1 Introduction
The ever increasing demand for lighter engine fuel and the higher price of light crude oil has stimulated the petroleum refining industry into looking for the possibility of processing heavy fractions as well as cheaper heavy crude oil. In this context, hydrocracking process is of a major interest for the refiners. Hydrocracking catalysts are bifunctional associating a hydro–deshydrogenating function with an acidic one. The cracking activity is mainly controlled by the support. For supported catalysts, various supports as zeolithe [1], MCM [2] or ASA (amorphous silica alumina) [3,4] supported catalysts have been reported in the literature and among these supports, ASA possesses a moderate acidity which allows one to obtain an optimal gas oil yield. Since the feeds to be treated contain significant amounts of sulfur and nitrogen, the catalysts must resist poisoning by hydrogen sulphide and ammonia. The hydro–deshydrogenation function is then provided by mixed sulfides of W and Ni and the active phase consists in well-dispersed WS2 nanocrystallites decorated with Ni atoms. This active phase is obtained through the sulphidation of an oxide precursor prepared by incipient wetness impregnation of ASA with an aqueous solution containing the elements to be deposited. To improve these catalysts, this work focuses on the interest of new starting materials for the preparation of the impregnating solutions, instead of ammonium metatungstate and nickel nitrate salts. Different heteropolytungstates, with Anderson, Keggin or its derived lacunary based structures have been prepared and characterized to be used as starting materials for the oxide precursors preparation. In particular, heteropolyanion (HPA) nickel salts have been prepared and impregnated on an ASA support to provide catalysts with varying Ni/W ratio, the WO3 content being maintained at 12 wt%. All the HPA based catalysts were fully characterized in order to monitor the structure of oxide precursors as well as the dispersion of the active elements, at each step of preparation. Finally, hydrogenation activity of HPA based catalysts was also compared to the conventional preparation.
2 Experimental
2.1 Preparation of the Anderson HPA: NiW6O24H64−
The Anderson ammonium salt (NH4)4NiW6O24H6 was obtained according to literature data by precipitation from an aqueous solution of sodium tungstate, nickel sulfate and ammonium chloride at pH = 6 [5]. The recrystallization allows one to purify this ammonium salt. The Ni/W ratio (1/6) was checked by chemical analysis.
2.2 Preparation of the Keggin-type HPA nickel salts
The starting materials were the well-known pure heteropolyacids H3PW12O40 and H4SiW12O40 prepared according to the literature data [6,7]. The first step was the preparation of the barium salts in solution by ionic exchange of the acidic protons by barium cations. The nickel salts of the Keggin HPA PW12O403− and of its derived lacunary HPA PW11O397− (obtained by removing one WO6 octahedron) were then obtained by a successive ionic exchange between barium and nickel cations.
The exchanges proceeded according to the following equations for the Keggin compound:
(3H+, PW12O403−) + (1.5 Ba2+, 3 OH−) → (1.5 Ba2+, PW12O403−) + 3H2O |
(1.5 Ba2+, PW12O403−) + 1.5 (Ni2+ + SO42−) → (1.5 Ni2+, PW12O403−) + 1.5BaSO4 |
The nickel salt of the lacunary HPA PW11O397− was also obtained following the same preparation procedure. The formation of the lacunary specie was due to the addition of 3.5 equivalent of barium hydroxide (instead 1.5). Indeed the pH of the solution raised to 3 and the more stable HPA specie was PW11O397−. This procedure is shown in Fig. 1.
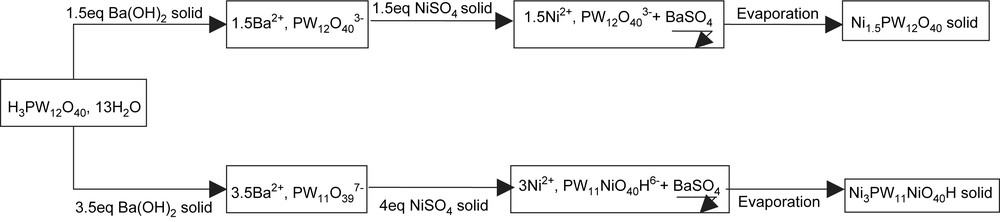
Synthesis scheme for the preparation of the nickel salts of Keggin based heteropolyanions.
The impregnating solutions were directly prepared, containing only Ni ions and the corresponding HPA. BaSO4 was eliminated by filtration of the precipitate. The bulk compounds were afterwards obtained by evaporation.
2.3 Preparation of catalysts
Catalysts were prepared by incipient wetness impregnation of ASA support with Keggin HPA based solutions. Unfortunately the solubility of the ammonium Anderson compound is too low to prepare catalyst with a suitable tungsten loading. No further study was then performed on this solid. Reference NiW solids having the same metal loadings were also prepared for comparison purposes by using the conventional impregnating solutions of ammonium metatungstate (NH4)6H2W12O40 and nickel nitrate Ni(NO3)2. The solids were dried overnight at 100 °C and then calcined at 500 °C under oxygen. Oxide precursors were then sulfided in a glass vial at atmospheric pressure under a H2S/H2 mixture with a p(H2S)/p(H2) ratio about 0.17 and a gas flow of 2 L/h/g of catalyst. The samples were heated under the sulfiding mixture at a rate of 5 °C/min up to 350 °C and maintained at this temperature for 2 h. They were then cooled down to room temperature under the reactive mixture.
Table 1 lists the different nickel salts, the corresponding Ni/W ratio – which was checked by chemical analysis – nomenclature of new materials based and reference catalysts. The quantity of WO3 loading was chosen at 12 wt% because it is the maximum loading obtained for the lowest soluble Keggin HPA nickel salt.
Description of the catalysts prepared using the new starting materials or conventional impregnating solutions.
Catalysts nomenclature | Precursor | Ni/W | WO3 loading (wt%) |
A | Ni3/2PW12O40 | 0.125 | 12 |
B | Ni2SiW12O40 | 0.17 | 12 |
C | Ni3PW11NiO40H | 0.36 | 12 |
RefA | (NH4)6H2W12O40 + Ni(NO3)2 | 0.125 | 12 |
RefB | (NH4)6H2W12O40 + Ni(NO3)2 | 0.17 | 12 |
RefC | (NH4)6H2W12O40 + Ni(NO3)2 | 0.36 | 12 |
2.4 Characterization techniques
FT-IR: Spectra were recorded using a Nicolet 510 Fourier Transform IR spectrometer. The samples were analyzed in the 200–4000 cm−1 spectral range using the KBr pellet technique with 1 wt% of the sample in KBr.
Raman: The Raman spectra of the samples, maintained at room temperature, were recorded using a Raman microprobe (Infinity from Jobin-Yvon), equipped with a photodiode array detector. The exciting light source was the 532 nm line of a Ar YAG laser and the wavenumber accuracy was 2 cm−1. Due to the intense lines of ASA supports, only the 800–1200 spectral range characteristic of the W–O stretching mode is presented here.
TEM: Transmission electron microscopy studies were performed on a TECNAI electron microscope operating at an accelerating voltage of 200 kV. The sample was dispersed in ethanol and then deposited on carbon films supported on copper grids.
2.5 Catalytic testing
Toluene hydrogenation test was conducted at 623 K and 6 MPa. The liquid feed contained 73.6 wt% cyclohexane (used as solvent), 20.0 wt% toluene, 5.9 wt% dimethyldisulfide, used as sulfiding agent and 0.5 wt% aniline. Aniline was present to inhibit the acid function in order to avoid isomerisation reactions. This test was proceeded to evaluate and to compare the hydrogenation function of each prepared catalyst. The H2/feed ratio was constant and equal to 450 Nl/l. The liquid feed flow was 16 cm3 h−1 (LHSV of 4 h−1) during the sulfidation stage and 8 cm3 h−1 (LHSV of 2 h−1) during the catalytic test. Catalyst balls were filtered and their diameter did not exceed 3 mm. This fraction was diluted in solid SiC (4 cm3 for 4 cm3 of catalyst). After sulfidation (2 °C min−1 followed by 2 h at 350 °C), conversions were determined by the analysis of effluent through on-line gas chromatography every 45 min after the first measurement. Catalytic results were expressed in terms of toluene hydrogenation (HYD) reaction rates, calculated from the measured toluene conversions (hydrogenation products).
3 Results and discussion
The experimental Ni/W ratios reported in Table 1 were in agreement with those expected from the preparation. Fig. 2 showed the Raman spectra of nickel salts Ni3/2PW12O40, Ni2SiW12O40, Ni3PW11NiO40H and of (NH4)4NiW6O24H6. In this figure are also reported Raman spectra of the starting materials H3PW12O40 and H4SiW12O40 whose lines characteristic of the W–O terminal stretching mode are observed at 1011 and 998 cm−1 respectively, in agreement with literature data [8,9]. Raman spectra of the nickel salts indicated that the Keggin structures PW12O403− and SiW12O404− were preserved after the ionic exchange by comparison with spectra of the corresponding heteropolyacids. 31P NMR (spectra not reported here) analysis confirmed this result with the chemical shift observed at −15.4 ppm for Ni3/2PW12O40 in agreement with values already reported for the PW12O403− Keggin entities [10]. In Fig. 2-f corresponding to NiW6O24H64− ammonium salt, the main line corresponding to W–O terminal vibration mode is observed at 947 cm−1; this feature and the whole spectrum (not presented here) are characteristic of Anderson HPA structure (Tungsten or Molybdenum based) as already observed for Al(Co)Mo6O24H63− [11]. The nickel salt of PW11NiO40H6− did not exhibit the same line as observed for Ni3/2PW12O40 but presented a characteristic line at 989 cm−1 corresponding to the W–O terminal vibration mode of the HPA. In the preparation procedure (Fig. 1), when pH was raised with the addition of 3.5 equivalent of barium hydroxide, the Keggin anion which is kinetically stable only at pH < 3, was then converted into the so-called lacunary anion. In Fig. 2-d a slight extra-peak at 988 cm−1 is observed indicating probably the presence of some lacunary species SiW11O398−. Their formation could be explained by the preparation procedure and local pH increase in the solution during barium hydroxide addition.
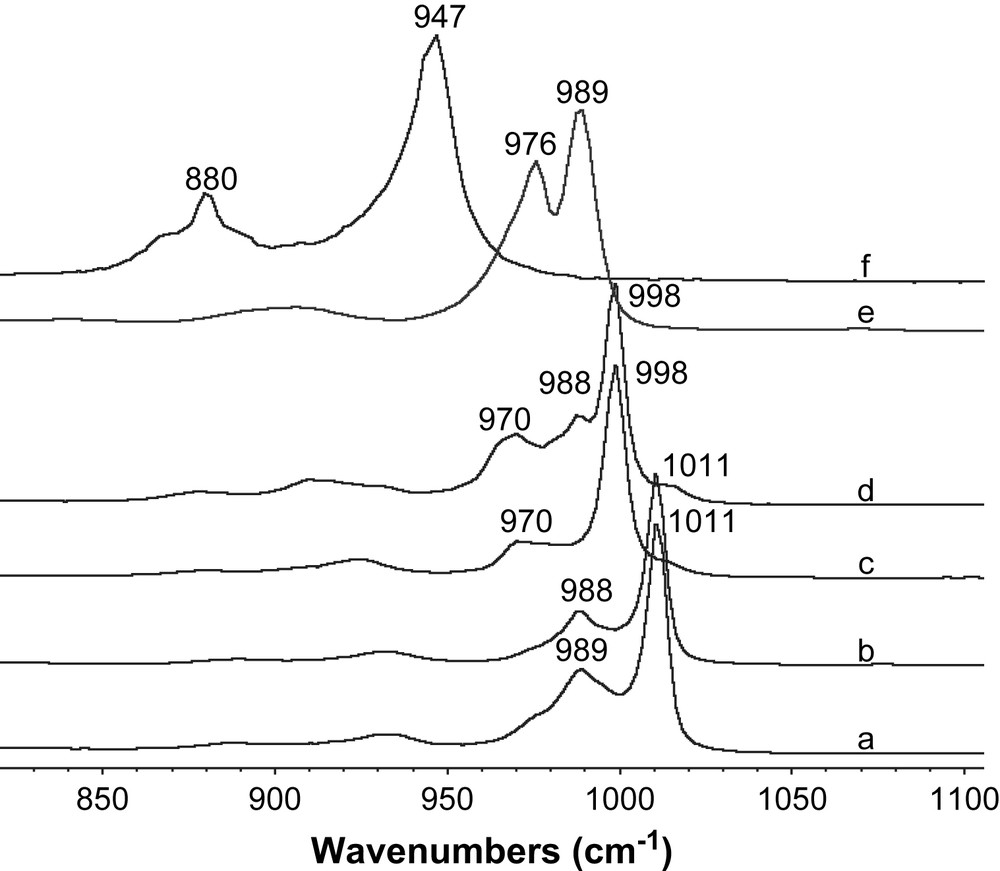
Raman spectra of H3PW12O40 (a) Ni3/2PW12O40 (b) H4SiW12O40 (c) Ni2SiW12O40 (d) Ni3PW11NiO40H (e) and (NH4)4NiW6O24H6 (f).
IR analyses allowed us to follow the preparation procedure associated to Ni3PW11NiO40H. In Fig. 3 are reported the IR spectra of the barium and nickel salts of the lacunary HPA and the nickel salts of PW12O403− and SiW12O404−. Typical absorption bands of such anions were observed in the range 500–1100 cm−1 [8]. The spectrum of the Ba7/2PW11O39 salt (Fig. 3-c) shows two bands at 1089 and 1044 cm−1 assigned to the vibration ν P–O of the central PO4 tetrahedron. In fact, the prominent band observed at 1080 cm−1 (Fig. 3-b) for the ν P–O vibration in Keggin HPA PW12O403− splits into two components due to the symmetry decrease of the PO4 tetrahedron in PW11O397−. In the IR spectrum of the corresponding nickel salt, the observation of one band at 1062 cm−1 clearly indicates that Ni cations restore the symmetry of the central tetrahedron and are inserted in the HPA structure to form the PW11NiO40H6− anion [12]. FT-IR spectrum 3-a (Fig. 3-a) is characteristic of SiW12O404− HPA in agreement with literature data [8], showing again that this HPA is not modified during ionic exchange to form its nickel salt.
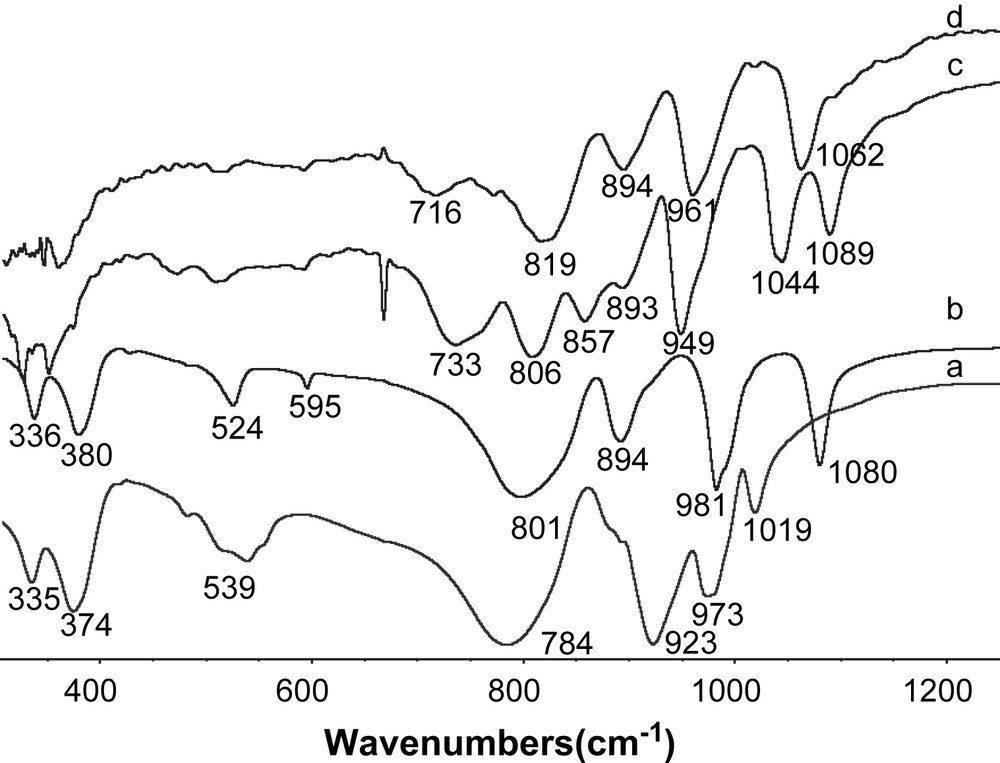
IR spectra of Ni2SiW12O40 (a) Ni3/2PW12O40 (b) Ba7/2PW11O39 (c) and Ni3PW11NiO40H (d).
The aqueous solutions of the nickel salts Ni3/2PW12O40, Ni2SiW12O40, Ni3PW11NiO40H have been used to prepare ASA supported catalysts. Characterization of the catalysts at each step of the preparation by Raman spectroscopy allowed us to follow the evolution of the starting materials upon drying and calcination (Figs. 4 and 5). A catalyst prepared from conventional starting materials ammonium metatungstate and nickel nitrate was also studied for comparison purposes.
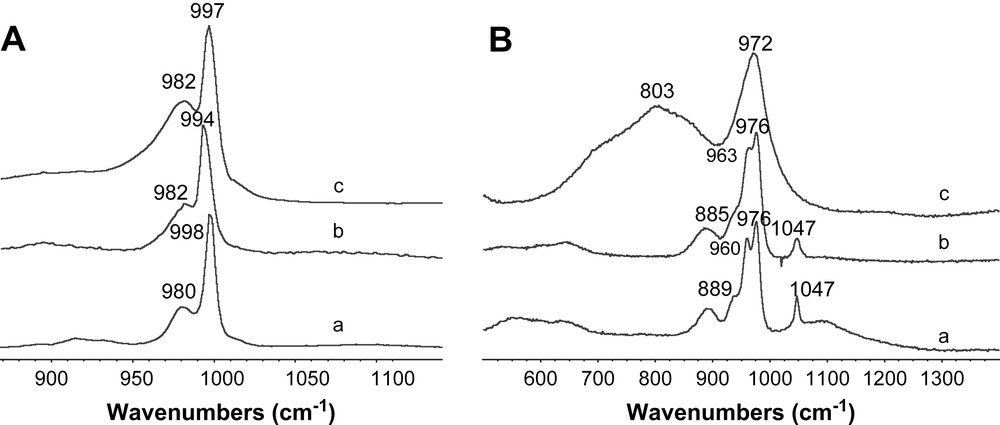
Raman spectra of Ni2SiW12O40 (A) impregnating solution (A,a), B catalyst after drying (A,b), B catalyst after calcination (A,c), and of conventional catalyst (B) impregnating solution (B,a), ref B catalyst after drying (B,b) and ref B catalyst after calcination (B,c).
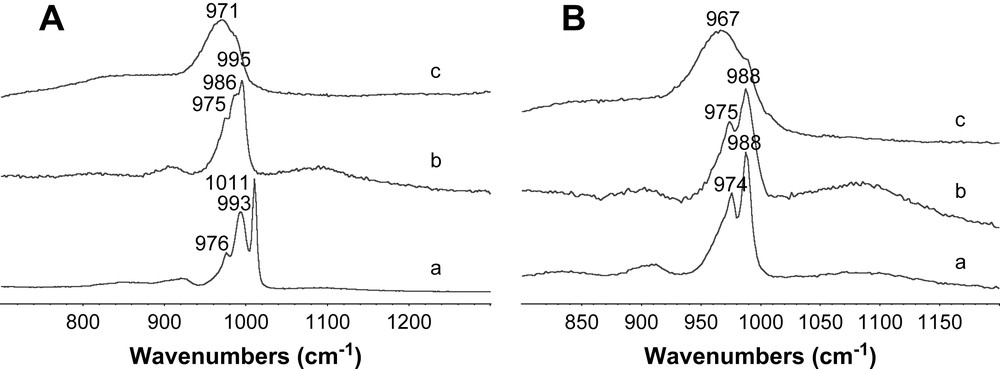
Raman spectra of Ni3/2PW12O40 (A) impregnating solution (A,a), A catalyst after drying (A,b), A catalyst after calcination (A,c), and of Ni3PW11NiO40H (B) impregnating solution (B,a), C catalyst after drying (B,b) and C catalyst after calcination (B,c).
The Raman spectra of the conventional (Fig. 4-B,b), Ni2SiW12O40 (Fig. 4-A,b) and Ni3PW11NiO40H (Fig. 5-B,a) dried catalysts present almost the same lines as the starting material (Figs. 4-B,a, A,a and 5-B,a). Only weak broadening and shift due to support effect were observed indicating HPA entities preservation on the ASA support after drying. However Ni3/2PW12O40 features (Fig. 5-A,a) are not clearly observed in the corresponding dried catalyst (Fig. 5-A,b). After calcination, the large band around 967–972 cm−1 is attributed to the formation of a well-dispersed polytungstate phase for Ni3PW11NiO40H, Ni3/2PW12O40 (Fig. 5-A,c, B,c) and the conventional catalyst (Fig. 4-B,c). Raman spectrum of Ni2SiW12O40 calcined catalyst is rather different and one can observe the SiW12O404− HPA features. SiW12O404− entities which are destroyed during calcination at 500 °C are certainly re-formed after thermal treatment and transfer in air.
The toluene hydrogenation test was performed on catalysts described in Table 1. Results are reported in Fig. 6 and demonstrate that A, B and C catalysts were active in toluene hydrogenation. As observed for conventional catalysts, the activity of the HPA based catalyst increased when the Ni/W ratio was raised. Moreover, HPA based catalysts which contain phosphorus are slightly more efficient as the reference ones.
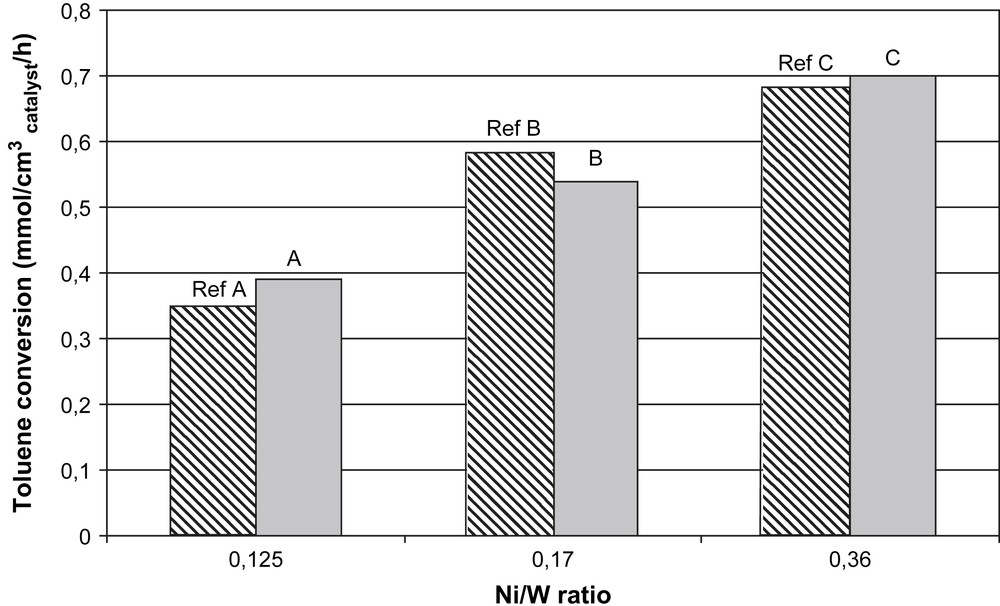
Hydrogenation activity of catalysts prepared with conventional impregnating solutions (hatched) and HPA nickel salts (bicolore).
Transmission electron microscopy (TEM) was used to evaluate the morphology of the WS2 crystallites obtained after sulfidation. Taking into account the catalytic results, we focused this study on Ni3/2PW12O40 and Ni3PW11NiO40H catalysts and the corresponding conventional catalysts with Ni/W = 0.125 and Ni/W = 0.36. As already mentioned in the literature [13] one can observe an inhomogeneous distribution of the WS2 slabs on ASA support. A typical TEM micrograph is shown in Fig. 7 that corresponds to Ni3PW11NiO40H catalyst. The average slab lengths and stacking degrees of catalysts are listed in Table 2. These results show that the average length and stacking of the HPA based catalysts were higher than those obtained for the conventional ones. However, whatever the material is, the average length (between 14.6 and 20.8 Å) is smaller than values reported for WS2 on ASA support [13] and for MoS2 supported phase [14]. As shown in Fig. 8 for Ni3PW11NiO40H catalyst whose slabs are the largest for this study, the majority of the slabs have a length between 10 and 20 Å. This is also observed for reference catalyst C.
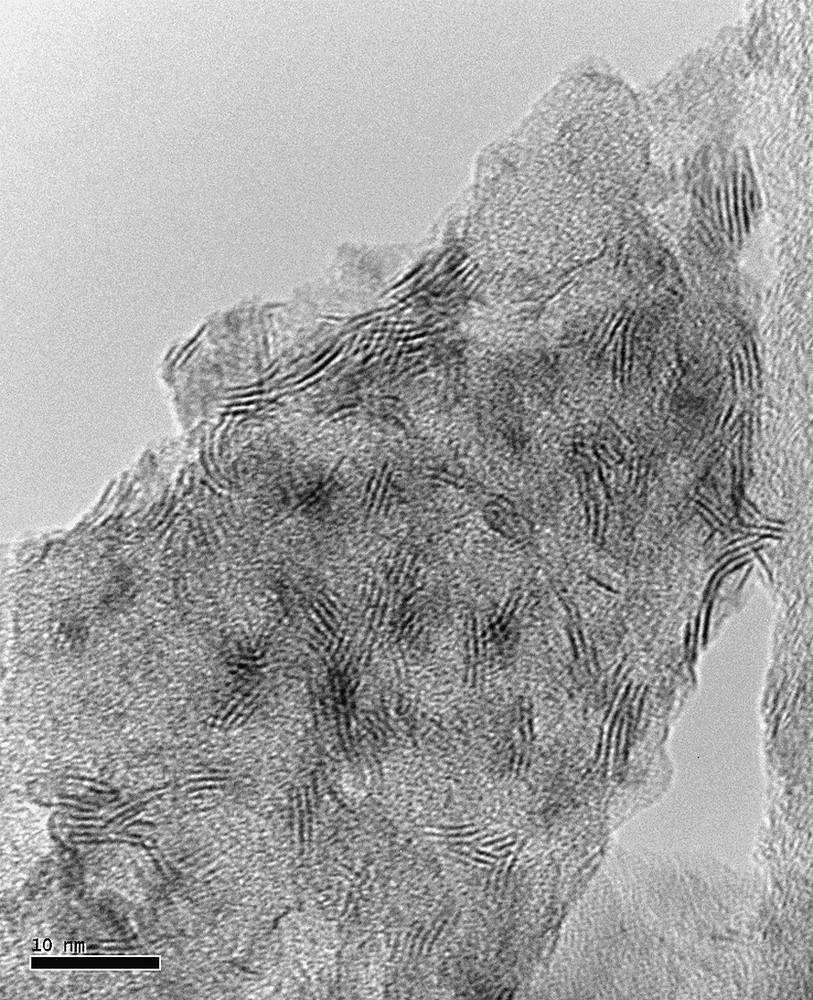
TEM picture of Ni3PW11NiO40H catalyst.
Average slab length, average stacking degree of studied catalysts.
Catalysts nomenclature | Average slab length (Å) | Average stacking degree |
A | 17.3 | 1.23 |
RefA | 14.6 | 1.19 |
C | 20.8 | 1.53 |
RefC | 17.9 | 1.49 |
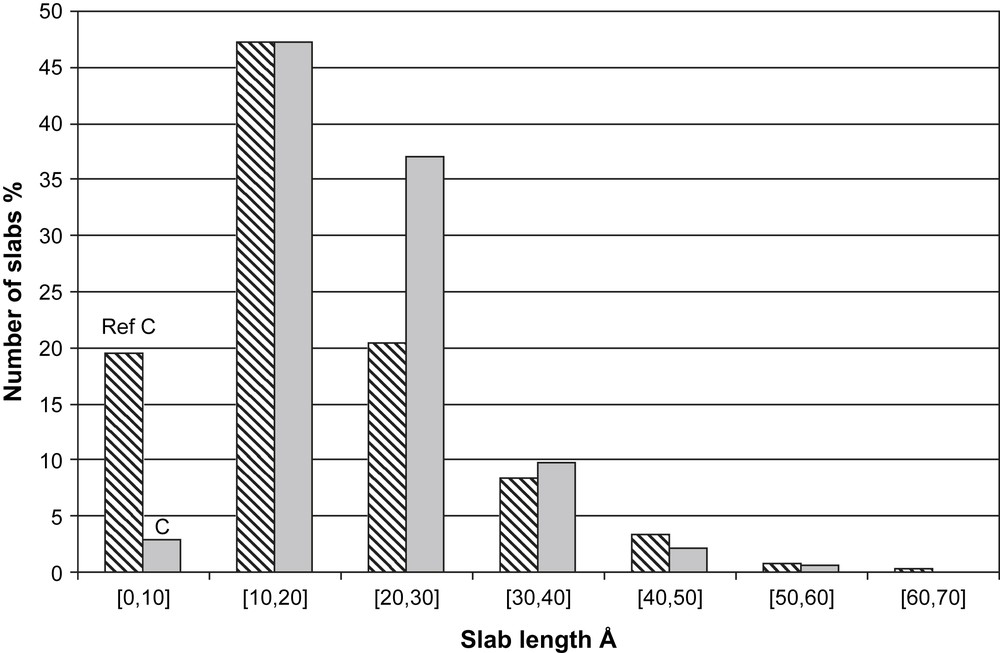
Slab length distribution of Ni3PW11NiO40H catalyst (bicolore) and its corresponding conventional catalyst (hatched).
The dispersion of the WS2 crystallites seems to be better in conventional catalysts and does not explain the catalytic results. As it was already reported for CoMo catalyst [15,16], the efficiency of HPA catalyst could arise from the promoting effect that is enhanced by the proximity of Ni and W in HPA nickel salt used in the impregnating solution.
4 Conclusion
In this work, well-characterized heteropolytungstate nickel salts were used to prepare supported ASA hydrocracking catalysts. Each step of catalyst preparation was followed by Raman analysis: in most catalysts, HPA are preserved after drying and a well-dispersed polytungstate phase is present after calcination except for Ni2SiW12O40 where SiW12O404− HPA could re-form after calcination and transfer in air. Hydrogenation activity of HPA based catalysts was also compared to conventional preparation. Results showed that new starting materials based catalysts were almost equivalent to their reference prepared by using the conventional impregnating solutions of ammonium metatungstate and nickel nitrate. A slight increase is observed by using Ni3/2PW12O40 and Ni3PW11NiO40H catalysts. TEM results showed that WS2 dispersion in conventional catalysts was higher than the dispersion observed in HPA catalysts. In these catalysts, a better promoting effect could explain the catalytic results. It could be due to the use of the HPA nickel salt which allows a proximity between W and Ni.
Nevertheless, further characterizations of the sulfided solids are under progress to better understand the influence of the use of these new HPA starting materials on their catalytic behavior.