1 Introduction
Within the key perspective of process intensification, microstructured reactors appear as a highly promising technology [1] and perspectives of mass production are announced by technology providers [2,3]. These reactors are generally based on the assembly/stacking of microstructured metallic platelets coated with suitable catalysts. Due to their high heat and/or mass transfer, low pressure drop and good phase contacting, they are particularly adapted to the large domain of catalytic oxidation reactions. Specificities for catalyst wash coating, platelet assembly and operating conditions have been carefully considered for implementing correctly these new structured reactors [4]. However, a major challenge remains to investigate the catalytic process along the microchannels in view of further modelling and better control of the operating conditions. For that purpose, an access to effective gas and surface adspecies concentration profiles is required, rather than establishing it only by calculation. Until now, only some attempts have been reported to establish a gas concentration profile by sampling the effluents with a micro-capillary along structured reactors (in general monolith based), completed with a thermal mapping for detecting hot spots along the catalytic bed [5].
In this work, a new experimental reactor concept is described, which allows true surface analysis by Diffuse Reflectance Infrared Fourier Transform Spectroscopy (DRIFTS) measurements over a microstructured reactor, under reaction conditions through an adapted window without affecting the flow along the microchannels. The reaction chosen to validate this concept is the oxidation of carbon monoxide, which represents a key step in the process of hydrogen purification before feeding PEM fuel. A Pt/CeO2–Al2O3 catalyst, known to be active for that reaction, was selected for this preliminary operando DRIFTS analysis.
In order to evidence specificities of the microstructured system, the related results were compared to those obtained in a conventional fixed bed DRIFTS reactor with a similar catalyst in powder form and under closed operating conditions.
2 Experimental
2.1 DRIFTS reactors
2.1.1 Optically accessible DRIFTS microstructured reactor
Fig. 1A shows a schematic design of the optically accessible microstructured reactor. The catalyst is coated as a thin layer onto a microstructured metal platelet. This platelet is placed into a reactor housing designed to be adapted to a xy table of an Infrared optical microscope “Nicolet Continuum” linked to an infrared spectrometer “Nicolet Nexus” equipped with an MCT detector [6]. This set-up is connected to a conventional gas supply ensemble and an analysis set-up (micro-gas chromatograph Agilent 3000 instrument) equipped with a channel module used for H2, O2, N2, CH4 and CO measurements (MolSieve 5A, BF PPU Plot, with Ar as carrier gas) and a channel for CO2 and H2O (PPU, BF PPQ, with He as carrier gas). The housing can be heated up to 300 °C by electrical cartridges. Its design allows a laminar flow well distributed among the parallel channels, as demonstrated by DFT calculations (not reported here). The CaF2 window is directly in contact with the platelet, avoiding significant by-pass between the channels. By moving automatically the reactor under the infrared beam, the spatially resolved DRIFTS analysis is carried out along the reactor channels under reaction conditions. For this reactor, the optical length of the IR beam within the reacting zone, i.e. the geometrical distance between the reactor window and the catalyst adlayer, is evaluated to be about 0.5 mm (see Fig. 1). The IR signal was collected in the form of absorbance (log(1/R) transform) which is the most adapted to the present case of poorly absorbing adsorbates to obtain DRIFTS data proportional to the adsorbate surface concentrations [7]. Background single beam spectra were acquired by focussing on a catalyst free zone of the microreactor to account for all internal and external non-catalytic contributions. Though a minor specular reflection contribution cannot be discarded, it is assumed that the signal comes essentially from diffuse reflectance.

Design of the optically accessible DRIFTS reactors. (A): microstructured (in house), (B): fixed bed (from Spectratech®).
2.1.2 Fixed bed DRIFTS reactor
The DRIFTS analysis carried out at the surface of a fixed bed reactor loaded with the same catalyst but packed under powder form was performed by using an in situ DRIFTS cell from Spectratech [8] (Fig. 1B).
About 20 mg of powder catalyst sieved at 200–400 μm was loaded in the cell on a layer of silicon carbide to improve the thermal exchange within the fixed bed. A CO/O2/Ar mixture was passed over the catalyst at different temperatures.
For this reactor, the optical length of the IR beam within the reacting zone is evaluated to be about 10 mm. A partial by-pass of the fixed bed as indicated in Fig. 1 prevented full conversion to occur, as already stated by Meunier et al. [9]. However, the effective conversion levels were measured in a conventional tubular fixed bed reactor under the same operating conditions. The same mode of spectra acquisition was used as for the microstructured reactor.
2.2 Catalysts preparation and platelets coating for the microstructured DRIFTS reactor
The structured platelet used in this study was made of Aluchrom® (Iron–chromium–aluminium resistance alloy), cleaned and annealed at 1000 °C before coating. An alumina sublayer was deposited first by an optimized wash coating recipe (using a solution of acrylic acid mixed with a suspension of alumina, acetic acid and boehmite), followed by drying at room temperature and slow calcination (1 K/min) up to 1000 °C. Ceria was then impregnated from a solution of Ce(NO3)3 and the mixed oxide layer slowly calcined up to 550 °C. About 2 wt.% of platinum was then added by impregnation from a solution of Pt(NH3)4(OH)2 followed by a slow calcination (1 K/min) up to 550 °C [10].
2.3 Catalysts preparation for the fixed bed DRIFTS reactor
The powder catalyst used in the fixed bed DRIFTS reactor was prepared by a sol–gel method with a formulation close to the one used for the structured microreactor [11,14].
2.4 DRIFTS kinetic measurements and gas analysis of effluents
For both DRIFTS reactors (fixed bed or microstructured), the catalysts were reduced under a flow of 50% H2 in He from room temperature to 270 °C at 10 °C min−1 and kept 2 h at 270 °C before cooling under He.
In a typical catalytic test for investigating temperature effect, the reactors were heated from 25 °C to 300 °C, and eventually ramped down to room temperature. The DRIFTS spectra were recorded continuously and the bands' intensity calculated after proper background treatments.
2.4.1 Microstructured DRIFTS reactor
During catalytic testing for mapping gas and adspecies concentrations along the microstructured channel, the operating conditions (flow rates and temperature) were maintained constant and the microstructured reactor displaced regularly under the IR beam until the whole channel was investigated (6 analyses per channel). The gas inlet composition was fixed at CO:O2:He = 50%:25%:25%. This high concentration of reacting species was chosen to maximize the DRIFTS signals due to the short optical length (around 0.5 mm).
2.4.2 Fixed bed DRIFTS reactor
This reactor offering an optical length about 20 times larger than for the microstructured one, the gas inlet composition was fixed as either CO:O2:Ar = 0.5%:0.6%:98.9%, to maximize the absorbance of adsorbed species and minimize the one of gas phase reactants and products, or CO:O2:He = 50%:25%:25% to compare strictly with the conditions selected for the microstructured system.
During all these operations and for both DRIFTS reactors, the outlet gas concentration was analysed by the on-line micro-gaseous chromatograph (similar to the microstructured system).
3 Results
3.1 CO oxidation in the fixed bed DRIFTS reactor
The spectra obtained during the CO oxidation reaction in the fixed bed DRIFTS reactor on the Pt/CeO2–Al2O3 catalyst were acquired in the temperature range 20–300 °C by ramping up the system at 5 °C/min, with 8 min at each constant temperature interval (20, 50, 100, 150, 200, 250, 300 °C). They revealed two domains of temperature corresponding to two distinct states of the catalytic surface, as shown in Fig. 2.
- (i) from room temperature to about 180 °C, the band of CO linearly adsorbed on Pt phase at 2069 cm−1 (named L-CO in Fig. 2) remains rather narrow and stable, as well as the doublet of gaseous CO at 2100–2175 cm−1 (not reported in Fig. 2, due to its small intensity). In the mean time the doublet at 2360–2330 cm−1, characteristic of gaseous CO2 (named CO2 gas in Fig. 2), develops very slowly from 100 °C to above. Other adspecies characteristic of the basic ceria–alumina support (formates and carbonates adspecies) were also observed in this temperature range within the range 1622–1554 cm−1.
- (ii) from 180 to 300 °C, the doublet of gaseous CO and the linearly adsorbed CO band at 2065 cm−1 are no longer detected, while the gaseous CO2 doublet is now large and stable with temperature. This abrupt change in surface and gas concentration obviously reflects the light-off and run away of the CO oxidation, leading to the full conversion of CO into CO2 (at least for the flow crossing the reactor), as checked in a conventional fixed bed reactor without by-passing effect. No major changes were observed for the support adspecies.

Changes in CO conversion at reactor outlet (A) and in CO2 gas phase and linear carbonyls concentration (B) versus temperature for the Pt/CeO2–Al2O3 in the fixed bed DRIFTS reactor. CO:O2:He = 50:25:25.
This temperature light-off (leading to a higher temperature of the catalyst bed as compared to the reactor temperature) is confirmed by observing a marked hysteresis between ramping up and ramping down of temperature (A). This latter effect is the characteristic of a quasi autothermal system where the heat produced by the reaction overcompensates the heat losses by convection and/or conduction.
3.2 CO oxidation in the microstructured DRIFTS reactor
Fig. 3 illustrates the DRIFTS mapping along a central microchannel (every 6 mm) under reacting conditions (here at 300 °C). Due to the small optical length (0.5 versus 10 mm) and the small amount of reflecting material in that device, only the most pronounced bands (L-CO, gaseous CO and CO2 and adsorbed formates/carbonates lumped together) are quantified.

Changes in DRIFTS spectra from inlet to outlet in the microstructured reactor during the oxidation of carbon monoxide at 300 °C over Pt/CeO2–Al2O3.
Fig. 4 reports the changes in gaseous CO2 band intensity along the reactor axis for the Pt/CeO2–Al2O3 catalyst. This concentration is expressed as a local CO2 flow rate after calibration done at the reactor outlet where the effective CO2 flow rate (obtained by mass chromatography) is compared to the DRIFT response of the CO2 gas IR band.
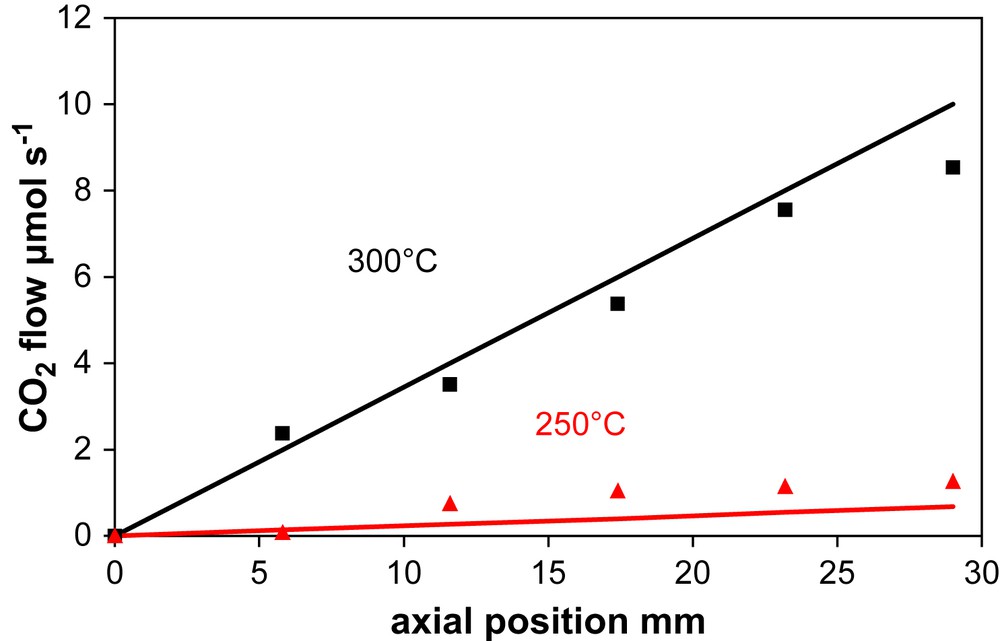
Experimental (symbols) and calculated (lines) CO2 concentration (expressed as local flow rate) profiles along a microchannel at 250 and 300 °C over the Pt/CeO2–Al2O3 catalyst.
Quasi linear increases of CO2 concentration with temperature are observed, whatever the temperature.
As seen in Fig. 5, in contrast with the previous linear increases in CO2 gas concentration along the microchannels, no significant change in surface concentration was observed for the main detected adspecies, within the uncertainty of spectrum acquisition. Note here that the latter was mainly due to: i) the weakness of the IR signal coming from the limited amount of reflecting material (see Fig. 3); and ii) the lack of homogeneity of the coated adlayer (thickness, conformity, microcracks, surface structural defects, etc), revealed by the optical microscope and quite in line with the spot resolution (50 × 50 μm). Thus, formates and carbonates adspecies were lumped together within the range 1622–1554 cm−1 and linear carbonyl adsorbed on platinum particles were detected within the range 2084–2053 cm−1. The above statement indicated a constant coverage of the catalyst all along the channels, within the explored temperature range (225–300 °C).

Changes in (A) formates and carbonates adspecies (lumped within 1622–1554 cm−1) and (B) linear carbonyl on platinum (2084–2053 cm−1) along the microstructured channels between 225 and 300 °C for the Pt/CeO2–Al2O3 catalyst.
3.3 Changes in conversion at reactor outlet
Fig. 6 reports the changes in CO conversion with temperature measured at the outlet of the DRIFTS microstructured reactor. At variance with the CO conversion curves obtained with the fixed bed DRIFTS reactor, no light-off effects (abrupt increase of conversion around 180 °C and hysteresis loop between ramp up and down temperature changes) were observed with the microstructured reactor: the conversion increased in a monotonic way, with the same values when ramping the temperature up and down, as expected from an isothermal and kinetically controlled process.

Changes in CO conversion with temperature at the outlet of the DRIFTS microstructured reactor for Pt/CeO2–Al2O3 catalyst. Experimental (symbols) and modelled (continuous line) data.
4 Discussion
4.1 Comparison between the microstructured and fixed bed DRIFTS reactors
As depicted in Figs. 2 and 6, thermal effects were observed only in the fixed bed DRIFTS reactor. This is a characteristic of a reaction light-off and run away (generally ascribed to heat transfer limitation occurring in non-isothermal systems), leading to full conversion of linear carbonyl adspecies on Pt particles, with the simultaneous disappearance of the gas phase CO and the abrupt increase in gaseous CO2 (Fig. 2). The two temperature domains observed experimentally correspond respectively to two distinct states of the active surface: i) below 180 °C, full coverage of Pt particles by linear carbonyls, strongly inhibiting the oxygen activation; and ii) above 180 °C, the Pt particles are mainly covered with activated oxygen, immediately reacting with CO as soon as colliding with the Pt surface.
In contrast, over the whole 25–300 °C temperature range, the microstructured reactor leads to a monotonous change in CO conversion with temperature (Fig. 6). This demonstrates the excellent heat transfer in this microstructured system which prevents any run away of the CO oxidation, confirming this unique property of microstructured reactors [1,4].
4.2 Specificity of the Pt/CeO2–Al2O3 catalyst in the microstructured reactor
As demonstrated by the DRIFTS analysis carried out in the microstructured reactor (illustrated in Fig. 5), the coverage of platinum particles by linear carbonyls remains high all over the explored reacting conditions (little changes between room temperature and 300 °C), due to the efficient heat transfer with the metallic substrate, evacuating continuously the heat produced by the CO oxidation. Therefore, it can be inferred that under these conditions, no Pt sites are available for oxygen activation, which means, as generally agreed in the literature [13], that the main source of active oxygen is ensured by ceria, able to activate gaseous oxygen for feeding continuously the Pt/ceria interface where the CO oxidation can proceed. Note that the proper intrinsic activity of the ceria/alumina support has been found negligible from specific experiments not reported here.
4.3 Modelling of CO oxidation over Pt/CeO2/Al2O3 in the microstructured reactor
Campman [12] and Nibbelke et al. [13] studied extensively CO oxidation over a three-way Pt/CeO2/Al2O3 catalyst. They proposed first the following mechanism on the Pt phase:
COg + ∗ ⇌ CO∗ | (1) |
O2,g + ∗ → O2∗ | (2) |
O2∗ + ∗ → 2O∗ | (3) |
CO∗ + O∗ → CO2,g + 2∗ | (4) |
Assuming that the O2 adsorption is rate-limiting and that the CO adsorption is equilibrated give the following rate equation:
(5) |
The priori values of the parameters for Pt, mainly from surface science studies (Table 1), were substituted in rate Equation (5) and incorporated into a one-dimensional plug flow reactor model. The corresponding differential equation was integrated numerically over the reactor length and the value of k2f was adjusted within the range of values given in Table 1 to give an adequate description of the experimental conversions, as shown as the solid line in Fig. 6.
A priori values of the kinetic parameters for Pt.
Parameter | Value | References |
Lt | 0.04 mol/kgcat (∼60% dispersion) | Germani et al. [14] |
k2f | 0.2–2 × 107/bar s (s0 ≈ 0.01–0.1) | Luntz [15] |
E2f | 0 kJ/mol | Herz and Marin [16] |
K1 | 2 × 10−6/bar s | Zhdanov et al. [17] |
E1 | −130 kJ/mol | Herz and Marin [16] |
Actually, as stressed above, the specificity of ceria promoted Pt/CeO2/Al2O3 is to offer a second route for oxygen activation over the ceria surface in parallel to the main route of oxygen activation on the Pt phase. It can be formalized by adding the following elementary steps to the above mechanism restricted to the Pt phase:
O2,g + s → O2s | (6) |
CO∗ + O2s → CO2,g + Os + ∗ | (7) |
CO∗ + Os → CO2,g + s + ∗ | (8) |
CO2 + s ⇌ CO2s | (9) |
For the present study, due to a lack of kinetic data, the simplified mechanism based on steps (1)–(4) was considered for calculating the CO conversion over the Pt/CeO2–Al2O3 at the reactor outlet. Fig. 6 shows the CO2 flow as a function of the axial position at 2 different temperatures as calculated by rate Equation (5) (line) and the experimental IR CO2 bands (points). The adequacy of the chosen simplified model is confirmed for these axial profiles.
One can note here that the above modelling of the CO oxidation along the platelet channels is assuming isothermal conditions, which confirms the excellent heat transfer between the catalytic sites and the metallic substrate.
5 Conclusions
This preliminary study was carried out to demonstrate the potential of an original DRIFTS reactor that was designed for characterizing the “operando” behaviour of microstructured platelets (element of a stacked microreactor). Its unique ability to map the surface adspecies and gas concentration by means of the DRIFTS/microscope analysis was used for establishing a kinetic model of the CO oxidation able to describe correctly the experimental data corresponding to this mapping.
Comparing the CO conversion changes with temperature between this new tool and a conventional fixed bed DRIFTS reactor brought further evidence of the exceptional heat management of the microstructured reactor (isothermal behaviour without heat transfer limitation) while the fixed bed one was much more adiabatic (leading to quasi autothermal conditions due to major heat transfer limitation).
Thus, despite the drawback of a set-up difficult to handle (combining prototype and commercial units), this tool offers a unique advantage for advanced operando kinetic studies. Further works are in progress to enlarge its field of application.