1 Introduction
Regarding chemical productivity, it would be attractive to expand methodology by using handy substrates [1]. On the other hand, major problems in chemical production are the handling of waste and the search for environmental tolerable procedures [2]. For the solving of this problem, environmental pressures are forcing the chemical community to search for more efficient ways of performing chemical transformations [3]. However, it would be much more efficient if two or more transformations could be realized in one or few synthetic sequences without isolating the intermediates. So, designing of new ways for the synthesis of fine chemicals through this category is important. The pyridine nucleus has attracted tremendous attention for over a century [4–8]. This moiety has widely been utilized for biological, biochemical, pharmaceutical, and asymmetric chemical investigations [9,10]. Among them, multi-aryl substituted pyridines specially trisubstituted ones have found wide use as building blocks for supramolecular chemistry [11,12], as chemosensors [13] asymmetric catalysis [14], photosensitization [15] and antitumor chemo-therapeutics [16]. Due to the importance of these pyridine derivatives, development of efficient methods to generate these compounds has attracted considerable interest. The principal approach for the synthesis of these compounds limits to the direct condensation reaction of acetophenones with various aryl aldehydes and ammonium acetate in the presence of protic or Lewis acids, which have been well documented in the literature [17–29]. However, some of the aryl aldehydes are volatile, toxic, or unstable [30]. So, there is a need to broaden the scope of this reaction using safer substrates, via a one-pot reaction in combination with “green chemistry”.
Despite advances in heterocyclic synthesis, no attention has been paid to using aryl alcohol as substrate in the preparation of 2,4,6-triarylpyridine so far. It may due to the lower reactivity of alcohols in condensation reactions. However, alcohols are generally considered to be superior to aldehydes in terms of handling, preparation, toxicity, stability and functionalization [31]. Therefore, representing a new method in this case, which can be applied to the various substrates with different natures, from the viewpoint of total synthesis and for the preparation of new 2,4,6-triarylpyridine derivatives, is highly desirable. On the other hand, the development of a less-toxic reaction protocol and avoiding purification steps would extend the scope of this transformation. These merits prompted us to focus our work on the using of aryl alcohols as precursors in the synthesis of 2,4,6-triarylpyridines.
2 Results and discussion
In recent years, ionic liquids have attracted increasing interest in the context of green synthesis [32,33]. Although ionic liquids were initially introduced as alternative green reaction media because of their chemical and physical properties of non-volatility, non-flammability, thermal stability, and controlled miscibility [33], today investigation on binary ionic liquids has illustrated some unique properties of them [34–36]. These results in combination with our previous experiences on the ability of ionic liquids in organic synthesis, especially with task specific room temperature ionic liquids [37–39], led us for the first time to develop an elegant route for preparing 2,4,6-triarylpydines, through a tandem process using various primary benzyl alcohols, acetophenones or 2-arylethanols and ammonium acetate promoted by [HMIm]NO3-[BMIm]BF4 as a binary task specific ionic liquid under microwave irradiation (Scheme 1).
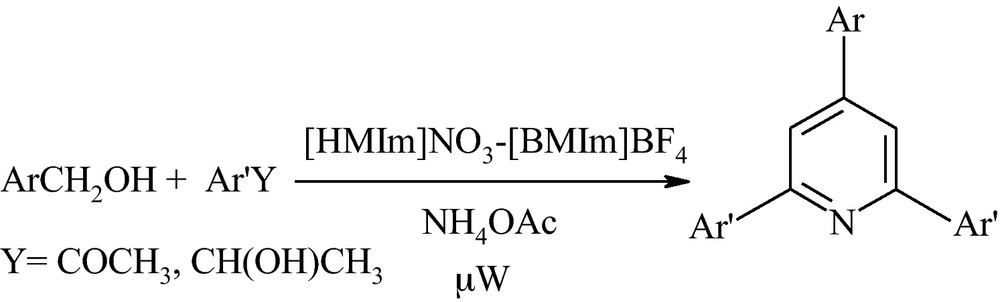
The experimental procedure for the reaction is remarkably simple and does not require the use of toxic or expensive organic solvents or reagents. At the beginning of our study and during the design of a suitable condition for the reaction course, we found that a two-step one-pot reaction sequence produces higher yields and omits the side products. Moreover, it is important to note that combination of [HMIm]NO3-[BMIm]BF4 is essential for this transformation, otherwise, the reaction does not proceed efficiently. More examination illustrated that [HMIm]NO3 acts as an oxidizing agent for conversion of aryl alcohols to the corresponding aldehydes and the reduced by-product (NO2) was detected during the reaction. Next, [BMIm]BF4 acts as a catalyst and promotes the reaction of the resulting aryl aldehyde with acetophenone and ammonium acetate. In order to evaluate the influence of the promoter and the temperature in this synthesis, the condensation reaction of benzyl alcohol with acetophenone and ammonium acetate in various reaction conditions was investigated (Table 1).
Tandem reaction for the synthesis of 2,4,6-triphenylpyrdine from benzyl alcohol and acetophenone under different conditionsa.
Entry | [Hmim]NO3 (10-2 mol%) | Co-ionic liquidb | Temprature (°C) | Yield (%)c |
1 | 1.1 | [BMIm]BF4 | 96 | 60 |
2 | 1.2 | [BMIm]BF4 | 96 | 79 |
3 | 1.3 | [BMIm]BF4 | 96 | 88 |
4 | 1.4 | [BMIm]BF4 | 96 | 94 |
5 | 1.5 | [BMIm]BF4 | 96 | 98 |
6 | 1.6 | [BMIm]BF4 | 80 | 93 |
7 | 1.5 | [BMIm]PF6 | 96 | 82 |
8 | 1.5 | [BMIm]OTf | 96 | 71 |
9 | 1.5 | [BMIm]Cl | 96 | 66 |
10 | 1.5 | [BMIm]Br | 96 | 69 |
11 | 1.5 | [BMIm]BF4 | 70 | 72 |
12 | 1.5 | [BMIm]BF4 | 60 | 54 |
13 | 1.5 | [BMIm]BF4 | 50 | 35 |
14d | 1.5 | [BMIm]BF4 | 96 | 7 |
15 | 1.5 | [Hmim]NO3 | 96 | 28 |
16 | 1.5 | PYR1n4TFSIe | 96 | 44 |
17 | 1.5 | C6(mim)2Cl2f | 96 | 39 |
a After 4 min.
b 100 mol%.
c Isolated yields.
d Under thermal condition.
e N-Butyl-N-methylpyrrolidinium bis(trifluoromethanesulfonyl)imide.
f 1,6-Bis(3-methylimidazolium-1-yl)hexane chloride.
First, different amounts of [HMIm]NO3 in the presence of [BMIm]BF4 were tested in this synthesis (Table 1, entries 1–6). Next, in order to show the effect of co-catalyst, the same reaction was performed with [Hmim]NO3 in the presence of different ionic liquids (Table 1, entries 7–10, entries 15–17). The experimental results persuaded us that combination of [HMIm]NO3 and [BMIm]BF4 at 96 °C is the most effective system for this transformation. It was found that increasing the temperature did not improve the reaction rate, while reducing the temperature led to lower yields. Among all tested systems, using 150 mol% of [HMIm]NO3 in [BMIm]BF4 (100 mol%) at 96 °C (200 Watt) was shown to be the best conditions (Table 1, entry 5). In order to show the effect of microwave irradiation, the same reaction was performed under traditional heating method and the product was obtained in only 7% yield (Table 1, entry 14). Using this optimized condition, a wide range of substituted and structurally diverse benzyl alcohols and acetophenones were subjected to this procedure to afford the corresponding products in high to excellent yields (Table 2).
Tandem synthesis of 2,4,5-triarylpyridines from benzyl alcohols and acetophenones promoted by [HMIm]NO3-[BMIm]BF4.
Entry | Ar | Ar’ | Y | Time (s) | Yield (%)a |
1 | C6H5 | C6H5 | COCH3 | 400 | 98 |
2 | 4-BrC6H4 | C6H5 | COCH3 | 420 | 99 |
3 | 2-FC6H4 | C6H5 | COCH3 | 480 | 97 |
4 | 4-ClC6H4 | C6H5 | COCH3 | 390 | 99 |
5 | 4-NO2C6H4 | C6H5 | COCH3 | 390 | 96 |
6 | 4-CH3C6H4 | C6H5 | COCH3 | 360 | 95 |
7 | 4-CH3OC6H4 | C6H5 | COCH3 | 370 | 94 |
8 | 2-naphtyl | C6H5 | COCH3 | 360 | 98 |
9 | 2-thiophenyl | C6H5 | COCH3 | 480 | 97 |
10 | 5-CH3-2-thiophenyl | C6H5 | COCH3 | 240 | 92 |
11 | C6H5 | 4-ClC6H4 | COCH3 | 220 | 99 |
12 | C6H5 | 4-CH3C6H4 | COCH3 | 390 | 92 |
13 | 2-CH3C6H4 | 4-CH3C6H4 | COCH3 | 420 | 95 |
14 | 4-CH3OC6H4 | 4-CH3C6H4 | COCH3 | 310 | 99 |
15 | 2-FC6H4 | 4-CH3C6H4 | COCH3 | 420 | 95 |
16 | 4-BrC6H4 | 4-CH3C6H4 | COCH3 | 300 | 98 |
17 | 4-NO2C6H4 | 4-CH3C6H4 | COCH3 | 330 | 96 |
18 | 2-naphtyl | 4-CH3C6H4 | COCH3 | 420 | 90 |
19 | 2-thiophenyl | 4-CH3C6H4 | COCH3 | 210 | 91 |
20 | 5-CH3-2-thiophenyl | 4-CH3C6H4 | COCH3 | 240 | 90 |
21 | C6H5 | 4-ClC6H4 | COCH3 | 220 | 96 |
22 | 2-CH3C6H4 | 4-ClC6H4 | COCH3 | 300 | 89 |
23 | 4-CH3OC6H4 | 4-ClC6H4 | COCH3 | 310 | 93 |
24 | 4-ClC6H4 | 4-ClC6H4 | COCH3 | 300 | 94 |
25 | 4-BrC6H4 | 4-ClC6H4 | COCH3 | 260 | 95 |
26 | 2-FC6H4 | 4-ClC6H4 | COCH3 | 360 | 89 |
27 | 2-naphtyl | 4-ClC6H4 | COCH3 | 420 | 91 |
28 | 2-thiophenyl | 4-ClC6H4 | COCH3 | 180 | 88 |
29 | 5-CH3-2-thiophenyl | 4-CH3C6H4 | COCH3 | 420 | 92 |
24 | 4-ClC6H4 | 4-ClC6H4 | COCH3 | 300 | 94 |
a Yields refer to isolated pure products characterized by NMR, IR and Mass spectroscopy [17–29].
As is evident from Table 2, besides benzyl alcohol (entry 1), other substituted aromatic methyl alcohols also served as good substrates for this reaction. For example, substituted benzyl alcohols (such as bromo, fluoro, chloro, nitro, methyl and methoxy) gave the desired triaryl pyridine in excellent yields (> 93%) (Table 2, entries 2–7). Also, polycyclic methyl alcohol such as 2-naphthylmethyl alcohol or heterocyclic ones (thiophen-2-yl or 5-methylthiophen-2-yl) also gave the expected products in high yields (Table 2, entries 8–10). This methodology could be successfully extended to different acetophenones either possessing electron-donating (4-methyl) or electron-withdrawing groups (4-chloro) on the phenyl ring (Table 2, entries 11–29).
To further study of the scope of the reaction, we were also examined tandem reaction of benzyl alcohol, 1-phenylethanol (instead of acetophenone) and ammonium acetate with [HMIm]NO3-[BMIm]BF4 under microwave irradiations. First, this transformation with different mole ratios of [HMIm]NO3 (1.0–3.0 mole) was carried out (Fig. 1).

Tandem reaction of benzyl alcohol, 1-phenylethanol and ammonium acetate with [HMIm]NO3-[BMIm]BF4 under microwave irradiations.
It was observed that increasing the concentration of [HMIm]NO3 caused a considerable increase in the conversion of these alcohols to 2,4,6-triphenylpyridine. Also, it was found that the best ratio of benzyl alcohol or 1-phenylethanol to [HMIm]NO3 was 1:2.5. It is noteworthy that the 2.5 molar ratio of [HMIm]NO3 is necessary in this reaction, since [HMIm]NO3 acts as oxidant in oxidation of benzyl alcohol and 1-phenylethanol in the first step. Therefore, according to this result, a series of benzyl alcohols and three different types of 1-arylethanol were treated with ammonium acetate using [HMIm]NO3-[BMIm]BF4 under microwave irradiations (Table 3, entries 1–15).
Tandem synthesis of 2,4,5-triarylpyridines from benzyl alcohols and 1-arylethanols promoted by [HMIm]NO3-[BMIm]BF4.
Entry | Ar | Ar’ | Y | Time (s) | Yield (%)a |
1 | C6H5 | C6H5 | CH(OH)CH3 | 490 | 95 |
2 | 2-CH3C6H4 | C6H5 | CH(OH)CH3 | 480 | 89 |
3 | 4-BrC6H4 | C6H5 | CH(OH)CH3 | 420 | 97 |
4 | 2-naphtyl | C6H5 | CH(OH)CH3 | 450 | 96 |
5 | 2-thiophenyl | C6H5 | CH(OH)CH3 | 270 | 92 |
6 | C6H5 | 4-CH3C6H4 | CH(OH)CH3 | 550 | 96 |
7 | 2-CH3C6H4 | 4-CH3C6H4 | CH(OH)CH3 | 510 | 93 |
8 | 4-BrC6H4 | 4-CH3C6H4 | CH(OH)CH3 | 420 | 94 |
9 | 2-naphtyl | 4-CH3C6H4 | CH(OH)CH3 | 510 | 98 |
10 | 2-thiophenyl | 4-CH3C6H4 | CH(OH)CH3 | 210 | 96 |
11 | C6H5 | 4-ClC6H4 | CH(OH)CH3 | 310 | 92 |
12 | 2-CH3C6H4 | 4-ClC6H4 | CH(OH)CH3 | 450 | 96 |
13 | 4-BrC6H4 | 4-ClC6H4 | CH(OH)CH3 | 300 | 91 |
14 | 2-naphtyl | 4-ClC6H4 | CH(OH)CH3 | 510 | 95 |
15 | 2-thiophenyl | 4-ClC6H4 | CH(OH)CH3 | 270 | 90 |
a Yields refer to isolated pure products characterized by NMR, IR and Mass spectroscopy [17–29].
As illustrated in Table 3, the corresponding products obtained in high to excellent yields and in short reaction times.
3 Experimental
3.1 General procedures
All chemicals were purchased from Merck chemical company. [HMIm]NO3 was synthesis according to the literature [40]. All products were identified by comparison of their physical and spectral data with those of authentic samples. Thin layer chromatography (TLC) was performed on UV-active aluminum-backed plates of silica gel (TLC Silica gel 60 F254). 1H NMR spectra were recorded (CDCl3 solution) on a Bruker DRX-500 Avance spectrometer. Chemical shifts (δ) are given in ppm relative to TMS. Coupling constants are given in Hz. Low-resolution mass spectra (LRMS) were recorded on a Bell and Howell 21-490 spectrometer. IR spectra were recorded on a Shimadzu IR-435 spectrophotometer. Melting points were determined using Stuart Scientific SMP2 apparatus. The microwave system used for these experiments includes the following items: Micro-SYNTH labstation, complete with glass door, dual magnetron system with pyramid-shaped diffuser, 1000 Watt delivered power, exhaust system, magnetic stirrer, “quality pressure” sensor for flammable organic solvents, ATCFO fiber optic system for automatic temperature control.
3.2 Typical procedure for the synthesis of 2,4,6-triphenylpyridine from benzyl alcohol and acetophenone
A mixture of benzyl alcohol (1 mmol), [HMIm]NO3 (1 mmol) and [BMIm]BF4 (1 mmol) was stirred under microwave irradiation (96 °C, 200 watt in Micro-SYNTH) for 200 s. Once conversion to benzaldehyde was completed (TLC), acetophenone (2 mmol) and ammonium acetate (3 mmol) were added and the reaction allowed to stir under microwave at the same temperature until the completion of the reaction. When the reaction was completed, quenched with ice-water (10 ml) and extracted with ethyl acetate (3 × 5 ml). The organic layer was dried (Na2SO4), concentrated under vacuum and recrystallized by 25% ethyl acetate/n-hexane to give 2,4,6-triphenylpyridine in 98% yield.
3.3 Typical procedure for the synthesis of 2,4,6-triphenylpyridine from benzyl alcohol and 1-phenylethanol
To a mixture of benzyl alcohol (1 mmol) and 1-phenylethanol (2 mmol) in [BMIm]BF4 (1 mmol), [HMIm]NO3 (2.5 mmol) was added. The reaction mixture was stirred in a microwave oven (MicroSYNTH) at 96 °C (200 watt). When conversion to the benzaldehyde and acetophenone were completed, as indicated by TLC, ammonium acetate (3 mmol) was added and the reaction allowed to irradiate under microwave condition. After completion of the reaction (490 s), the reaction mixture was poured into 10 ml ice-water and extracted with ethyl acetate for three times. The combined organic layer was washed with water and dried over Na2SO4. The solvent was evaporated and the pure 2,4,6-triphenylpyridine in 95% was obtained by recrystallization with 25% ethyl acetate/n-hexane.
3.4 Spectral data for selected compounds
2,6-diphenyl-4-(thiophen-2-yl)pyridine (Table 2, entry 9): mpt 154 °C. IR (KBr, cm−1) νmax: 3050, 1595, 1400, 760, 690. 1HNMR (500 MHz, CDCl3) δH: 8.21 (d, J = 13.7, 4H), 7.89 (s, 2H), 7.63 (d, J = 3.4, 1H), 7.54 (t, J = 7.35, 4H), 7.50–7.45 (m, 3H), 7.19 (t, J = 4, 1H). EIMS m/z 315, 314, 313, 312, 167, 149, 57. 4-(naphthalen-2-yl)-2,6-di-4-tolylpyridine (Table 2, entry 18). Mpt 124 °C. IR (KBr, cm−1) νmax: 3100, 3050, 2900, 2870, 1610, 1590, 1500, 810, 740. 1HNMR (500 MHz, CDCl3) δH: 8.22 (s, 1H), 8.15 (d, J = 8.05, 4H), 8.01–7.97 (m, 4H), 7.92 (t, J = 6.4, 1H), 7.88–7.86 (m, 1H), 7.58–7.55 (m, 2H), 7.34 (d, J = 8, 4H), 2.46 (s, 6H). EIMS m/z 385, 91, 77, 67, 57, 55. 2,6-bis(4-chlorophenyl)-4-(4-(phenoxymethyl)phenyl)pyridine (Table 2, entry 27): mpt 154 °C. IR (KBr, cm−1) νmax: 3120, 2900, 2800, 1580, 1340, 810, 770. 1HNMR (500 MHz, CDCl3) δH: 8.12 (d, J = 8.25 Hz, 4H), 7.82 (s, 2H), 7.69 (d, J = 8.35 Hz, 2H), 7.44–7.26 (m, 9H), 7.13 (d, J = 8.32, 2H), 5.16 (s, 2H). EMIS m/z 481, 188, 91, 77, 67, 65, 55, 51. 2,6-bis(4-chlorophenyl)-4-(5-methylthiphen-2-yl) pyridine (Table 2, entry 29): mpt 184 °C. IR (KBr, cm−1) νmax: 3118, 2912, 2803, 1595, 1490, 1400, 1070, 805, 770. 1HNMR (500 MHz, CDCl3) δH: 8.09 (d. J = 8.35, 4H), 7.75 (s, 2H), 7.48 (d, J = 8.35, 4H), 7.49 (d. J = 3.35, 1H), 6.84 (d, J = 2.53, 1H), 2.57 (s, 3H). EMIS m/z 396 (M++1), 395, 70,69,54,55.
4 Conclusion
This work demonstrates a novel, simple, efficient and green protocol by a microwave-promoted tandem process for the synthesis of 2,4,6-triarylpyridines using [HMIm]NO3-[BMIm]BF4 as a binary task specific ionic liquid. Moreover fast, clean and easy work-up of the expected products could be considered as other advantages of this method. To the best of our knowledge, this is the first example of tandem process for the synthesis of these pyridine derivatives under microwave irradiations. The use of [HMIm]NO3-[BMIm]BF4 makes this method a versatile alternative procedure for the synthesis of Kröhnke pyridines. Further studies to delineate of the mechanism and limitations of the present methodology are underway.
Acknowledgments
The authors are grateful to the Center of Excellence of Chemistry of University of Isfahan (CECUI) and also the Research Council of the University of Isfahan for financial support of this work.