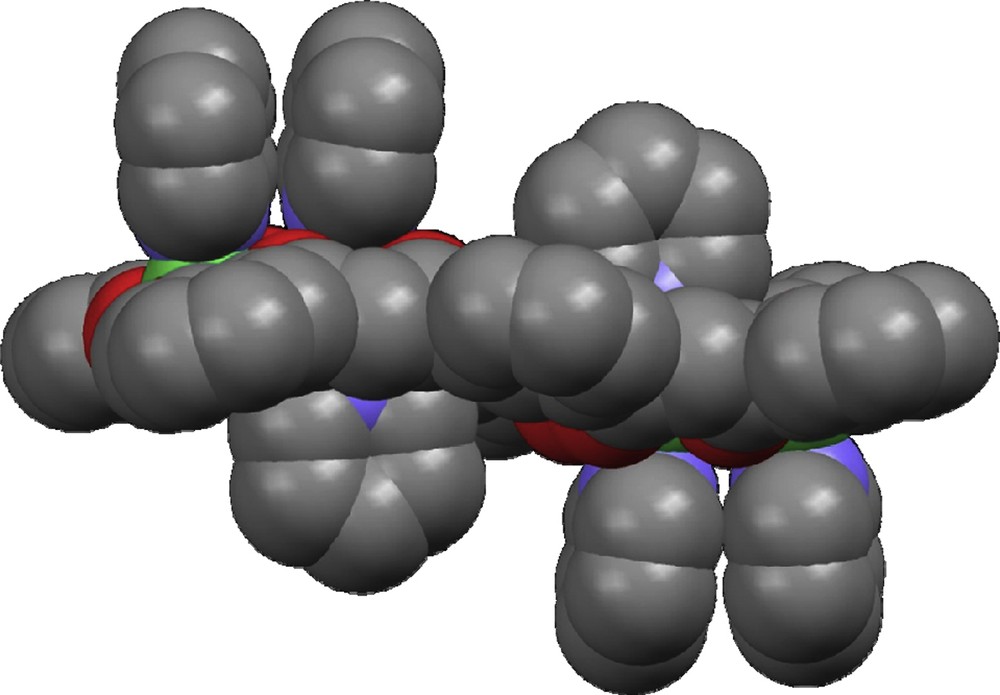
Space-filling diagram of the molecular structure of [Ni4L2(py)6] (1), emphasizing the fact that the binding of a second pyridine ligand to the five-coordinate metal is precluded for steric reasons.
The magnetic properties of complex 1 were investigated by means of bulk, variable temperature magnetization measurements under a constant magnetic field of 1 T, on a powdered, air-dried sample of the complex.1 The results are shown in Fig. 3 in form of χMT vs T plots, where χM is the paramagnetic susceptibility per mole of 1. At 300 K, the product is 4.45 cm3 K mol−1 (nearly that corresponding to four independent Ni(II) centers with g = 2.08) and decreases steadily on cooling down to 0.3 cm3 K mol−1 at 2 K. This behavior reflects the presence of weak antiferromagnetic coupling between the Ni(II) ions within the [Ni2] pairs. A quantification of the Ni⋯Ni exchange coupling through the diketone O double bridge was performed through a fit to the susceptibility data of a theoretical χM = f(T) expression derived from the Van Vleck equation. The latter was the one derived for the spin Hamiltonian H = 2×[−2JS1S2 + gβSTz], where S1 = S2 = 1 and ST is the total spin of one Ni2 pair [17]. No attempt was made of including other additional coupling pathways, such as potential Ni⋯Ni interactions via the L4− ligands or introducing single-ion zero field splitting parameters into the χM = f(T) expression since, being the main interaction antiferromagnetic, other possible weaker interactions or effects will be masked by the former. The best fit was obtained for J = −5.04 cm−1 and g = 2.08 (Fig. 3) which confirms that the coupling within the Ni2 pairs is relatively weak. Magneto-structural correlations for alkoxido-bridged Ni(II) ions indicate that Ni–O–Ni angles smaller than 99° favor ferromagnetic interactions [18,19]. The angles observed in 1 are 98° and 99°, respectively, thus near the limiting value. The fact that weak antiferromagnetic coupling is observed does not contradict the above mentioned correlations, since these were conducted with all octahedral Ni(II) centers.

Plot of χMT vs T per mol of complex 1 at a constant field of 1 T. The solid line is a fit to the experimental data.
In conclusion, the multidentate ligand H4L has been employed in the appropriate conditions to successfully prepare a tetranuclear [Ni4] cluster with a targeted linear topology featuring a [Ni–Ni⋯Ni–Ni] sequence. Magnetic investigations have demonstrated the existence of weak antiferromagnetic exchange within the [Ni–Ni] pairs. The geometric constraints imposed by the multidentate donor L4− cause an unusual combination of square pyramidal with octahedral coordination geometries within couples of adjacent Ni(II) centers. This non-anticipated property could be used for the deliberate preparation of heterometallic clusters with the same topology as 1, involving metals with a preference for octahedral geometry together with cations that coordinate preferentially within a square pyramidal environment.
Acknowledgements
We acknowledge the Spanish Ministry of Science and Education (GA), the Leiden University Study Group (Werkgroep Fundamenteel Materialen-Onderzoek, WFMO) (PG). The Advanced Light Source (SJT) is supported by the Director, Office of Science, Office of Basic Energy Sciences, of the U.S. Department of Energy under Contract No. DE-AC02-05CH11231.
1 Anal. Calcd. (Found) for 1·(3.7MeOH·2H2O): C, 58.57 (59.09); H, 4.66 (4.06); 5.06 (5.06).
2 Crystal data for 1·(4.5MeOH): C82.50H76N6Ni4O16.50. Data were collected on a yellow needle (0.20 × 0.04 × 0.04 mm), monoclinic, space group P21/n (no. 14) with a = 11.664(4), b = 18.406(7), c = 18.854(7) Å, β = 103.706(7)°, V = 3932(2) Å3, Z = 2, ρcalcd = 1.394 g/cm3, μ = 1.276 mm−1. Out of 17,634 reflections measured, 5614 (Rint = 0.0605) were independent, on station 11.3.1 of the Advanced Light Source synchrotron facility (T = 150 K, 3.11° < θ < 25.54°, λ = 0.77490 Å). The structure was solved by direct methods (SHELXS97), and refined on F2 (SHELXL-97). The molecule was refined anisotropically and its hydrogens were placed geometrically. The disordered solvent molecules were refined isotropically with displacement parameter and geometrical restrains, while their hydrogens were omitted from the refinement. Refinement of 503 parameters converged at final wR2 = 0.1579, R1 = 0.0719 and S = 1.081 (for 3996 reflections with I > 2σ(I)), −0.648 < Δρ < 1.162 e Å3. CCDC-666285 contains the supplementary crystallographic data for this paper. These data can be obtained free of charge via www.ccdc.cam.ac.uk/data_request/cif or by emailing data_request@cdc.cam.ac.uk, or by contacting the Cambridge Crystallographic Data Centre, 12, Union Road, Cambridge CB2 1EZ, UK; fax: +44 1223 336033.