1 Introduction
Numerous archaeological organic materials, precious remains of past society history, are available in Museums, in public or private collections, or are discovered in novel excavations: residues stored in jars, embalming material, waterproofing agents, adhesives, resins, etc. Their identification is not always possible based on sole interpretation at the macroscopic scale, which is an active area of research in Human Sciences and many questions have been shelved. A recently developed way to assess their composition, their degree of alteration and to get some insight into their history is to proceed to their molecular and isotopic analysis in order to enhance our understanding of knowledge and technologies of the past.
Various classes of raw organic materials have been exploited since ancient times to be incorporated into artefacts: bitumen, animal fat, beeswax, resin, vegetal tar, etc. Easily accessible, they were exploited for their useful properties: water insolubility, plasticity, aesthetic or medical properties. The chemical analysis of these natural substances which are complex molecular mixtures presenting wide ranges of molecular weight, volatility and polarity, is a real analytical challenge. The wide range of materials used is an additional difficulty, both because of the nature of components and their proportions in mixtures and because of the various preparation techniques. Moreover, alteration processes emphasize this complexity by modifying the initial molecular fingerprint. The alteration is both linked to human handling (heating) and to natural ageing during their long history (photochemistry, oxidation, biodegradation). It is thus clearly established that a better understanding of these interactive alteration processes is crucial to improve both preservation and restoration of archaeological artefacts.
This paper describes how molecular and isotopic techniques commonly used in petroleum geochemistry can be applied to the analysis of organic substances used by past societies. The chemical approach consists in the identification of diagnostic biomarkers [1–3], derived from naturally occurring organic substances. Biomarkers are compounds which have a carbon skeleton specific enough to be related to their biological lipid precursors, thus enabling the identification of the natural substances used. These compounds should ideally be resistant to the various alteration processes undergone by materials (thermal treatment, abiotic oxidation, biodegradation, etc.). The analytical procedures mainly described in the literature for the characterisation of organic residues present on art and archaeological items are generally carried out on raw samples, without any previous fractionation [4–8]. These techniques serve to determine main classes of components within complex mixtures and are useful to obtain preliminary data which have to be confirmed by further analyses.
The procedure presented in this paper describes a detailed characterisation of the overall composition of samples. Fractionation enables concentration of compounds, some of which are present in minute amounts and therefore are not detected by other procedures. Such minor compounds may have a key value to characterise a sample's origin or degree of alteration. Biomarkers were identified by gas chromatography–mass spectrometry (GC–MS) and high performance liquid chromatography–mass spectrometry (LC–MS). Although it is possible to characterise archaeological materials by their molecular composition alone, gas chromatography-combustion-isotope ratio mass spectrometry (GC-C-IRMS) is often an important complementary technique which provides more accurate information on the sources of archaeological organic matter [9–11].
The results provided by molecular and stable carbon (13C/12C) isotopic studies of two different series of samples are presented and discussed in this paper:
- - Dammar resins (Khao Sam Kaeo, Thailand, 4th B.C.–2nd B.C.). These resins, used for centuries as waterproofing agents, have played an important economical role in Southeast Asian countries. Their identification on archaeological remains is often contentious.
- - Organic deposit on Osiris statuettes (Pharaonic Egypt). Contrary to materials used for embalming human bodies, little is known about the mixture used for funerary material (e.g. for these statuettes).
This paper will show how chemical data obtained on these samples give in fine details about the history of these ancient organic materials (nature, production techniques, alteration state, trade networks).
2 Experimental
The analytical flow chart [12] is directly adapted from the methodology used in petroleum geochemistry to analyse bitumen, source rocks and crude oils.
The organic sample was extracted ultrasonically three times with a mixture of dichloromethane/methanol (1:1 v/v). The analyses of separated fractions were performed using gas chromatography–mass spectrometry (GC–MS), high performance liquid chromatography–mass spectrometry (LC–MS) and gas chromatography-combustion-isotope ratio mass spectrometry (GC-C-IRMS) after splitting the total organic extract into fractions according to different polarities by SiO2 column liquid chromatography (Fig. 1, Table 1). This analytical flow chart allowed us to isolate aliphatic and aromatic hydrocarbons, esters, ketones, triacylglycerols, alcohols and acids.

Fractionation scheme used for organic samples (CC: column liquid chromatography; TLC: thin layer chromatography).
Relative yields of the different fractions of samples 2307, 2308 and 1749.
Sample | 2307 | 2308 | 1749 |
Mass of analysed material (mg) | 90 | 90 | 44 |
Organic extract – OE (% raw material) | 98 | 99 | 94 |
Apolar fraction (% OE) | 2 | 2 | 3 |
Polar fraction 1 (% OE) | 43 | 47 | 20 |
Polar fraction 2 (% OE) | 55 | 48 | 77 |
GC–MS analyses were carried out using a Varian CP-3800 gas chromatograph interfaced with a Varian 1200L mass detector. The unit includes a CP8410 autosampler. The chromatograph and triple quadrupole spectrometer 1200L are controlled by a Varian MS Workstation version 6.4.1. and a Windows 2000 pro-operating system (on-column injector, injector initial temperature: 35 °C, HP-5 MS column, 30 m × 0.32 mm i.d., 0.25 μm film thickness, temperature program: 40 °C (1 min), 40–100 °C (10 °C/min), 100–300 °C (4 °C/min), isothermal 300 °C). Helium was used as carrier gas (initial pressure: 1.5 psi). The GC–MS data were collected every 700 ms. Mass spectra were produced at 70 eV, source 180 °C, in full detection mode over 50–700 a.m.u. The GC–MS Workstation Saturn® (Varian) software was used to undertake the semi-quantitative analysis of the 14 selected components present in the ketone fraction. Integration of the GC peak area of each component was normalised to the area of the whole triterpenic components.
HPLC–MS analyses were performed on an Agilent 1100 series (Agilent technologies, Waldbronn, Germany) solvent delivery system, coupled to an Esquire 3000plus (Bruker Daltonics, Bremen, Germany) fitted with an APCI source. This was operated with a vaporizer temperature of 420 °C, capillary temperature of 325 °C and corona current of 5 μA. High purity nitrogen was used for the nebulizer and dry gas, at 3 bars and 6 ml/min respectively. Spectra were obtained over the range m/z 200–1500. A C18-A Polaris column (250 × 2.0 mm i.d., 5 μm, Varian Inc., USA) was used with n-propanol (A) and acetonitrile (B) as mobile phase at a flow rate of 0.2 ml/min (program mobile phase: 100% B (0 min), 70% B (30 min), 55% B (50 min), 30% B (70 min), 100% A (80 min)). All samples were dissolved in CH2Cl2.
The compound specific stable carbon isotope analyses (δ13C values) of the fatty acids were obtained by the use of an Agilent 6890 GC coupled to a Thermoquest/Finnigan MAT Delta S isotope ratio mass spectrometer by a combustion (C) interface III (GC-C-IRMS) under a continuous helium flow [13,14]. The combustion interface consists of two ceramic furnaces, an oxidation reactor with CuO/NiO/Pt wires at 940 °C and a reduction reactor with Cu wires at 600 °C. Water was removed from the effluent gas by passing a Nafion tube (Perma Pure, Toms River, NJ, USA) with an annular back-flow of He. The IRMS ion source pressure is lower than 6 × 10−6 bar. The GC was operated with the same type of column and temperature program used for GC–MS analyses. The background subtraction and δ13C values were calculated using the Thermoquest/Finnigan ISODAT 7.2 software. The repeatability and intermediate precision of the GC-C-IRMS procedure, and the performance of the GC and combustion interface were evaluated every 5 analyses by injection of an in-house mixture of n-alkanoic acids (UNIL-FAME MIX) of known isotopic composition (δ13C10:0 = −31.1‰, δ13C12:0 = −29.7‰, δ13C14:0 = −29.2‰, δ13C16:0 = −29.6‰, δ13C18:0 = −29.2‰, δ13C12:0 = −27.6‰, δ13C20:0 = −29.4‰, δ13C21:0 = −28.6‰, δ13C22:0 = −28.4‰), and at least three replicate analyses of the samples. The standard deviations for repeatability ranged between 0.05 and 0.4‰ for the main FAME and for intermediate precision between 0.3 and 1.1‰. The accuracy of the GC-C-IRMS analyses was checked every 10 analyses by injection of a FAME isotope standard (icosanoic acid methyl ester, δ13C20:0 = −25.4‰) prepared by A. Schimmelman from the Biogeochemical Laboratories at Indiana University, USA. The isotopic shift due to the carbon introduced in the fatty acid methylation was corrected by a mass balance equation:
δ13CFAME = fFA δ13CFA + fMeOH δ13CMeOH |
3 Results and discussion
3.1 Homogeneous organic material: Dipterocarpaceae resins from Thailand
Investigations were carried out on two resin-like samples (numbers 2307 and 2308), visually different in colour, discovered during the 2005 campaign of terrestrial excavations at Khao Sam Kaeo, on the north coast of the Thailand Peninsula. The Upper Thai Peninsula site of Khao Sam Kaeo is an especially relevant site for the understanding of the mechanisms of early trans-Asiatic trade and cultural exchanges [15]. The exchanges closely linked Indian Ocean societies to South China ones and took part in the formation of Indian and Southeast Asian societies which share several cultural values. As Dammar resins have been traded for centuries to be used, amongst others, as waterproofing agents and therefore have played an important economical role in Southeast Asian countries, their occurrence at Khao Sam Kaeo was very likely. Palynological characterisation failed to identify the resin because of the contamination of samples by surrounding pollen. The so-called Dammar resins are produced by trees from the Dipterocarpaceae family which consists of 17 genera and nearly 500 species mainly tropical. The molecular characterisation pattern of dammars has been described extensively in the literature: besides a usually small sesquiterpenic fraction (C15), these resins contain an important triterpenic fraction (C30) consisting largely of compounds from the tetracyclic dammarane and the pentacyclic oleanane and ursane series [16–18]. The members of the Dipterocarpaceae are difficult to distinguish one from another on the basis of their triterpenoid compositions. In the case of archaeological samples, ageing, alteration processes and the possible mixture with other raw organic materials (beeswax, bitumen, animal fat, etc.) make the Dammar identification even more difficult.
Sample 2307 consists of brown lumps with beige surfaces. Sample 2308, easy to grind, is an orange to brown organic matter. The age estimation of these two organic materials based on dating of geological layers where they were discovered is about 4th B.C.–2nd B.C. In order to elucidate their chemical composition and to characterise their origin and alteration state, these samples were investigated for biomarkers by gas chromatography–mass spectrometry (GC–MS). For comparison, samples of various species of recent Dammars, as well as other archaeological organic samples presumed to be Dammar-bearing ones, were also analysed [19].
3.1.1 Determination of sample composition
GC–MS analysis of fractions of the two Khao Sam Kaeo organic samples led to the separation of sesquiterpenoids and triterpenoids, suggesting the occurrence of triterpenic plant resin as a component of these samples (Fig. 2).
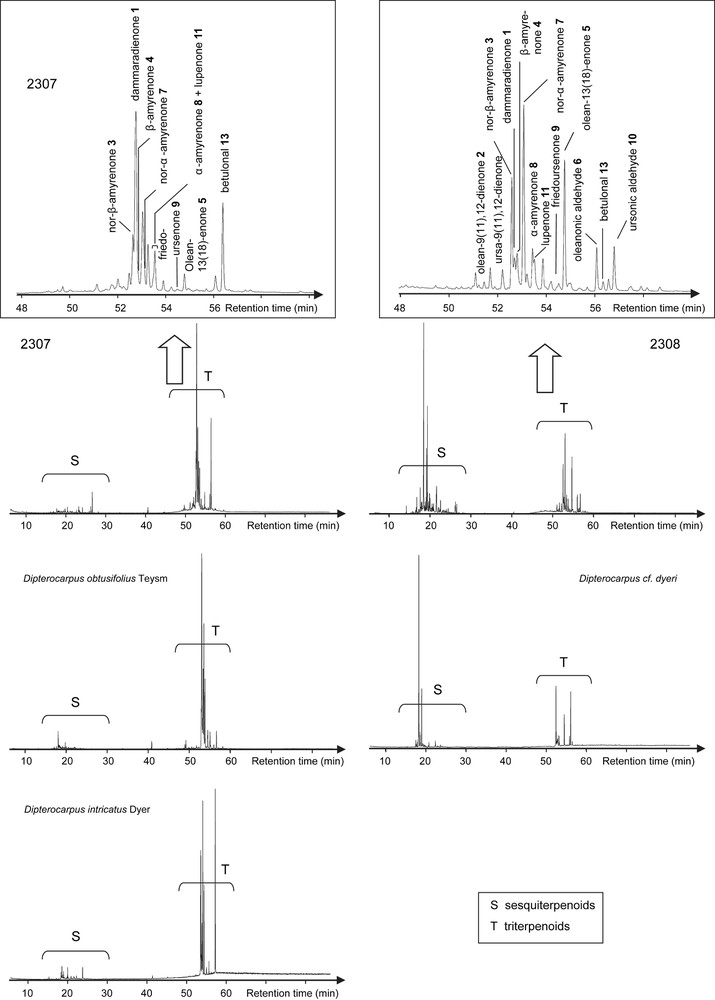
Reconstructed ion chromatogram (RIC) by GC–MS of ketone fractions from archaeological samples 2307 and 2308, and of fresh Dammars (Dipterocarpus obtusifolius Teysm, Dipterocarpus cf. dyeri, Dipterocarpus intricatus Dyer).
The two corresponding ketone fractions display one tetracyclic dammarane derivative (1) together with pentacyclic oleanane (2, 3, 4, 5 and 6) and ursane (7, 8, 9 and 10) derivatives [18], which correspond to the Dammar specific biomarkers pattern [16]: the two organic residues are made up of Dipterocarpaceae resin (structures are shown in Appendix). Among these compounds, there are also a few other triterpenoids which have been previously identified in Dammar but which do not display any Dammar biomarker value (11 and 12, Fig. 2).
Triterpenoid compositions of fresh Dammars from two major genera – Dipterocarpus and Shorea – were previously studied in the laboratory. Their taxonomy was clearly established by palynologists Dr. Bui Thi Mai and Dr. Michel Girard (CEPAM, Valbonne, France). Ketone fractions from fresh Dipterocarpus resins are dominated by an unknown triterpenic component (compound 13, Fig. 3) detected by GC–MS. Compound 13 was not found in the fresh Shorea resin. It was tentatively identified as betulonal by comparison with published mass spectrometric data and related mass spectra. Comparison of the experimental data of this Dammar component with those of synthetic betulonal obtained in the laboratory by betuline oxidation confirms this identification. This compound seems to be a biomarker characteristic for genera, because it is found in all Dipterocarpus species analysed in this study, but not in the Shorea one. Moreover, it appears to be unexpectedly resistant to alteration processes because it has been detected in almost all archaeological samples of this study. We can therefore hypothesize that the Dammars found in both Khao Sam Kaeo samples are from Dipterocarpus genus.
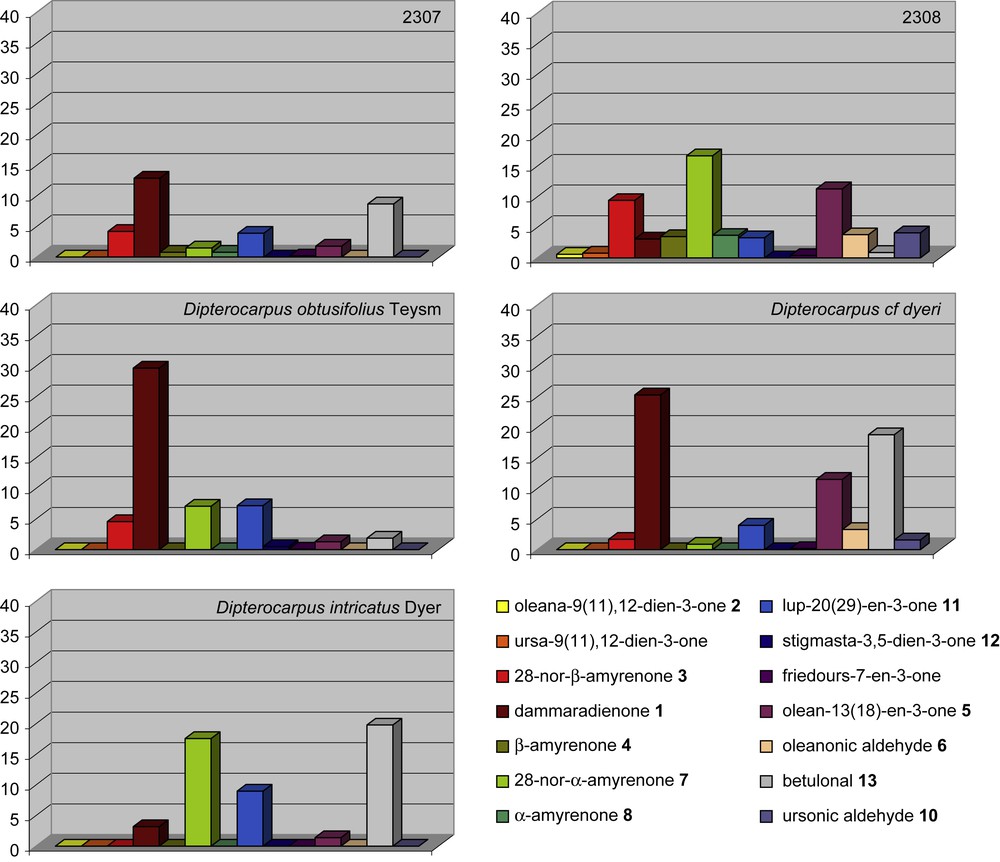
Relative abundance (by GC–MS) of selected ketones in % of total amount of triterpenic ketones from archaeological samples 2307 and 2308 and fresh resins (Dipterocarpus obtusifolius Teysm, Dipterocarpus cf. dyeri, Dipterocarpus intricatus Dyer).
Several pieces of evidence show that the two resins might rather belong to different species. As shown in Fig. 2, the sesquiterpenoids content is higher in sample 2308 than in sample 2307, where this biomarker class is only weakly detected by GC–MS. Among the fresh Dipterocarpus species studied in the laboratory, only Dipterocarpus cf. dyeri displays the same distribution (D. cf. dyeri, incorrect taxonomic appointment, probably refers to the species D. dyeri Pierre). This assumption is confirmed by the triterpenic ketones composition and their relative abundance (Fig. 3): oleanonic aldehyde 6 and ursonic aldehyde 10 occur only in sample 2308 and in D. cf. dyeri. Triterpenic ketones distribution of resin 2307 is more similar to the one of Dipterocarpus obtusifolius Teysm than to the one of Dipterocarpus intricatus Dyer which contains no nor-β-amyrenone 3 and only a small amount of dammaradienone 1.
3.1.2 Tapping procedure
In the apolar fraction of resin 2307, anthracene 14, pyrene 15 and fluoranthene 16 were identified. They are typical pyrolytic products stemming from incomplete combustion of organic matter. Partial burning may occur during the resin collection. The resin can exude naturally from the bark of living unhealthy trees or after animal damage or even due to wind action [20], but generally the commercial resin is obtained by tapping, a traditional method consisting in cutting a gentle incision in the bark before collecting periodically the exudates. When the flow stops, the cut is either refreshed by scrapping or another wound is made into the wood to induce further flow [20]. Most frequently, however, the wounded surface is burnt to stimulate resin exudation. This burning explains both the coal fragments detected in this sample during the palynological analysis and the darker colour of this resin compared to that of the 2308 one. As pyrolytic compounds were not identified in sample 2308, this resin was probably not submitted to the same thermal treatment during the tapping process.
3.1.3 Alteration
In both 2307 and 2308 samples, proportions of dammaradienone 1 compared to the total amount of triterpenic ketones are significant (respectively 1.5 and 2.3%, Fig. 3). This compound is described to have a good thermal stability [21–23] and a high sensitivity to light [5,17]. Consequently, these resins must have been protected from light due to their burial.
In all other archaeological organic resins mostly excavated from marine sites and analysed in the laboratory, two series of triterpenic degradation compounds have been characterised as des-A-triterpenoids 17 and A-neo-triterpenoids 18, compounds which are not detected in fresh resins. Series of similar des-A-triterpenoids have been observed by Corbet [24] and Trendel [25] in petroleum and various sediments (Mahakam delta sediment [24], Baltic sea sediment [26], muds of temporary ponds near Strasbourg [25]). The most likely possibility is that these substances are derived from the microbially mediated oxidative loss of the A-ring from 3-oxygenated pentacyclic triterpenic precursors, such as α- and β-amyrins (or more likely their ketone counterparts), characteristic constituents of higher plants, notably of tree barks [24,27–29]. Microbiological processes are also responsible for the progressive aromatisation, via dehydrogenation, of ring B to ring E [30]. These degradation substances derive mainly from the oleanane, lupane, ursane and sometimes friedelane series [24]. A-neo-triterpenoids (e.g., 18, Appendix) are ring A-contracted alkenes formed by rearrangement of their precursors (e.g. β-amyrine) under slightly acidic conditions [31,32], and were, for instance, identified in a lignite sample by ten Haven [33]. No compound belonging to either of one of these series was detected in samples 2307 and 2308, indicating that these types of degradation processes have not taken place, a feature which may be explained by the different excavating environmental conditions: terrestrial sites for samples 2307 and 2308, marine sites for the others.
3.2 Composite organic material: crusts on Osiris statuettes from Pharaonic Egypt
Mummification was practised in Egypt for at least 2000 years but only two descriptions of the mummification process are available from the two Greek authors Herodotus (5th century B.C.) and Diodorus of Sicily (1st century A.D.). Although the development of different analytical techniques like mass spectrometry allowed the analysis of embalming material by using only a small amount of sample, the identification of the ingredients is still very difficult. This is mainly due to both the high number of components within the mixture and to alteration processes (oxidation, microbial transformation, chemical reactions among constituents), which may severely change the molecular fingerprint of the primary organic material. Meanwhile, analytical investigations have revealed key materials used for embalming human bodies [2,34–38]. Nevertheless, many components and their sources remain unidentified. Furthermore, little is known about the variety of mixtures which have been used for distinct purposes, e.g. the mummification of animals and statues related to funerary material.
We show here the analysis of an organic deposit covering the Osiris statuette E27140 (Louvre Museum, Department of Egyptian Antiquities, room 13, display case 6, Fig. 4), a piece of a series of four statuettes [39]. Sample 1749, collected near the waist at the back of statuette, is a black organic matter easy to grind (265 mg), containing some lumps with a white surface, together with a piece of tissue (linen?). In ancient Egypt the year was divided into three seasons: the flood of the river Nile, the sowing and the crop. The end of the first period was celebrated with the festivity of “choiak”. It was the feast of fertility and rebirth and was thus closely related to the adoration of the god Osiris. An important part of the associated ritual was the preparation of small statues (ca. 20–30 cm) of Osiris. A mixture of mud and grains (cereal seeds?) was put into a mould with the shape of Osiris. After drying, the statue was enwrapped with linen and treated with an embalming-type material. Finally, the statues were brought to tombs. The aim of the study was to elucidate the chemical composition of sample 1749 by identifying diagnostic biomarkers. A comparison of the analytical results of this series of samples with embalming material used for human mummies was scheduled to emphasize similarities and differences in both composition and complexity of the materials.

Photographs (©Louvre Museum, provided by Marc Etienne) of a statuette of Osiris (sample 1749).
3.2.1 Bitumen
The presence of bitumen/asphalt was proven by the identification of steranes 19 and hopanes 20 (Fig. 5) in the less polar fraction, namely saturated hydrocarbons (Fig. 1). Steranes and triterpanes are the fossil degradation products of widespread sterols and bacteriohopanetetrols [3].
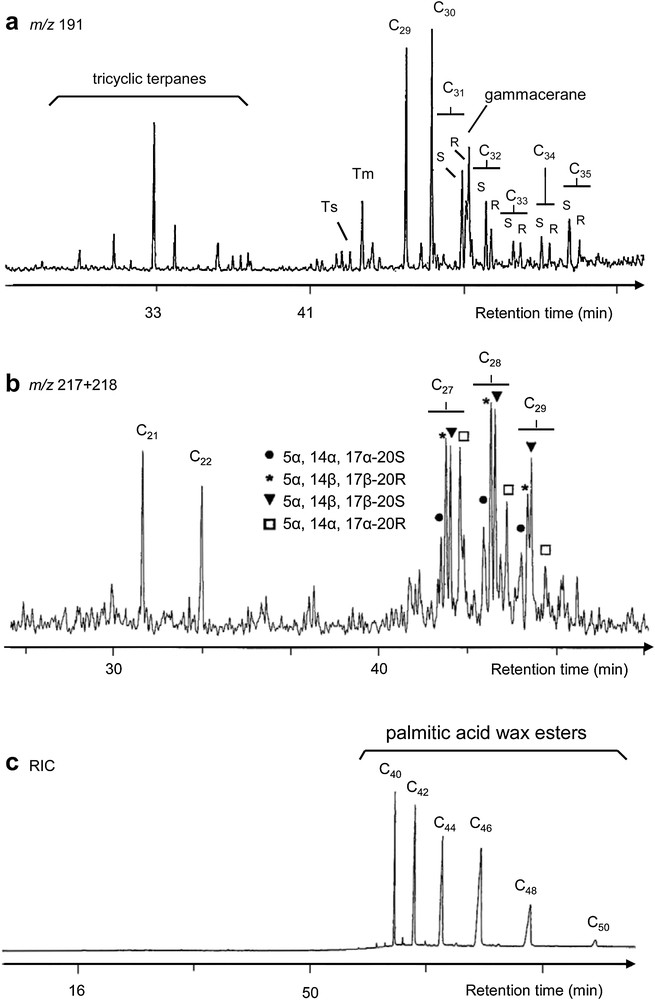
Hopane (a, m/z 191) and sterane (b, m/z 217 + 218) mass fragmentograms (GC–MS) of the aliphatic fraction, reconstructed ion chromatogram (c, RIC, GC–MS) of the ester fraction showing the distribution of palmitic acid wax esters of sample 1749. Cxx denotes the total carbon numbers of the molecule, Tm = 17α-trisnorhopane, Ts = 18α-trisnorneohopane.
Several parameters have been calculated after single ion monitoring experiments by GC–MS on steranes and hopanes. In comparison with adequate reference materials, the relative concentrations of the different stereoisomers of cholestanes, 24-methylcholestanes and 24-ethylcholestanes and of hopanes allow one to determine the source of bitumen, which in this case, closely resembles the composition of Dead Sea asphalt (Table 2, [40,41]).
Relative concentrations of the different stereoisomers of cholestanes, 24-methylcholestanes and 24-ethylcholestanes and of hopanes calculated after single ion monitoring experiments (according to Connan and Nissenbaum, 2004 [40]).
Steroids | Hopanoids | ||||||||
% C27 | % C28 | % C29 | S/(S + R) | ββ/(αα + ββ) | Ts/(Ts + Tm) | C29/C30 | C35/(C31–C35) | Gama/hop | |
Sample 1749 | 35 | 38 | 27 | 0.35 | 0.54 | 0.24 | 0.48 | 0.16 | 0.58 |
The presence of bitumen in the mixture reveals a plausible explanation for the dark colour of the material. Black colour is the colour of rebirth which will be the fate of the mummy in the afterworld [2,42]. There is ample evidence that asphalt was also often used as ingredient for embalming material of humans [2,43].
3.2.2 Beeswax
The gas chromatogram of the least polar fraction of the group of hydrocarbons containing heteroatoms (“Ester fractions”, Fig. 1) is simple and shows a distribution of about 10 major and minor components (Fig. 5). On the basis of mass spectrometrical data, the compounds have been identified as palmitic acid wax esters with even numbered carbon chains ranging from C40 to C50. Although wax esters are widespread, such a distribution is typical for beeswax [43–45]. The white colour of some lumps is probably due to “waxy regrowth”, specific feature of materials containing waxes. Beeswax was an important and quantitatively significant ingredient in balms which have been used to mummify human bodies [2,34,43].
3.2.3 Animal fats
Triacylglycerols 21, indicative of the presence of fats or oils, were detected in the ketone fraction (Fig. 1) by direct injection probe in mass spectrometry. Several data clearly exclude vegetable oils as the major potential source of lipids and suggest that the lipids originate from animal fat: the distribution of the free fatty acids and of the fatty acids released upon hydrolysis of triacylglycerols with a large predominance of chains with 16 and 18 carbon atoms (Fig. 6), the presence of a mixture of branched-chain components (C15:0, C17:0) among the acids [46].
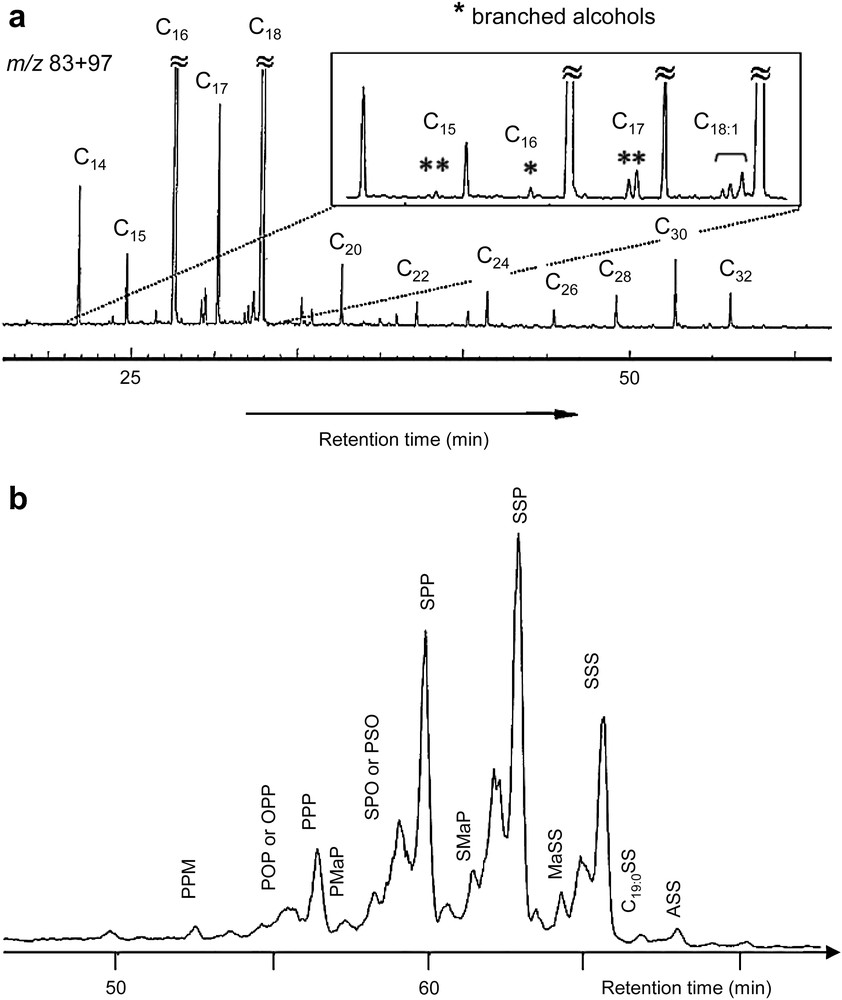
Mass fragmentogram m/z 83 + 97 showing the distribution of the fatty acids released upon hydrolysis of triacylglycerols (a) and reconstructed ion chromatogram (RIC) by LC–MS of the triacylglycerols fraction (b) from the Osiris organic deposit 1749. Abbreviations of acyls: myristic (M) C14:0, palmitic (P) C16:0, margaric (Ma) C17:0, oleic (O) C18:1, stearic (S) C18:0, arachidic (A) C20:0.
While the detection of animal fats is straightforward, identifying their biological origin is much more challenging [47]. The presence, in the fraction of free fatty acids, of a complex mixture of unsaturated C18 chains is characteristic of ruminants (bovine, ovine – biohydrogenation of unsaturated dietary fats), the distribution of monogastric or non-ruminant animal acids (such as human, porcine, fowl) being characterised by the presence of oleic acid (Z-9-octadecenoic acid) as the only unsaturated fatty acid (Fig. 6; [46,48–50]). Furthermore, carbon isotopic composition (ratio 13C/12C called δ13C) of individual compounds is strongly dependent on biological pathways and on transformation processes and can be used as a basis for distinguishing between the different possible origins of biological molecules [13,14]. The δ13C values obtained for the C16:0 and C18:0 free fatty acids by gas chromatography-combustion-isotope ratio mass spectrometry (GC-C-IRMS) are close to −29‰/PDB. This finding suggests again that the lipids derive from ruminant animals including sheep and cattle (δ13C −30 to −28‰/PDB) and not from monogastric animals, such as pigs (δ13C −25 to −24‰/PDB) [48,50]. HPLC–APCI MS analysis of fats allowed the triacylglycerol (TAG) molecular species to be identified, as well as the position of fatty acid substitution within those TAGs to be determined [51]. This distribution is dependent on biological origins [52]. The TAGs profile of this embalming-type material reflects a fatty acid composition showing a high proportion of saturated fats (Fig. 6). Stearic and palmitic acid containing components dominate the distribution including components between PPM and ASS. Odd-chain fatty acid containing TAGs can be seen, including MaSS. This profile is close to the TAGs distribution of other ruminant fats analysed in the laboratory and favours a tallow origin.
The alcohol fraction is dominated by two series of compounds which present a base peak at m/z 85 and m/z 99. The mass spectrometrical data were consistent with γ-and δ-lactones (22 and 23) bearing a side chain ranging from 8 to 18 carbon atom. These structures were confirmed by comparison of their analytical data with those of synthetic lactones (side chain with 10, 14, 15 and 18 carbon atoms) based on the following criteria: identical mass spectra and co-elution on two capillary columns with different stationary phases (DB-5 and DB-17) in gas chromatography. They were detected in other embalming materials [2] including samples studied in the laboratory (e.g. balm of Padiimenipet mummy, 116 A.D., Louvre Museum). It is established that long chain aliphatic γ-and δ-lactones are present in animal fats [53] and that they originate from hydroxy fatty acids [54,55].
3.2.4 Higher land plants resin
Several polycyclic triterpenoids belonging to the oleanane and lupane series, characteristic of higher land plants were also detected in very small relative concentrations. These compounds are not specific of a particular resin. Only two among the four biomarkers generally used to identify an ancient resin mastic produced by a Pistacia tree (Pistacia lentiscus or Pistacia atlantica) were, however, present in this sample: moronic 24 and oleanonic acids 25 [56,57]. Tetracyclic triterpenoids from the masticadienonate series 26, which are the most diagnostic molecules occurring in present non-altered Pistacia resin, were not detected [58–60]. This might be explained by their high sensitivity towards alteration [54]. In conclusion, the molecular composition shows the presence of a higher land plant resin which could be produced by a Pistacia tree. This assumption needs to be confirmed with the analysis of other samples of the same Osiris series. Resin mastic was actually quoted as present in several human embalming materials [61].
4 Conclusion
In the case of ancient organic material on which human science researchers can only make assumptions, molecular and isotopic analysis often gives the possibility of making conclusions. Precise structural elucidation of biomarkers is an acute tool for the characterisation of pure materials or mixtures, degraded or non-altered. Among other things, it allows one to identify natural substances (biological and geographical origin) to evaluate their degradation state and then to significantly enhance our understanding of knowledge and technologies of past societies.
The two samples from Khao Sam Kaeo are pure Dammar resins from Dipterocarpus genus but from different species (D. cf. dyeri and D. obtusifolius Teysm) collected by different tapping procedures. The by-products resulting from the alteration of pristine biomarkers occurring in resins – des-A-triterpenoids and A-neo-triterpenoids – identified in archaeological samples from marine site excavations, were not present in these two samples unearthed from terrestrial sites. New diagnostic parameters, essential for the precise identification of dammar, were suggested. The presence of these resins in the Khao Sam Kaeo area was clearly demonstrated and suggests that the Upper Thai Peninsula was clearly an exchange area.
The composition of the embalming-type material of the Osiris statuette analysed is comparable to that of the true balm used for the mummification of humans: beeswax, animal fat (tallow), Dead Sea asphalt, higher land plant resin (probably Pistacia). This feature shows that the symbolic of the mixture used in the “Osiris” organic deposit is probably the same as that used in human balms. The identification of Dead Sea asphalt points again out that this bitumen was present and used in Pharaonic Egypt.
Acknowledgments
The authors thank DFG, DFH/UFA and MENESR (International Research Training Group 532 – GRK532) for financial support (post-doctoral fellowship of Joachim Maurer, PhD fellowship of Pauline Burger). They gratefully acknowledge Bérénice Bellina (CNRS – UMR 7528 “Mondes iranien et indien”, Ivry-sur-Seine, France) who has accepted to supply some resin samples from the 2005 campaign of excavations at Khao Sam Kaeo, and curator Marc Etienne (Department of Egyptian Antiquities, Louvre Museum, Paris, France) for Osiris statuettes. We are extremely grateful to Dr. Bui Thi Mai and Dr. Michel Girard (Laboratoire de Palynologie, CEPAM, Valbonne, France) for pollen analysis and to Estelle Motsch and Raymonde Baltenweck, Université Louis Pasteur, Strasbourg, for mass spectral analysis.