1 Introduction
Volatile Organic Compounds (VOCs) are recognized as major contributors to air pollution, either directly through their toxic nature, or indirectly as ozone and smog precursors. Catalytic combustion is an efficient way to control the emission of VOCs at low concentration. The most efficient catalysts belong to two categories: supported noble metal catalysts (Pd, Pt and Au) [1,2] and transition metal oxides such as Co and Cu oxides [3]. Noble metal shows the lower light-off temperatures in the oxidation of hydrocarbons [4]. Palladium and platinum are extensively used as active and selective components in the complete oxidation of VOCs [5]. Palladium is particularly interesting for catalytic combustion application in low VOC concentration due to its unusual behaviour in an oxygen or air-rich atmosphere [6]. Modification of palladium with gold often results in improvement in the catalytic activity of palladium for oxidation [2,7]. The addition of a second metal can favour the reduction of the first, increase the dispersion of a metal which has a tendency to form large particles or decrease its fritting [8]. The activity and selectivity of support Pd and Au catalysts in total oxidation have been reported to depend on the type of metal oxide support [9,10]. Therefore, the selection of an efficient support is a decisive factor to obtain catalysts with a good performance. The state and structure of the support strongly influence the catalytic activity and selectivity of the gold-based catalysts. Titania and zirconia have been found to be promising materials, both as an active centre and a support, for VOCs oxidation [11,12]. Recently, much attention was paid to the synthesis of nanostructured mesoporous oxides with high surface area and uniform pore size distribution using the surfactant templating method [13,14,15]. In the present work TiO2–ZrO2 mixed oxides were synthesised using a single surfactant. The results concerning the synthesis and characterisation of the palladium and/or gold supported catalysts are presented and the catalysts were tested in complete toluene oxidation. The specific properties of the nanostructured mesoporous titania–zirconia mixed oxide and their influence on the catalytic activity results are also discussed.
2 Experimental
Nanostructured mesoporous TiO2–ZrO2 mixed oxides with various Ti:Zr molar ratios, 80/20, 50/50 and 20/80, were synthesised using a single surfactant as templating agent. A micellar solution of CTMABr was prepared by dissolving the surfactant at room temperature in an aqueous acidic solution (pH = 2). The inorganic sources were added drop by drop to the solution. The precursors employed were Ti (OC3H7)4 (98%, Aldrich) and Zr (OC3H7)4 (70%, Chempur). The surfactant/precursors molar ratio was 0.33. The gel obtained after stirring for 1 h at room temperature was sealed into teflon autoclaves and heated. The hydrothermal treatment was performed for 24 h at 80 °C. The template was completely removed after 48 h of ethanol extraction. The mesoporous titania–zirconia mixed oxide was dried at 40 °C and calcined in air at 400 °C for 4 h. The synthesised supports were denoted TZ 80/20, TZ 50/50 and TZ 20/80 (titania–zirconia is denoted as TZ).
The new supports are impregnated with 0.5 or 1.5 wt% of palladium and 1 wt% of gold. The catalysts were prepared in accordance to the order of deposition of the promoters (gold and palladium):
- ● 0.5 and 1.5 wt% of palladium on mesoporous supports denoted respectively as Pd/TZ and 1.5Pd/TZ;
- ● 1 wt% of gold on mesoporous supports denoted as Au/TZ;
- ● 0.5 wt% of palladium on mesoporous supports promoted by 1 wt% of gold, denoted as PdAu/TZ;
- ● 1 wt% of gold on mesoporous supports promoted by 0.5 wt% of palladium, denoted as AuPd/TZ.
The palladium and/or gold samples were prepared by a method previously described by our group [2]. Gold was deposited using the Deposition–Precipitation method and a HAuCl4 solution. Palladium supported samples were prepared by aqueous impregnating method using palladium nitrate. The samples were dried at 80 °C and calcined in air at 400 °C for 4 h.
The solids obtained were all characterised by thermal analysis, specific area analysis, X-ray diffraction (XRD), H2 temperature programmed reduction (TPR) and electron paramagnetic resonance (EPR).
The structures of the solids were analyzed by powder XRD technique at room temperature with a Bruker diffractometer using Cu Kα radiation scanning 2θ angles ranging from 10 to 80°.
TPR experiments were carried out in an Altamira AMI-200 apparatus. The TPR profiles were obtained by passing a 5% H2/Ar flow (30 ml min−1) through the calcined sample (about 100 mg). The temperature was increased from −40 to 300 °C at a rate of 5 °C min−1. The hydrogen concentration in the effluent was continuously monitored by a thermo conductivity detector (TCD).
Thermal analysis measurements were performed using a Netzsch STA 409 equipped with a microbalance differential analysis (DTA) and a flow gas system. The dried catalyst was treated under air; the temperature was raised at a rate of 5 °C min−1 from room temperature to 1000 °C.
The specific areas of solids were determined by BET method using Quantasorb Junior apparatus and the gas adsorbed at −196 °C is pure nitrogen.
EPR measurements were performed at −196 °C and 25 °C on an EMX Brüker spectrometer. A cavity operating with a frequency of 9.5 GHz (X band) was used. Precise g values were determined from simultaneous precise measuring of frequency and magnetic field values. All EPR spectra were treated with the Brüker WINEPR program.
The mono and bimetallic catalysts were tested in the oxidation of toluene. Toluene oxidation was carried out in a conventional fixed bed microreactor and studied between 25 and 400 °C (1 °C min−1) with “operando” Diffuse Reflectance Infrared Fourier Transform (DRIFT) spectroscopy. The reactive flow (100 mL min−1) was composed of air and 1000 ppm of gaseous toluene or propene. Before the catalytic test, the solid (100 mg) was calcined under a flow of air (2 L h−1) at 400 °C (1 °C min−1) and reduced under hydrogen flow (2 L h−1) at 200 °C (1 °C min−1).
3 Results and discussion
The X-ray diffraction patterns and UV-visible spectra (not shown) of the samples have shown that the synthesised supports remain amorphous after calcination at 400 °C. Anatase and tetragonal crystalline phases of titania and zirconia, usually obtained [16] were not observed.
The BET areas, the pore volume and the average pore diameter obtained by nitrogen adsorption–desorption isotherms (not shown) of the supports are presented in Table 1. The BET supports areas changes according to the titanium/zirconium molar ratio. However, the low differences between the surface area of the untreated and calcined sample can be explained by the amorphous character of the titania–zirconia mixed oxides.
BET surface areas, average pore diameter of the supports before and after the catalytic test.
Sample | BET Surface area (m2/g) | Pore diameter (nm) | |
Calcined at 400 °C | After the catalytic test | ||
TiO2–ZrO2 80/20 | 601 | 553 | 2.9 |
TiO2–ZrO2 50/50 | 410 | 353 | 5.5 |
TiO2–ZrO2 20/80 | 372 | 323 | 2.0 |
The catalytic performances of the supports were carried out in toluene total oxidation (Fig. 1). Catalytic activity of the samples at the temperature for 50% of toluene conversion (T50) changes according to the titanium/zirconium molar ratios and their surface areas: TZ 80/20 > TZ 50/50 > TZ 20/80. Among these supports the catalyst TZ 80/20 is the most active.
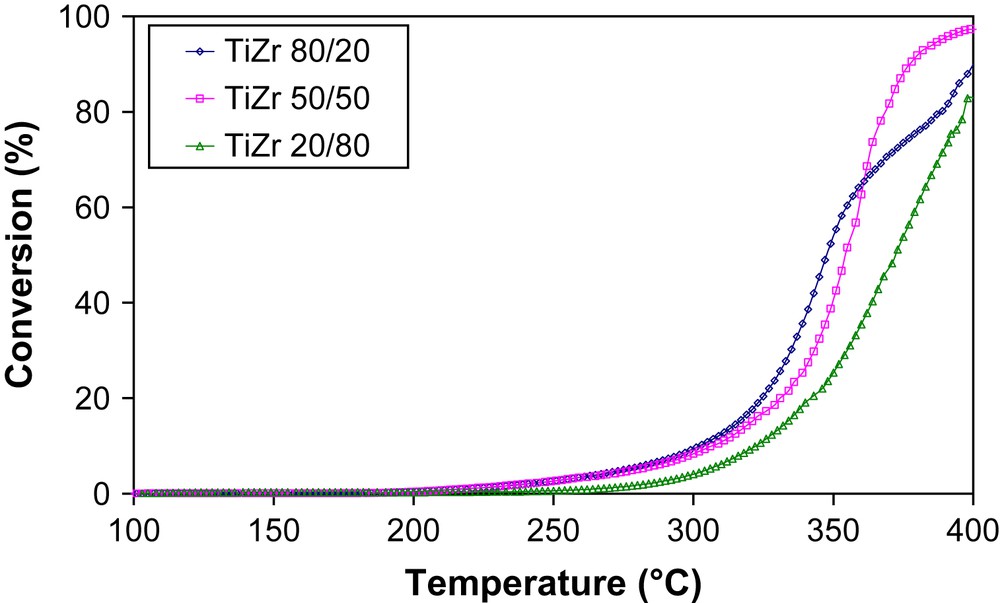
Conversion of toluene on the various TiO2–ZrO2 supports.
The TPR profiles of calcined supports (Fig. 2) have shown a lowest temperature of reduction for the more active catalyst. Whatever the Ti/Zr molar ratio is, the supports were not enough active for total toluene oxidation.
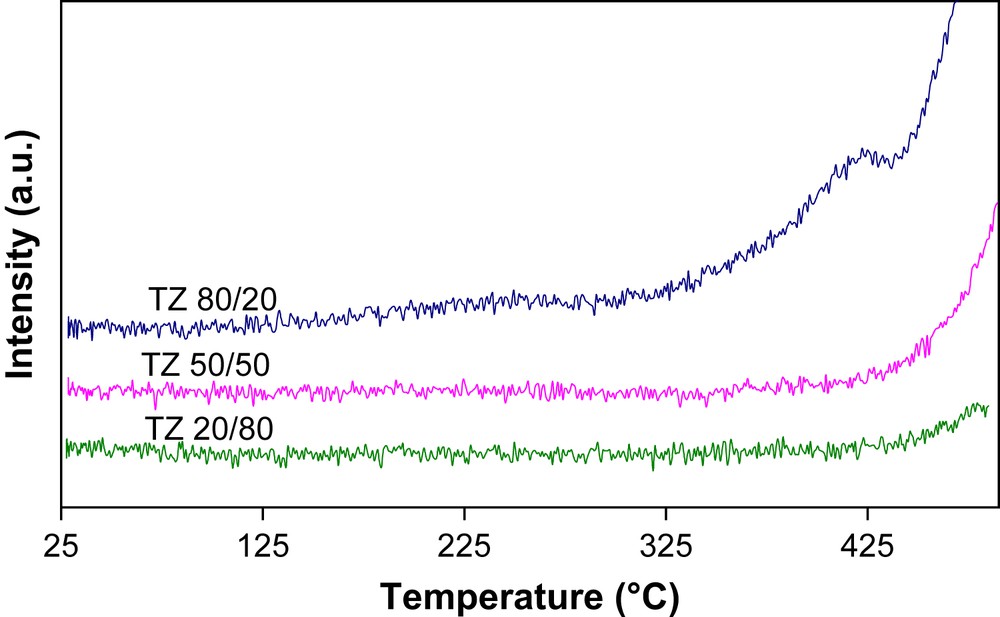
H2-TPR profiles of the calcined supports: TZ 80/20, TZ 50/50 and TZ 20/80.
The results of the catalytic activity measurements obtained for the catalysts on mesoporous titania–zirconia supported Pd and/or Au are shown in Fig. 3. It is important to note that, whatever the support used, the catalytic activity, given by the T50 values (Table 2), follows the same order: PdAu/TZ > 1.5Pd/TZ > AuPd/TZ > Pd/TZ > Au/TZ > TZ. The PdAu/TZ sample exhibits the highest catalytic activity in comparison with the other samples. For this catalyst, the complete toluene conversion is reached at 220 °C and it should be noted that for the same sample without precious metals, total oxidation is not observed under 400 °C. Depending on the order of the deposition of the palladium and gold, the PdAu/TZ sample shows significantly a higher conversion in comparison with the AuPd/TZ sample. In the case of all types of the TiO2–ZrO2, when palladium is loaded before gold the catalytic activities are lower in comparison with the samples, when gold is loaded firstly. The impregnation of palladium on Au/TZ is therefore, very interesting for VOC oxidation and there must be a synergetic effect between Pd and Au on the catalyst obtained. Gold can electronically influence the catalytic properties of Pd [17]. Thus, Hutching and co-workers [18] had correlated the high activity for H2 oxidation on AuPd/TiO2 catalysts to a core–shell morphology with a gold-rich core and a palladium-rich surface. The best activity for PdAu/TZ can be explained by this morphology and the bad activity of AuPd/TZ by an inverse core–shell.
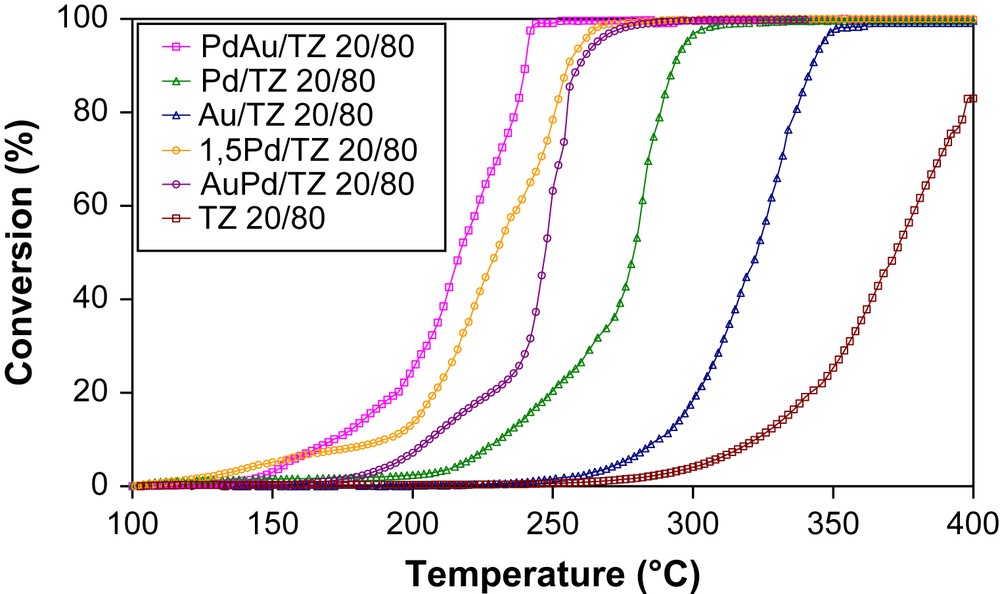
Conversion of toluene on the various catalysts.
T50 values for the samples.
Sample | T50% of toluene conversion (°C) | |
TZ 80/20 | TZ 20/80 | |
PdAu/TZ | 202 | 218 |
AuPd/TZ | 242 | 250 |
Pd/TZ | 251 | 280 |
Au/TZ | 340 | 309 |
1.5Pd/TZ | 225 | 231 |
TZ | 351 | 371 |
TPR profiles of the precious metal samples supported on mesoporous TZ 20/80 are shown in Fig. 4. For pure TZ 20/80 no peak is registered until 450 °C (Fig. 2). For the supported samples the first low temperature peak of hydrogen consumption was detected, which is assigned to the PdO → Pd° reduction at the surface of the catalysts. The negative peak at about 80 °C is due to hydrogen desorption on palladium [18]. The second positive peak in the range 100–160 °C should correspond to the reduction of PdO particles more dispersed and/or in the porous structure or oxygen on gold and also the reduction of a part of the support. The peak above 250 °C is connected to the reduction of the support. Moreover, the experimental H2 consumption is more than the theoretical prediction. Therefore, in comparison with the sample before impregnation, the reduction of mesoporous TiO2–ZrO2 mixed oxide in interaction with noble metal is observed at low temperature.
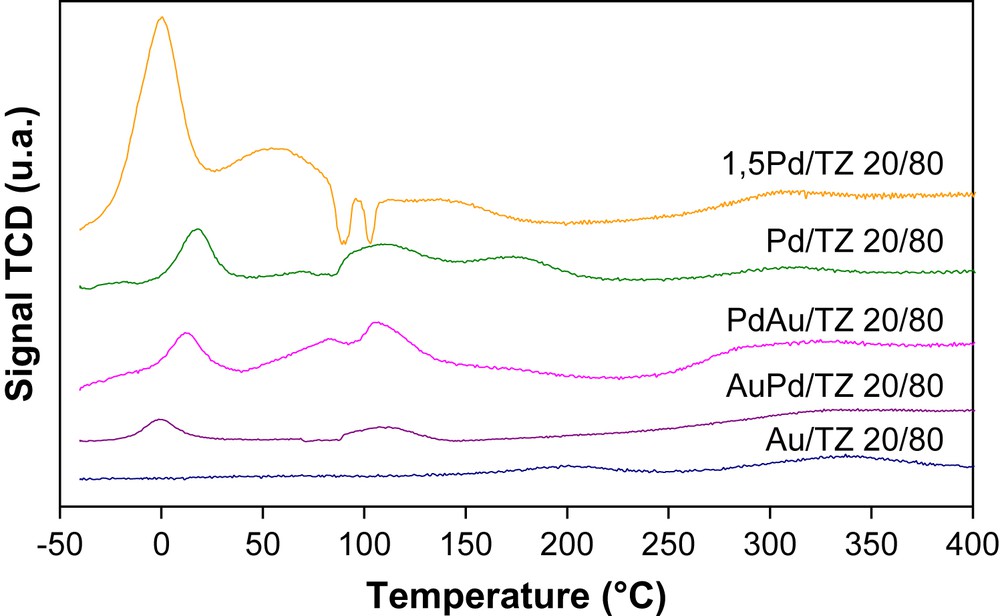
H2-TPR profiles of the calcined catalysts TZ 20/80.
“Operando” DRIFT was carried out for the toluene reaction on PdAu/TZ 80/20, Pd/TZ 80/20 and Au/TZ 80/20 samples. For the toluene reaction on PdAu/TZ 80/20 catalyst (Fig. 5.A), the peaks observed after the introduction of toluene at 1498 and 1526 cm−1 correspond to the aromatic νcc vibration as another band at 1596 cm−1 which appeared at 200 °C. The band at 1498 cm−1 corresponds exclusively to toluene whereas the bands at 1596 and 1526 cm−1 increasing until 300 °C and decreasing after this temperature, are also attributed to the formation of intermediate aromatic products during the toluene oxidation which stopped at high toluene conversion. Moreover, the broad band between 3100 and 3600 cm−1 corresponds to the O–H bond vibration (Fig. 5.B). The bands at 3025 cm−1 should be generated from the C–H bond vibration of methyl group of toluene. This band disappears at high temperature. The peaks observed at 3065 cm−1 which increase till 250 °C and then decrease with temperature, should correspond to the C–H bond vibration of toluene and aromatic intermediate products. The broad bands at 1656 cm−1 are generated at high temperature (over 250 °C) from new compounds or cokes during the reaction. Bellamy [19] has shown that this peak is characteristic of an aromatic poly-substitution cycle.
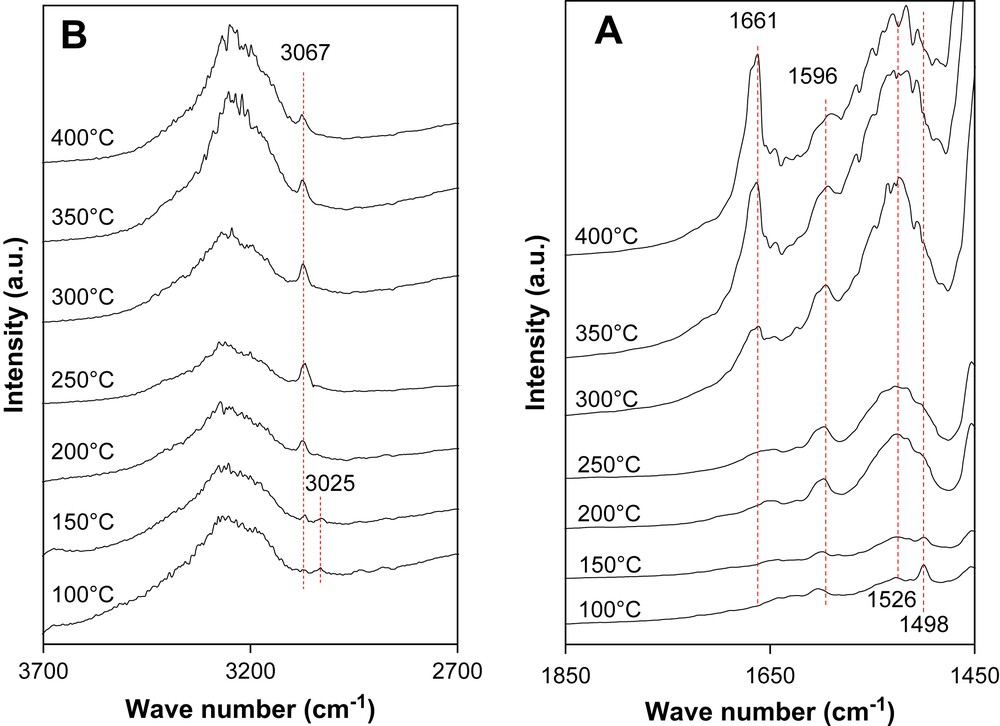
“Operando” DRIFT spectra of toluene on PdAu/TZ 80/20.
Results of the same type were found for both other catalysts for toluene oxidation. However, it was important to observe that, the toluene νcc absorbance was shifted to lower wave numbers: 1498 cm−1 for Pd/TZ 80/20 and 1426 cm−1 for Au/TZ 80/20. The shift of the wave number of the νcc band is correlated to the catalytic activity. This activity increases with the electronic density of the aromatic cycle of toluene, therefore when the interaction or adsorption between the aromatic cycle with the catalyst decreases. In fact, the adsorption of the VOC over noble metal supported catalysts has been studied by Paulis et al. [20] and by Ordonez et al. [21] and they studied the influence of the hydrocarbons adsorption processes on the oxidation reaction. These works showed that the lower adsorption of molecule on the catalyst the lower is the combustion temperature. The same tendency is observed for our catalysts.
The “in situ” calcination of the solids after the catalytic test was also studied. The intensity of the broad peaks corresponding to coke formed during toluene oxidation, decrease between 300 and 400 °C, by the combustion of this coke. This is in agreement with the results obtained by the EPR signal at g factor of 2.003 corresponding to coked catalyst.
Moreover, both the DTA exothermic signals at about 328 and 608 °C observed for the studied samples after the toluene tests (Fig. 6) should correspond to two types of hydrocarbon molecules, respectively light coke corresponding to (polysubstituted) monoaromatic compounds and more heavy coke to polyaromatics [22]. Moreover, the lowest weight loss between 100 and 700 °C was observed for our best PdAu/TZ catalyst (Table 3). The stability of the catalysts was evaluated for a toluene conversion of 15% (for a better viewing of the deactivation) but no deactivation was observed after 48 h.
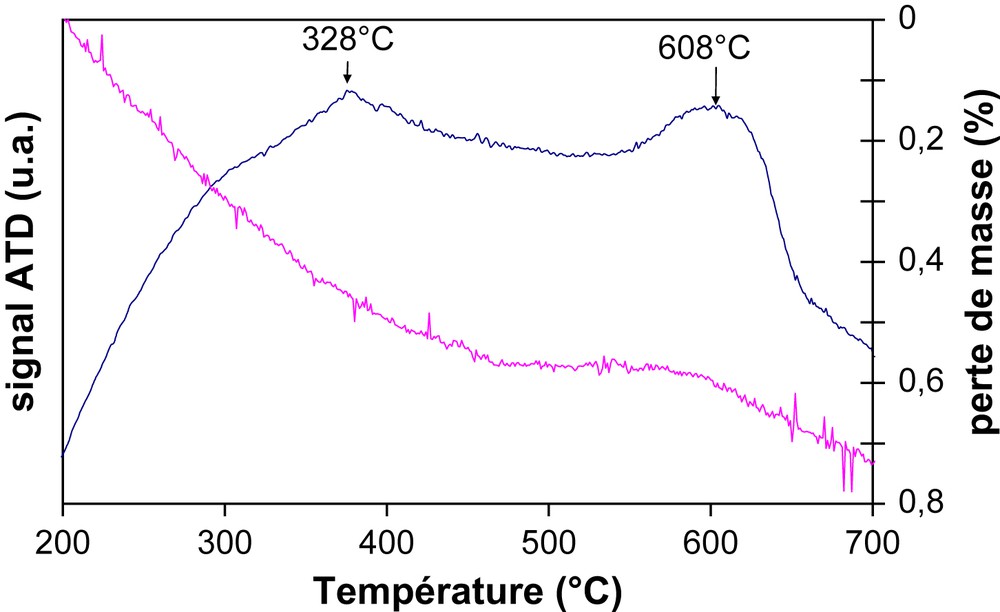
DTA–TGA profile of PdAu/TZ 80/20 under air.
Mass losses for the used samples between 100 and 700 °C under airflow.
Sample | Mass loss (%) |
PdAu/TZ 80/20 | 1.7 |
Pd/TZ 80/20 | 4.6 |
Au/TZ 80/20 | 3.9 |
4 Conclusion
Nanostructured mesoporous TiO2–ZrO2 mixed oxides with Ti:Zr mole ratios of 80/20, 50/50 and 20/80, are synthesised using a mixture of zirconium propoxide and titanium isopropoxide as Zr and Ti source and also CTMABr as surfactant. The new supports are impregnated by 0.5 or 1.5 wt% of palladium and 1 wt% of gold. Whatever the support used, the catalytic activity for toluene total oxidation follows the same order: PdAu/TZ > 1.5Pd/TZ > AuPd/TZ > Pd/TZ > Au/TZ > TZ. The promotional effect of gold added to palladium is then observed for the PdAu/TZ sample. Moreover, the hydrogen TPR profile of the catalyst shows that the experimental H2 consumption is higher than theoretically expected. Therefore, in comparison with the TPR profile before impregnation, we observe the reduction of the support in interaction with the noble metal at low temperature. Otherwise, “operando” DRIFT allows one to follow the VOCs oxidation, but also suggest an interaction between the adsorbed molecule and the catalyst, which decreases when the activity for oxidation reaction increases. Coke formation is also observed and its presence after test is also shown with DTA–TGA experiments by exothermic signals between 300 and 600 °C, by EPR with the signal at a factor g = 2.003 and a BET area decrease.