1 Introduction
Pectic molecules are complex polysaccharides present in many food preparations, from raw vegetables to cooked vegetables and beverages. In foods, these polysaccharides are considered as fibres, or as gelling agents. In addition to the texturizing properties described for pectins, more recently, these molecules have been considered for their biological activities. In fact, pectin molecules are heteropolymers consisting of three distinct sequences known as homogalacturonan (HGA), rhamnogalacturonan-I (RG-I), and rhamnogalacturonan-II (RG-II) [1]. Homogalacturonan sequences are constituted of (1 → 4)–linked α–d–galacturonic acid residues with partially methylated and/or acetylated substitutions [2] while RGI and RGII consist, respectively, of rhamnogalacturonan or galacturonan sequences with various backbones on RGI and conserved sequences on RGII backbones. Concerning pectin biological activities, it was shown that dietary fibres consisting in whole pectin lower total [3] and LDL cholesterol concentration in humans [4], while RGI sequences stimulate macrophage activation in vitro [5]. Another biological property can be underlined in medicine where pectins revealed to be active on cancer growth and metastasis [6].
Other polysaccharides containing only uronic acid sugars such as the HGA pectic sequence can be found as homoglucuronans present in Mucorales [7] or obtained during fermentation of the Sinorhizobium meliloti M5N1CS strain (NCIMB 40472). There, the bacterial polymer consists of d-glucuronic acid residues β-(1 → 4) linked and partially acetylated in C-2 and/or C-3.
In order to determine potential interactions between uronic acid sequences and lipid molecules and try to understand the activity of pectin on cholesterol reduction in human blood, studies were carried out with homogalacturonan and homoglucuronan molecules mixed with cholesterol or linolenic acid, respectively. Analyses of constituted complexes were conducted using nuclear magnetic resonance (NMR) spectroscopy as described in the literature [8,9].
2 Material and methods
2.1 Materials
Glucuronic acid, galacturonic acid, trigalacturonic acid, linolenic acid, cholesterol, deuterium oxide and deuterated chloroform were from Sigma-Aldrich (Saint Quentin Fallavier, France).
Native glucuronan was produced by the Sinorhizobium meliloti M5N1 CS mutant strain (NCIMB 40472) in bioreactors under conditions described by Tavernier M.L. (2008) [10]. A complete deacetylation of the polymer was performed according to methods previously described [11,12]. An oligoglucuronan with a degree of polymerisation of 3 (DP 3) and low molecular weight (LMW) glucuronans, average DP = 20, were obtained by degradation of the deacetylated glucuronan with a fungal glucuronan lyase [10,12].
The high molecular weight galacturonan (HMW galacturonan) was obtained from citrus pectin extracted with HCl (4 M, 50 °C, 3 h). After neutralization of the pectin extract with 4 M NaOH, HMW galacturonan molecules were recovered by precipitation with two volumes of ethanol (during overnight at 4 °C). The precipitate containing HMW galacturonans was collected by centrifugation (15 000 × g, 30 min, 4 °C); then, it was dissolved in water and dried by lyophilisation. The HCl treatment induced a deacylation of the molecule.
Low molecular weight galacturonan (LMW galacturonan) was obtained by degradation of the previous HMW galacturonan preparation. The degradation was obtained by heating a 1% HMW galacturonan solution (in water, adjusted to pH 4.4 with NaOH) at 121 °C for 40 min [13,14]. The LMW molecules were obtained as described above except for the quantity of ethanol added; here, seven volumes of ethanol were necessary to precipitate the LMW galacturonan fraction.
2.2 NMR spectroscopy
1H and 13C NMR experiments were carried out at 25 °C on a Bruker Avance 300 spectrometer (Bruker, Wissembourg, France) equipped with a narrow-bore magnet operating at a resonance frequency of 75 MHz for 13C and at 300.16 MHz for 1H. The HOD signal was presaturated using a presaturation sequence provided by Bruker. All carbohydrate samples: glucuronic or galacturonic acids, triglucuronic or trigalacturonic acids, LMW glucuronan or galacturonan and HMW glucuronan or galacturonan (5 mg) were first dissolved in D2O (99.9%; 0.5 ml) and freeze-dried to replace exchangeable protons with deuterium, prior to be finally dissolved in the same D2O volume. Chemical shifts were expressed in parts per million relative to external sodium–3–(trimethylsilyl)–propionate–d4 and residual HOD signal (internal standard) for aqueous phases. Chemical shifts were referenced to tetramethylsilane (internal standard) and the residual CHCl3 signal for organic phases. The complete assignment of protons and carbons from uronic sequences, cholesterol and linolenic acid was performed by combination of 2D NMR analyses (COSY, TOCSY and HSQC) and by comparison with values referenced in the literature [15–18]. Two-dimensional ROESY spectra with water presaturation were acquired using a phase sensitive pulse sequence with TPPI, with mixing time of 300 ms and recycle delay of 3 s.
2.3 Mixture preparation
The carbohydrate solutions in D2O prepared as described above were supplemented with pure cholesterol or linoleic acid (molar ratio 1:1). The preparations were shaken manually during five minutes, then, 0.5 ml of CDCl3 was added to the mixtures. The preparations were mixed as previously, and the CDCl3 fraction was separated after centrifugation of the preparation (15,000 × g, 30 min, 4 °C). All fractions were collected and then analysed by NMR spectroscopy as above.
3 Results and discussion
3.1 Interactions between uronic sequences and cholesterol
Pectins usually used as gelling agent in the food industry have been shown to lower the cholesterol concentration in serum of humans consuming pectins of various origins [3]. However, until now, no study had concerned the role of the different pectin sequences in this pectin biological property. To study possible interactions between uronic sequences in pectin and cholesterol, mixtures made of cholesterol and HGA sequences from pectins were prepared. Structures of molecules are presented in Fig. 1. To determine if interactions between cholesterol and HGA may be selective or not, several sugar molecules containing uronic acids were tested, such as the galacturonic acid, trigalacturonic acid, Low Molecular Weight galacturonan (LMW galacturonan) and High Molecular Weight galacturonan (HMW galacturonan), but also glucuronic acid, triglucuronic acid, Low Molecular Weight glucuronan (LMW glucuronan) and (High Molecular Weight) HMW glucuronan. Complexes formation between carbohydrate and lipid molecule were studied using NMR spectroscopy.

Structure of galacturonic acid (a), glucuronic acid (b) galacturonic sequences (c) glucuronic sequences (d), cholesterol (e) and linolenic acid (f). All carbons are numbered.
First, we observed 13C NMR chemical shift variations (Δδ) from carbons of uronic acid in pure carbohydrate solutions between the same carbons when carbohydrate molecules were mixed with cholesterol in order to determine the saturation of carbohydrate by lipids. NMR data indicate that as the ratio of [lipids]: [carbohydrate] increased from 0.5 to 3, the change in chemical shift gradually increased and saturated at 1. For example, 13C NMR chemical shifts variations (Δδ) increased from 0.01 to 0.4 ppm from C–6 of HMW galacturonan in interaction with increasing ratio of cholesterol from 0.5 to 1. The upper ratio give the same Δδ⋅⋅ Then, all experiments were realised in saturated conditions.
Second, we compare 13C NMR chemical shifts variations (Δδ) to determine the influence of nature and molecular weight of uronic sequences (Fig. 2). The highest variation (Δδ = 0.40 ppm) was found for C–6 from the HMW galacturonan (Fig. 2a), but variations were also observed on C–6 from LMW galacturonan and trigalacturonan. These chemical shift variations came with a decrease of 5% of the intensity on C-6 signal from the HMW galacturonan and about 4% from LMW galacturonan and trigalacturonan. In addition, minor chemicals shifts variations (0.06–0.10 ppm) were detected for C-1, C–3 and C–5 in galacturonic sequences, but not in the monomer (Fig. 2a). We observe also signal intensity variations (Δi) of about 5% for C–1, 11% for C–3 and 15% for C–5 from the HMW galacturonan and LMW galacturonan. 1H NMR spectra of galacturonans sequences were carried out, but only those from HMW galacturonan can be exploited from chemical shift variation studies, due to the overlapping of signals in LMW galacturonan, trigalacturonan and monomer. The Δδ of 0.01, 0.02 ppm for H–1 and H–3/H–5 were observed respectively. The chemical shift and intensity variations revealed that galacturonans with higher molecular weight entrapped greatest concentration of cholesterol, because of the highest variations from the large molecules. Moreover, the entrapment involved mainly the C-6 of galacturonic residues, while such residues alone were not able to interact with cholesterol. Probably, a particular conformation of galacturonic sequences in HMW molecules allowed a high level of interaction with the cholesterol; such conformation was probably reduced in LMW and trigalacturonic molecules. In addition, the 13C NMR spectrum of the HMW galacturonan initially mixed with cholesterol showed small signals between 10 to 60 ppm (Fig,. 3c). These resonances were attributed to cholesterol carbons by comparison to signals on the 13C NMR spectrum obtained with a pure cholesterol solution in CDCl3 (Fig. 3b) and pure HMW galacturonan in D2O (Fig. 3a). In 13C NMR spectra of the other galacturonan sequences, such signals were not detected.
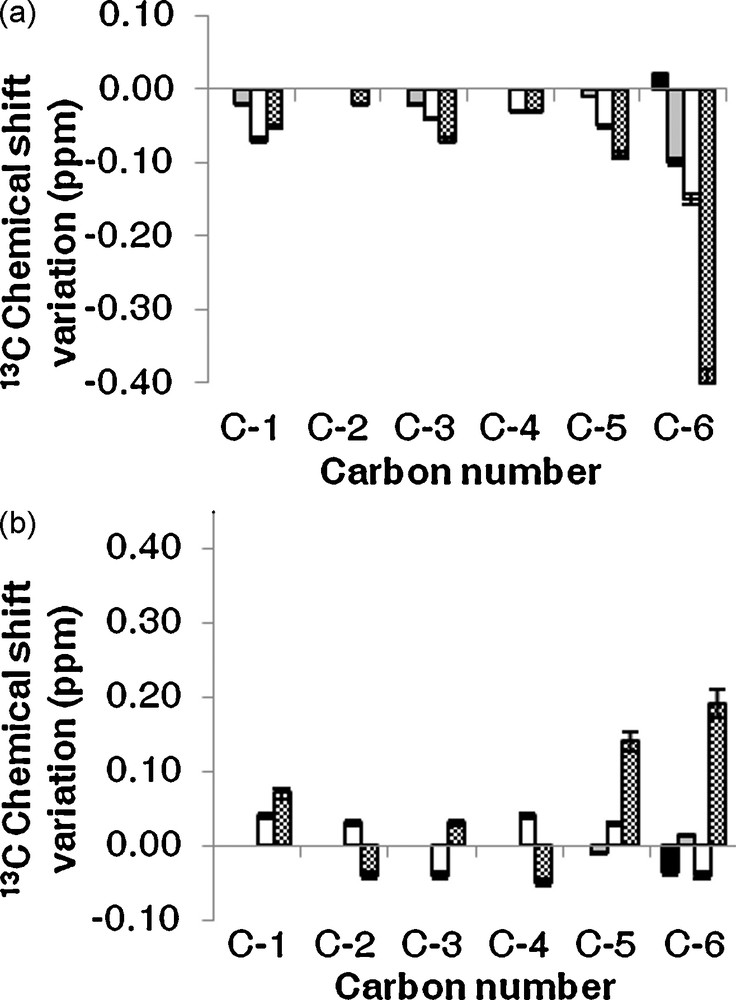
Experimental 13C chemical shifts variation pattern between uronic sequences in carbohydrate/cholesterol mixture and free uronic sequences. (a) Galacturonic sequences (b) Glucuronic sequences. (■: monomer of uronic acid, : Tri uronic acid, : LMW polysaccharide, : HMW polysaccharide).
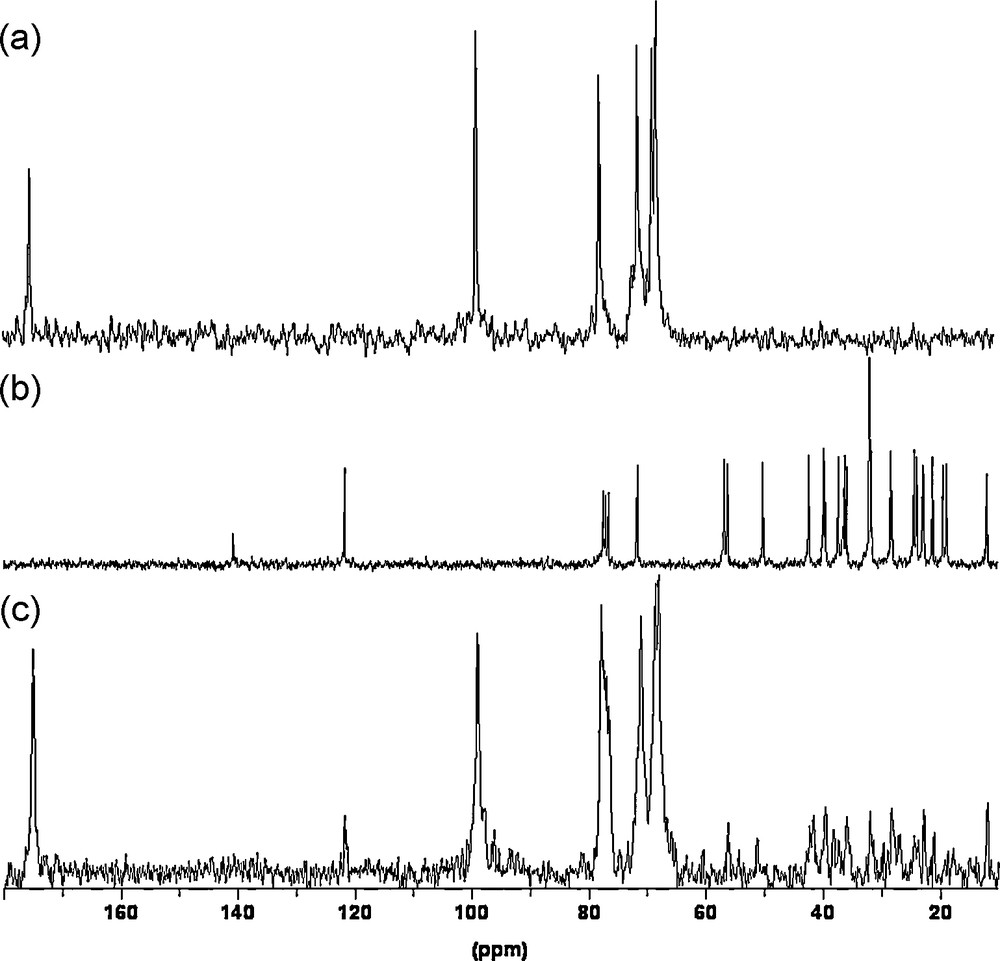
13C NMR spectra of HMW galacturonan in D2O (a), cholesterol in CDCl3 (b) and HMW galacturonan/cholesterol mixture in D2O (c) at 25 °C.
As for carbons in GalA residues, 13C NMR chemical shifts variations were observed for carbons from cholesterol mixed with galacturonans. The Δδ values were about 0.03 for C–3, C–5, C–6, C–13 and C–20 with the most important intensity variations on the C–6 signal (decrease of 14%), C–5 signal (decrease of 10%) and the C–3 signal (decrease of 5%) from the HMW galacturonan/cholesterol complex. For the other galacturonic sequences, the Δi of cholesterol signals were about 3%. The 1H NMR spectra showed chemical shifts variations of 0.01, 0.03 and 0.04 ppm on H–3/H–7, H–6 and H–4 signals, respectively, of cholesterol from HMW galacturonan/cholesterol complex. Minor chemical shifts variations (about 0.01 ppm) were detected for the same proton of cholesterol in interaction with other galacturonic sequences. For 1H NMR peaks, the intensities of signals decreased from 10 to 20% of H–3, H–4, H–6 and H–7 cholesterol signals from HMW galacturonan/cholesterol complex. These results let us suppose that interactions take place between atom of cycle A of cholesterol and C–3/H–3 and C–5/H–5 of GalA residues.
In order to understand the site of connectivity between cholesterol and galacturonan, two-dimensional ROESY NMR spectra were used to monitor the proximity between protons through space [9]. 2D ROESY spectrum of the HMW galacturonan/cholesterol complex (Fig. 4) showed the correlation of H–3 (3.47 ppm) of cholesterol with H–5 (4.35 ppm) on GalA residues and weak cross-peaks were observed between H–6 (5.39 ppm) of cholesterol and H–3 (3.75 ppm) on GalA residues. These results indicate that the interaction occurred with cycle A of cholesterol and mainly C–3 and C–5 of GalA residues.
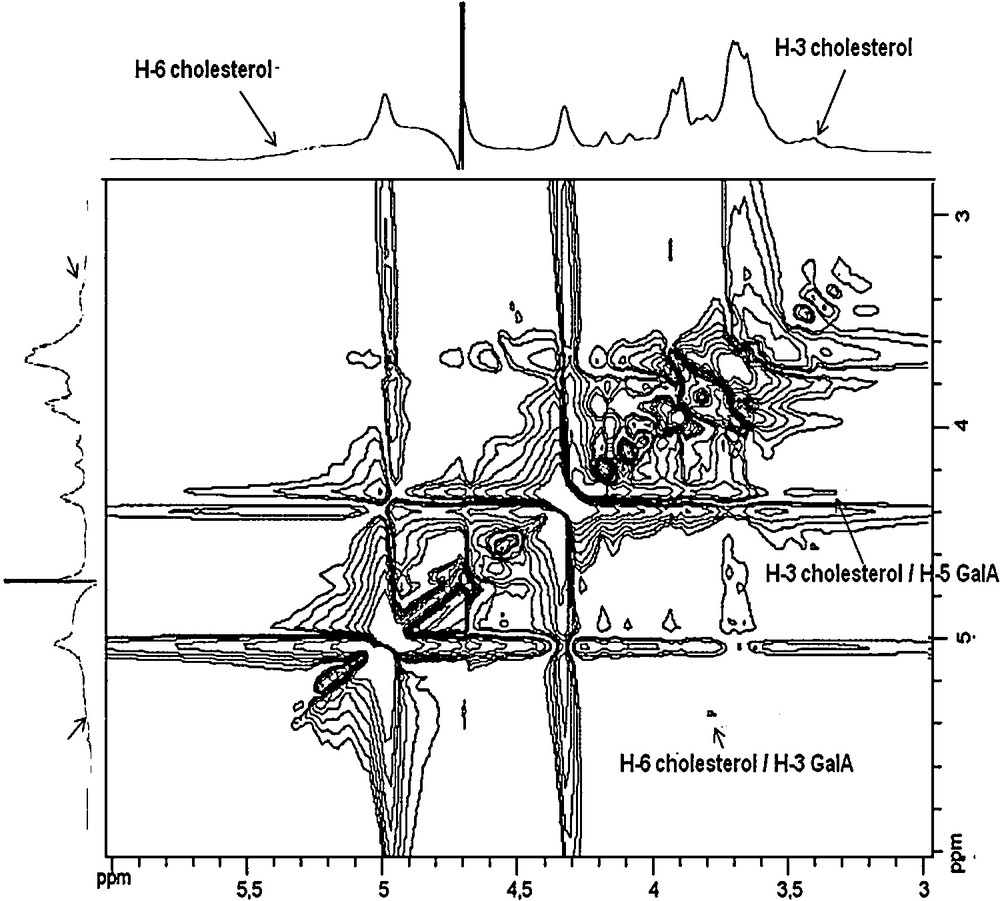
2D ROESY spectrum of HMW galacturonan/cholesterol mixture in D2O at 25 °C.
To determine if interactions were specific between cholesterol and GalA residues or not, the same experiments were carried out with High Molecular Weight (HMW glucuronan) and Low Molecular Weight glucuronan (LMW glucuronan), triglucuronan and glucuronic acid molecules. As for galacturonan molecules, 13C NMR analysis revealed chemical shift variations when glucuronan molecules were mixed with cholesterol (Fig. 2b). The highest variation was observed for C–6 (0.20 ppm) and C–5 (0.15 ppm) of glucuronic acid residues with a decrease of intensities of signals of about 7% in HMW molecules; minor chemical shift variations, between 0.02 and 0.07 ppm were detected for C–1 to C–4 (Δi about 4%) in glucuronic sequences mainly in HMW and LMW, and no Δδ was observed when cholesterol was mixed with the monomer (Fig. 2b). The 1H NMR spectra of HMW glucuronan/cholesterol complex showed chemical shift variations about 0.01 ppm for all glucuronan signals. In spectra obtained with glucuronan molecules initially associated to cholesterol, we clearly did not identify signals corresponding to carbons from cholesterol. This is probably due to the reduced concentration of lipid entrapped in the glucuronans. However, due to the Δδ and Δi observed, it was possible to suppose that the lipid was entrapped in the glucuronan molecules. As for carbons in GlcA residues, 13C NMR chemical shifts variations were observed for carbons from cholesterol mixed with glucuronans. The Δδ values were about 0.02 for C–3, C–5 and C–6 of cholesterol with very small intensity variations. From these results, we can propose that cholesterol interacts with glucuronic sequences as with galacturonic ones. The smallest Δδ observed from glucuronan sequences let us suppose that these interactions were more effective for galacturonan than for glucuronan.
Probably the molecules conformation may explain the differences observed for the entrapment of cholesterol by galacturonans or glucuronans in the saturated conditions. The β–(1 → 4)–glucuronan was shown to adopt a “ribbon-like” two-fold conformation [19,20]; perhaps, this conformation allows interactions mainly between the carboxylic group in C–6 and C-5, and cholesterol. This “helical” structure of glucuronan presented only few carbons as C–6 and C–5 of glcA residues in external parts to interact with cholesterol. Concerning the α–(1 → 4)–galacturonan, an important literature was focused on the formation of “egg-boxes” where homogalacturonan chains are linked together by ions, and where molecules can be entrapped [21,22]. In our experiments, no ions were added so, few “egg box” structures were present. To consider the entrapment of cholesterol in HGA, the lipid has to be included inside “apolar pockets” made of galacturonan residues, either on single chains or involving several HGA chains. HGA molecules can include cholesterol in their structure as it was observed with cholesterol inside cyclodextrins [23,24].
In the present study, we showed that interactions occur between pectin homogalacturonan sequences and cholesterol, probably due to the formation of “apolar pockets” along the pectin molecule, suitable to be filled by cholesterol molecules. Such interactions may occur in humans during feeding. In addition, galacturonan sequences revealed to be probably more suitable than glucuronan sequences to entrap cholesterol molecules.
3.2 Interactions between uronic sequences and linolenic acid
In order to determine if other apolar molecules may be entrapped inside pectin galacturonan sequences, the same experiments as above were conducted with galacturonan and glucuronan molecules as well as with the glucuronic and galacturonic acid monomers with linolenic acid instead of cholesterol. Fig. 1 shows the structures of molecules. 13C NMR chemical shifts variations observed from carbons of uronic acid mixed with linolenic acid between the same carbons when carbohydrate molecules were in pure solution were presented in Fig. 5. 13C NMR Δδ were observed for carbons in all galacturonan molecules (Fig. 5a). Whatever the galacturonan size, Δδ variations involved C–2, C–4, C–5 and C–6 while the treatment with linolenic acid did not affect the chemical shift of C–1 in the monomer and DP3 galacturonan residues as that of C–3 in the monomer (Fig. 5a). These Δδ come with Δi of 20% for C–6 and C–4 and 10% for C–2 and C–5. The 1H NMR spectrum of HMW galacturonan/linolenic acid complex showed Δδ about 0.01 ppm for H–2, H–4 and H–5 of GalA residues. Thus, the higher Δδ variation was observed for C-6 with Δi of 20% in LMW galacturonans, while in the previous study conducted with cholesterol, the higher Δδ variation was for C-6 in HMW molecules. Such differences, probably due to the lipid conformations, led us to analyse 13C NMR chemical shifts of carbons from pure linolenic acid dissolved in CDCl3 (data not shown) and those from linolenic acid in galacturonan-linolenic acid mixtures. The 13C NMR Δδ were observed for C–1, C–14 and C–15 of linolenic acid: a Δδ of 0.3 ppm was observed for C-1 with Δi of 3% in all samples where linolenic acid was mixed with galacturonan molecules. Moreover, a Δδ = 0.06 ppm were found for C–14 and C–15 (Δi = 5% and 2% respectively) from lipid molecules mixed with DP3 galacturonan molecules, and this value increased to 0.30 ppm (Δi = 10% and 3% respectively) when the lipid was mixed with LMW and HMW galacturonans and confirmed that highest molecular weight galacturonans entrap more lipid molecules than smallest ones. The 1H NMR spectra were also collected from galacturonic sequences/linolenic acid complexes. The higher Δδ variation was observed for H–14 (0.03 ppm) and H–15 (0.04 ppm) of linolenic acid, with Δi of 6% and 10% respectively, in the LMW galacturonans/linolenic acid complex. From these results we determine that linonenic acid was in interaction with galacturonans sequences. Currently, 2D ROESY experiments carried out in the HMW galacturonan/linolenic acid complex can be not interpreted due to the overlapping of signals in the spectra. High field NMR spectrometer will be used to resolve this problem.
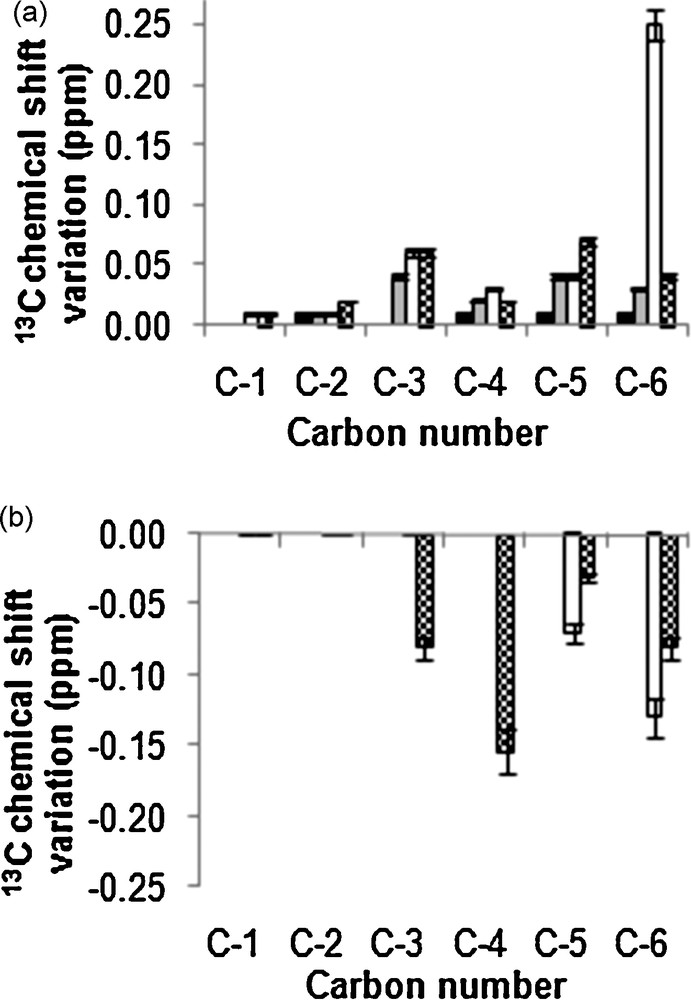
Experimental 13C chemicals variations pattern between uronic sequences in carbohydrate/linolenic acid mixture and free uronic sequences. (a) Galacturonic sequences (b) Glucuronic sequences. (■: monomer of uronic acid, : Tri uronic acid, : LMW polysaccharide, : HMW polysaccharide).
When glucuronan molecules were mixed with linolenic acid, increased 13C chemical shifts variations were observed for C–5, C–4, C–3 and C–6 (Δδ from 0.03 to 0.17 ppm with Δi from 4 to 18% respectively) only in HMW or LMW glucuronan molecules (Fig. 5b). In addition, the comparison of 13C NMR chemical shifts of carbons from pure linolenic acid dissolved in CDCl3 and those from linolenic acid in glucuronan/linolenic acid mixtures revealed that the lipid was associated to glucuronans by C–1 (Δδ = 0.3 ppm, Δi = 2%), C–14 (Δδ = 0.10 ppm, Δi = 2%) and C–15 (Δδ = 0.14 ppm, Δi = 2%), the same carbons were shown in the previous experiment to be associated to galacturonans. 1H NMR spectra were also collected from glucuronic sequences/linolenic acid complexes. The higher Δδ variation was observed for H–14 and H–15 (0.01 ppm), with Δi of 2%, in LMW and glucuronans/linolenic acid complex. The 13C and 1H Δδ obtained indicated that interactions between glucuronan molecules and linolenic acid were very small.
Thus, this study showed that homogalacturonan sequences can interact also with linolenic acid more efficiency than homoglucuronan ones. These entrapments can be compared to those between palmitic acid and cyclodextrins (CD) [25]. The chemical shift variations observed with linolenic acids were different for the two types of polysaccharides uronic acid sequences. The long chain of linolenic acid could probably interact with most carbon in an “apolar pocket” of galacturonan. On the other hand, the “helical” structure of glucuronan could offer only external carbon in interaction with linolenic acid. 2D ROESY experiments and determination of thermodynamics constants of the complexes will help us to identify the real interactions occurring in these complexes.
4 Conclusion
Our study revealed that homogalacturonan sequences present in pectin macromolecules can entrap lipid molecules such as cholesterol but also linolenic acid. Such interactions were shown to be also established between glucuronan molecules and the lipids tested, but differences observed for chemical shifts of carbons from the different uronic acid molecules were significant of various interactions due to specific conformations of uronic acid molecules from monomers to HMW polymers.
Further investigations, such as a determination of the thermodynamics constants of the complexes (Kd) by DOSY NMR experiment [26], 2D ROESY for uronic acid/linolenic acid complexes and molecular modeling will provide more convincing arguments for the identification of the governing interactions occurring between uronic sequences and lipids molecules.