1 Introduction
Surfactants are one of the most representative chemical products that are consumed in large quantities every day on a worldwide scale. Since it is known that surface-active compounds can adversely affect the aquatic environment, the biodegradability and biocompatibility of surfactants have become almost as important as their functional performance to the consumer. Because of this, there is a pressing need for developing efficient surfactants that are biodegradable and biocompatible.
Surfactants of this kind can be obtained by designing molecules that mimic natural amphiphilic structures (e.g., phospholipids [1], Nα-acyl amino acids [2], alkyl-glucosides [3]). Surfactant molecules from renewable raw materials that mimic natural lipoamino acids are one of the preferred choices for food, pharmaceutical and cosmetic applications. Given their natural and simple structure, they show low toxicity and quick biodegradation [4]. They can be produced by biotechnological and chemical methods using renewable raw materials such as amino acids and vegetable oils [5–11]. The value of amino acids as raw materials for the preparation of surfactants was recognized as soon as they were discovered early in the last century [12]. Initially they were used as preservatives for medical and cosmetic applications. Moreover, they were found to be active against various disease-causing bacteria, tumours, and viruses [13–15]. The combination of polar amino acids/peptides (hydrophilic moiety) and non-polar long chain compounds (hydrophobic moiety) for building up the amphiphilic structure has produced molecules with high surface activity. There is a large variety of amino acid/peptide structures. Moreover, the fatty acid chains can vary in their structure, length and number. These facts explain their wide structural diversity and different physicochemical and biological properties [4,9,16,17].
The amino acid or peptide moiety determines the main differences of adsorption, aggregation and biological activity between the amino acid/peptide-based surfactants. Hence, cationic, anionic, non-ionic and amphoteric surfactants can be obtained depending on the free functional groups. Further modification of these groups allows a fine-tuning of their properties to meet almost every particular application.
The amino acids and long aliphatic chains can be combined to each other to generate three main structures (Scheme 1) of amino acid-based surfactants, namely linear or single chain I, dimeric or gemini II and glycerolipid-like structures III. Linear structures I consist of an amino acid bearing at least one hydrophobic tail. Gemini or dimeric are amphipathic structures II with two polar heads (i.e., two amino acids) and two hydrophobic tails per molecule. Glycerolipid-like structures III can be considered analogues of mono-, diglycerides and phospholipids. They consist of one polar head and one or two hydrophobic moieties linked together through a glycerol skeleton.
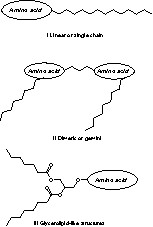
Structures of amino acid-based surfactants. The amino acid constitutes the polar head of surfactant. The hydrocarbon alkyl chain constitutes the hydrophobic moiety.
Our group has a wide experience on the synthesis by means of chemical, enzymatic or, usually, by a combination of both methodologies, of amino acid-based surfactants obtained from the condensation of natural saturated fatty acids, alcohols, amines and acyl glyceride derivatives with different amino acid head groups through ester and amide linkages [18–30]. Thus, saturated single-chain, double-chain, gemini and amino acid glycerolipid conjugate surfactants derived from amino acids of different ionic character have resulted to be, in all cases, highly biodegradable, with low toxicity, ecotoxicity and irritation effects. Water solubility and self-aggregation properties were directly associated with the chemical structure of the molecule. Being the cationic lipoamino acids the most antimicrobial active compounds. In this communication, we report some structure–activity relationship studies of amino acid-based surfactants of single chain and gemini structure for adsorption, self-assembling, and biological applications.
2 Single-chain amino acid/peptide surfactants
2.1 Synthesis
Linear amino acid surfactants are natural α-amino acids linked to long aliphatic chains through the α-amino, α-COOH or side chain groups (Scheme 2).
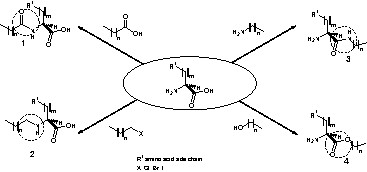
Different types of linkages between an amino acid and a hydrophobic alkyl chain: acyl-bond derivatives (1), alkyl-bond derivatives (2), amide-bond derivatives (3) and ester-bond derivatives (4).
Thus, fatty acids or alkyl halides can react with amino groups yielding the corresponding N-acyl and N-alkyl derivatives (Scheme 2, compounds 1 and 2), respectively. Alternatively, the carboxyl group of the amino acid can be condensed with alkyl amines or aliphatic alcohols to give N-alkyl amides and esters (Scheme 2, compounds 3 and 4) respectively.
Among the different types of linkages between the long aliphatic chain and the amino acid, the N-acyl (Scheme 3, series 1), N-alkyl amides (Scheme 3, series 2) and O- alkyl esters (Scheme 3, series 3) of arginine have attracted much interest to our group due to their low toxicity and high biodegradability in combination with their antimicrobial activity.
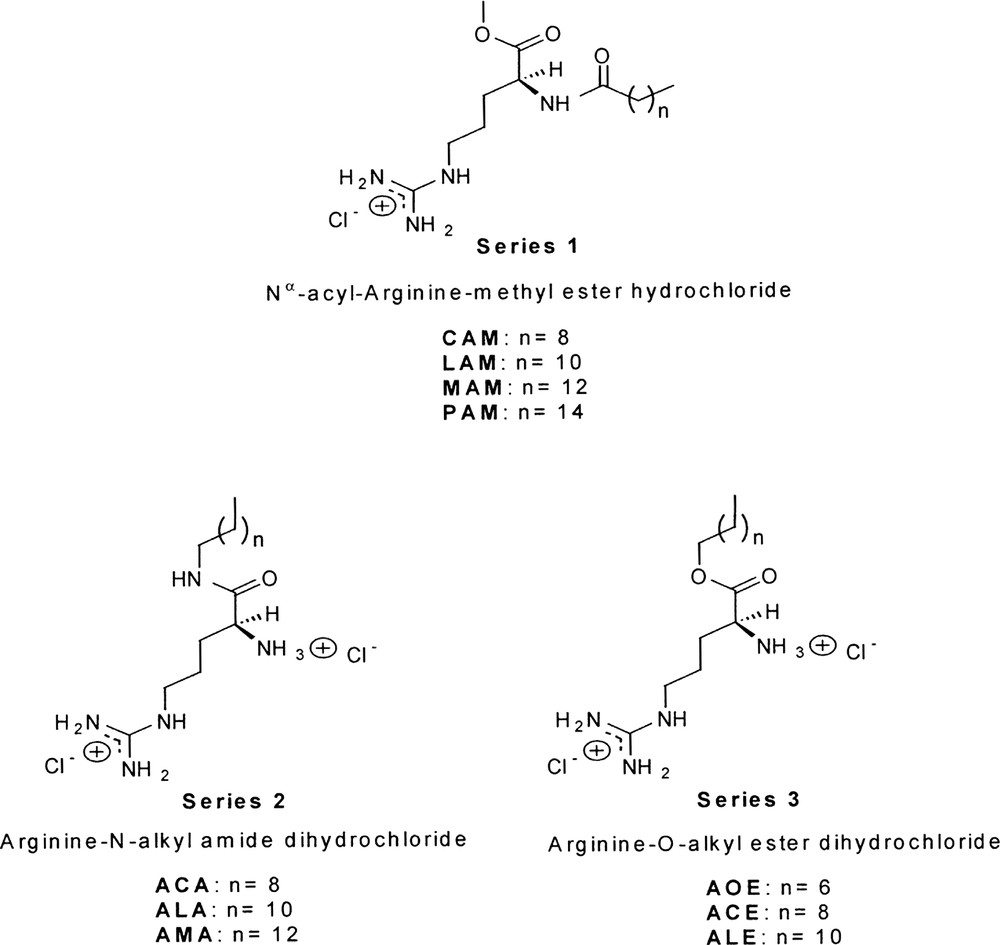
Chemical structure of single-chain arginine-based cationic surfactants.
The N-acylation of the amino terminal arginine (series 1) was prepared by condensation of fatty acids to arginine methyl ester hydrochloride using classical chemical methods [4]. The application of biotechnological procedures was not efficient for these compounds [30]. Series 2 was at first prepared by chemical procedures [25], however papain from Carica papaya latex was found to be a suitable catalyst for the formation of amide (series 2) and ester bonds (series 3) between Cbz–Arg–OMe and various long-chain alkyl amines and fatty alcohols [26]. In all cases, papain deposited onto polyamide was found to be the best biocatalyst configuration. The preparation of arginine alkyl esters was carried out in solvent free systems using the same alcohol reagent. Both series were enzymatic synthesized at multigram scale with a purity higher than 99%.
N-alkyl amide and ester derivatives of Nα-protected amino acids have also been prepared by lipases. In a study carried out with Candida Antarctica and Rhizomucor miehei lipases, it was found that these enzymes could readily catalyse the condensation of a number of Nα-Cbz-amino acids with α,ω-alkyldiamines or fatty alcohols [31].
2.2 Properties
Our group has reported that long-chain Nα-acyl arginine methyl ester compound (series 1, Scheme 1) are cationic surfactants with satisfactory toxicity profile, high biodegradability and a surface activity comparable to that of conventional long-chain quaternary ammonium salts. We have demonstrated that the morphology of their micelle aggregates and lyotropic phases depends on the hydrophobic moiety, temperature, composition and electrolyte content in the system. As a result, we have found that compounds of series 1 show a rich and unusual phase behaviour [32–34]. For instance, reversed vesicles (dispersion of lamellar liquid crystals in non-polar media) with biocompatible properties occurred in the lecithin–LAM/squalane system [35]. The PAM homologue was the only compound that showed lamellar lyotropic liquid crystals in the binary water/surfactant system [34]. These properties make them good alternatives for a wide range of industrial applications in the personal care, pharmaceutical and food sectors as well as in the design and synthesis of biomaterials. Furthermore, the arginine residue gives antimicrobial activity to the amphipathic molecule, a valuable property for a biocompatible surfactant [36].
Compared to series 1 the series 2 and 3 (Scheme 3) have two positive charged groups in the hydrophilic moiety, one in the primary amine and a second in the guanidine function. From the surface tension/concentration curves at 25 °C the critical micelle concentration which is associated to the hydrophobicity of the molecule (CMC), the maximum surface excess concentration at the air/aqueous solution interface (Γm) and the area per molecule whose value indicates the minimum area per surfactant molecule at the air/aqueous solution interface (Amin) were calculated. Table 1 summarises the surface parameters of the three series of compounds using the Gibbs adsorption equation [37]. In all cases they have the ability to decrease the surface tension of water until a constant value, γCMC and show a clear CMC in the surface tension/log C curves in the millimolar range. This indicates that they can be classified as surfactants with a surface activity similar to that of conventional cationic ones. Table 1 shows that the CMC depends on the straight alkyl chain and the nature of the hydrophilic moiety. For the three series of surfactants the larger the number of methylene groups in the alkyl chain the lower the CMC as it would be expected from the increase in the hydrophobic character of the molecule. The smaller the Amin, the more effective is its adsorption at the interfaces. We found that the Amin values for series 2 and 3 (62–114 × l0–2 nm2, and 96–122 × l0–2 nm2 respectively) were higher than that for the series 1 with the same alkyl chain length (67–62 × 10–2 nm2). This result indicates that the new molecules are less packed at the interface than those of series 1. The two charged groups in series 2 and 3 tend to spread them out on the interface due to an increase in the inter–intramolecular electrostatic repulsion forces.
Surface active properties of single chain surfactants from arginine at 25°C [32–34,49]
Compound | γcmca | CMCb | Γmc | Amind |
(10–3 N m–1) | (10–3 mol dm3) | (1014 mol m–2) | (102 nm2) | |
CAMe | 40 | 16 | ||
LAMe | 32 | 5.8 | 67 | |
MAMe | 32 | 2 | 62 | |
ACAf | 35 | 26 | 1.79 | 62 |
ALAf | 37 | 1.8 | 1.24 | 90 |
AMAf | 33 | 0.7 | 0.97 | 114 |
AOEg | 35 | 38 | 1.15 | 96 |
ACEg | 34 | 13 | 1.54 | 72 |
ALEg | 30 | 5 | 0.91 | 122 |
a Surface tension at the critical micelle concentration.
b Critical micelle concentration.
c Maximum surface excess concentration at the air/aqueous solution interface.
d Minimum area per surfactant molecule at the air/aqueous solution interface.
e Compounds of series 1 (Scheme 3).
f Compounds of series 2 (Scheme 3).
g Compounds of series 3 (Scheme 3).
The application of synthetic acyl amino acid/peptide vesicles as drug carriers as well as for the preparation of functional liposomes with lipopeptide ligands have been examined by several authors in the last years [38–39]. Vesicles of long-aliphatic-chain N-acyl amino acids showed encapsulation efficiencies for solutes comparable to that of conventional liposomes of lecithin. Recently, a new technology has emerged for the transfer of foreign DNA into cells forming non-toxic hydrophobic ion-paired complexes between long-chain arginine alkyl esters (Scheme 1, series III) with DNA [40]. Lipoamino acids are also particularly attractive as antiviral agents. Certain acyl amino acid derivatives have been found to produce inhibition on influenza neuraminidase [41]. A number of Nα-palmitoylated amino acids/peptides have been incorporated into model membranes affecting the transition temperature between the bilayer to hexagonal aggregation, a property associated with antiviral activity against cantell strain of Sendai virus (parainfluenza type 1) [42].
One important milestone in our research is the design and development of new amino acid-based surfactants with antimicrobial properties, which mimic natural amphiphilic cationic peptides [20,43]. To this end, Lys and Arg derivatives of long chain Nα-acyl, COO-ester and N-alkyl amide have been prepared. In particular, the Nα-acyl arginine methyl ester derivatives series 1 (Scheme 2) have turned out to be an important class of cationic surface active compounds with a wide bactericidal activity, high biodegradability and low toxicity profile. The antimicrobial activities were determined ‘in vitro’ on the basis of the minimum inhibitory concentration (MIC) values, defined as the lowest concentration of antimicrobial agent that inhibits the development of visible growth after 24 h of incubation at 37 °C. We have shown that essential structural factors for their antimicrobial activity include both the length of the fatty residue (akin with their solubility and surface activity) and the presence of the protonated guanidine function [43,44]. Amphoteric lipopeptide surfactants with antimicrobial activity comparable to those of LAM were found when neutral Gly or Phe amino acids were condensed to the Nα-lauroyl arginine [9]. More interestingly, condensation of a Nα-acyl-arginine residue to an acid-hydrolysed collagen gave rise to a family of amphoteric protein-based surfactants with antimicrobial activity being the homologue of C14 carbon atoms the most active [9]. The activity of all these amphoteric N-acyl arginine surfactants could be due to the presence of the long chain N-acyl-arginine residue and to the absence of intramolecular ionic interactions in the molecule. The free guanidine group together with the surface activity of these compounds could interact with the polyanionic components of the cell surface triggering the biocide mechanisms of these surfactants. In accordance with Ferguson's principle [45], the antimicrobial activity might be related to the combination of several physicochemical properties such as surface activity, adsorption and solubility.
The ultimate biodegradability [46] measurements for the three series of arginine-based cationic surfactants showed that all homologues (except AMA) can be considered biodegradable. Interestingly, using ester type bonds (series 3, Scheme 2) to link the hydrophobic and hydrophilic moieties accelerates their biodegradation considerably. This fact has also been described for sugar-based surfactants [47].
The haemolytic activity, HC50, which is the concentration of surfactant that causes 50% of haemolysis of red blood cells from healthy human donors [48] and the HC50/D ratio, where D is the haemoglobin denaturising index (DI), are the parameters commonly measured for evaluating the potential toxicity of the surfactants. The HC50/D or L/D is used for predicting the potential ocular irritation related to the sodium dodecyl sulphate (SDS) compound (L/DSDS: 0.44; irritant). The values of HC50 for series 1, 2 and 3 (Scheme 2) demonstrated that these compounds can be considered as non-haemolysing agents (HC50 < 1000 μg ml–1). For comparison’s sake, commercial cationic surfactants have HC50 ranging from 4 to 15 μg ml–1. Furthermore, according to the results of the L/D ratio first and by the in vivo eye irritation Draize test later, these linear arginine-based surfactants have no irritant effect in the eyes (non eye-irritants, L/D > 100) [49].
3 Amino acid-based gemini surfactants
Gemini surfactants are a novel class of amphipathic compounds consisting of two hydrophilic and two hydrophobic groups per molecule, linked through a spacer chain. These molecules can be considered dimmers of the single chain conventional surfactants of one hydrophilic and one hydrophobic group. Their interest lies on the number of unexpected surface activity properties, which makes them superior to the conventional surfactants. These molecules show extremely low critical micelle concentration values (CMC, a fundamental parameter of surfactants close related with their hydrophobicity), solubilizing, wetting, foaming, antimicrobial and lime soap dispersion properties [50–57].
3.1 Synthesis
An obvious strategy to increase the efficiency of cationic surfactants and reduce their environmental impact and potential toxicity is to build up gemini structures from environmentally friendly single chain arginine-based surfactants. To this end, our group has chemically synthesized and studied a new class of gemini cationic surfactants derived from the arginine: the Nα,Nω-bis(Nα-acylarginine)α,ω-alkylendiamides or bis(Args) [24,58–59]. These compounds consist of two symmetrical long-chain Nα-acyl-l-arginine residues of twelve, Nα,Nω-bis(Nα-lauroylarginine)α,ω-alkylendiamides [Cn(LA)2 series], and ten carbons atoms, Nα,Nω-bis(Nα-caproylarginine)α,ω-alkylendiamides [Cn(CA)2 series], linked by amide bonds to an α,ω alkylenediamine spacer chain of varying length (n = 2–10) (Scheme 4 left). This particular alkylenediamine spacer chain was chosen to control the distance between the charged sites of the molecule that modify the inter- and intra-hydrophilic–hydrophobic interactions. Bis(Args) were investigated in an attempt to develop a new class of environmentally friendly amino acid-based surfactants with surface activity exceeding that of CAM and LAM, and with at least the same antimicrobial, toxicity and biodegradability properties.
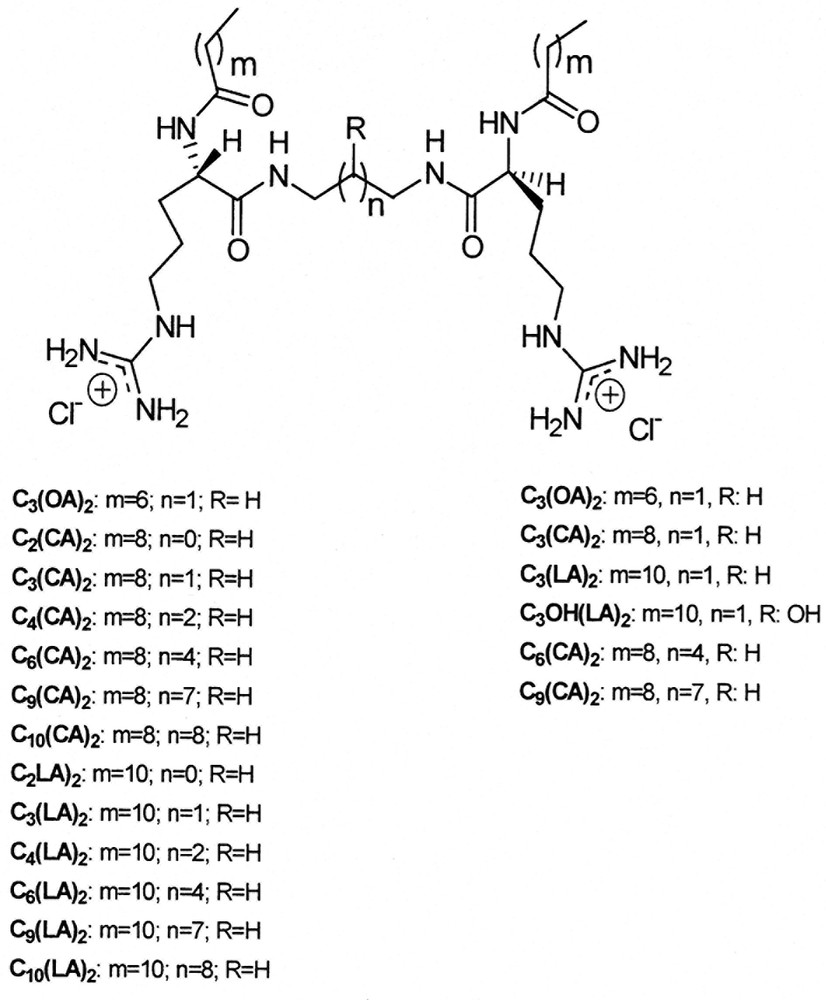
Structures of bis(Args) gemini cationic surfactants prepared by chemical procedures (left) and chemo-enzymatic procedures (right).
Recently a series of bis(Args) have been prepared at multigram scale by a chemoenzymatic approach using papain deposited onto Celite for the best results [27]. (Scheme 4, right).
3.2 Properties
In the light of our studies, it can be concluded that for most of the properties gemini surfactants are superior to the corresponding conventional monomeric surfactants. They were found to be more efficient surface-active molecules than the single chain structures: about three orders of magnitude for the equilibrium surface tension and about 20 times for the tension equilibration [58] and foam stability [60].
The dilution antimicrobial susceptibility test was carried out and the minimum inhibitory concentration (MIC) values were determined. Bis(Args) exhibited a broad spectrum of preservation capacity at MIC values in the range from 4 to 125 μg ml–1 [23]. The dimerization enhanced the antimicrobial activity for the geminis Cn(CA)2 compared with CAM. Given the peculiar structure of bis(Args), the compounds of the Cn(CA)2 series have a hydrophobicity that is more than twice the hydrophobicity of CAM. However, the presence of two ionic arginine groups in one molecule of Cn(CA)2 can make a positive contribution to the degree of the hydration of this series, showing a water-solubility similar to that of CAM. These two characteristics in the molecule can result in a more effective adsorption and diffusion of Cn(CA)2 on the cell interface, resulting in an antimicrobial action at lower concentrations.
Acute toxicity tests on freshwater crustacea (Daphnia magna), a very sensitive invertebrate [61], as well as on saltwater bacteria [62] (Photobacterium phosphoreum) were carried out to assess the aquatic toxicity. These standard tests represent two of the trophic levels that can be exposed to the cationic surfactants. Concentration values that cause inmobilization in 50% of the Daphnia after 24-h exposure (IC50) and 50% reduction in the light emitted by the bacteria after 30-min exposure (EC50) were determined. Values of IC50 and EC50 for the bis(Args) together with those of LAM and CAM are summarised in Table 2 [63]. Values reported for two series of conventional mono(Quats) DTAB and HTAB are also indicated. When increasing the hydrophobicity of the molecule, the acute toxicity raised for each series of surfactants in agreement with their CMC values, Table 3. Thus, Cn(CA)2 were less toxic than the Cn(LA)2 compounds due to their lower hydrophobic character. Interestingly, IC50 values for Cn(CA)2 compounds were similar to that of LAM. Furthermore, all of them were one order of magnitude less toxic than the conventional mono(Quats).
Aquatic toxicity values of Cn(LA)2, Cn(CA)2 series and CAM, LAM, DTAB and HTAB [63]
Compounds | Daphnia magna IC50a (mg l–1) | Photobacterium phosphoreum EC50b (mg l–1) | ||
mean | 95% confidence range | mean | 95% confidence range | |
C2(LA)2 | 4.4 | (3.5–5.3) | 28 | (18–43) |
C3(LA)2 | 2.1 | (1.8–2.4) | 2.4 | (2.2–2.7) |
C4(LA)2 | 4.6 | (3.9–5.2) | 5.8 | (4.3–8.0) |
C6(LA)2 | 2.4 | (1.9–2.5) | 3.0 | (2.0–4.5) |
C9(LA)2 | 2.2 | (1.9–2.5) | 13 | (10–17) |
C10(LA)2 | 16 | (11–20) | 20 | (15–28) |
LAM | 15 | (12–18) | 12 | (10–14) |
DTAB | 0.38 | (0.36–0.40) | 0.24 | (0.20–0.30) |
HTAB | 0.13 | (0.11–0.15) | 0.63 | (0.40–0.98) |
C2(CA)2 | 16 | (11–20) | 1.5 | (1.2–1.9) |
C3(CA)2 | 16 | (11–20) | 1.1 | (0.5–2.1) |
C4(CA)2 | 12 | (7–17) | 0.9 | (0.4–2.2) |
C6(CA)2 | 15 | (11–19) | 1.3 | (0.3–5.6) |
C9(CA)2 | 5.5 | (2.7–8.2) | 1.1 | (0.7–1.7) |
C10(CA)2 | 7.5 | (5.0–10) | 2.7 | (1.2–5.8) |
CAM | 77 | (56–98) | 4.0 | (3.1–5.3) |
a Concentration values that cause 50% inhibition in the crustacean mobility after 24 h of exposure.
b Concentration values that cause 50% reduction in the light emitted by the bacteria after 30 min of exposure.
CMC values (mg l–1) and HC50 (mg l–1) of CAM, LAM and bis(Args) homologues on human red blood cells [63]
Compound | CMCa (mg l–1) | HC50b (mg l–1) |
CAM | 6056 | 38.5 |
C3(CA)2 | 1533 | 110.5 |
C6(CA)2 | 1294 | 9.0 |
C9(CA)2 | 935 | 8.7 |
LAM | 2439 | 20.8 |
C3(LA)2 | 460 | 80.7 |
C3(OH)(LA)2 | 5887 | > 200 |
C3(OA)2 | 49 770 | > 200 |
a Critical micelle concentration.
b Hemolysis value.
Due to the complexity and hydrophobicity of the gemini compared with the single chain structures, the biodegradation rate of single chain structures such as LAM (90% in 14 days), was higher than that of the bis(Args) (50–90% in 14 days). The biodegradation rate of bis(Args) decreased when both the spacer chain and the alkyl chain length increased. Hence, the higher the hydrophobicity of the surfactants, the lower their biodegradation rate [63].
The haemolysis test showed again that the highest HC50 values were obtained for the compounds with the highest hydrophobic character, namely, those with the longest alkyl and spacer-chain lengths. There is considerable difference between the HC50 of these new gemini surfactants and those bearing a quaternary ammonium group at the polar head. MonoQuats have HC50 values between 0.05 and 0.1 μg m–1. The introduction of a hydroxyl function to the spacer chain make the compound more hydrophilic, in consequence the CMC increases and the HC50 increases.
Dimerization of LAM and CAM yields environmentally friendly antimicrobial gemini surfactants with lower haemolytic activity, aquatic toxicity and efficient surface activity than other cationic surfactants, (i.e. monoQuats). The increase of hydrophobicity of these molecules is a negative structural parameter for their environmental behaviour.
In summary, amino acid-based surfactants constitute a class of bio-based surfactants with excellent surface properties, wide biological activity, low potential toxicity and low environmental impact. Moreover, they can be prepared efficiently by chemical and enzymatic catalysis. All these features make them an outstanding clean and safe alternative to conventional specialty surfactants. Hence, these new generations of amino acid-based surfactants will contribute to meet the increasing demand of environmentally friendly surfactants for pharmaceutical and food industries.
Acknowledgements
This work was supported by the MEC, Projects QUI97-0570 and PPQ2000-1687-CO2–01 and UA-CSIC Interaccion de Tensioactivos con membranas.