1 Introduction
In recent years, it has been established that small organic molecules, in addition to metal complexes and biocatalysts, can be highly selective and efficient catalysts. As a consequence, organocatalysis is gaining importance in asymmetric synthesis, complementing bio- and metal-catalysis [1,2].
On the other hand, the development of water as a green medium for organic synthesis has become an important research area. Other than the economical and environmental benefits of using water, it may exhibit unique reactivity and selectivity in comparison with conventional organic solvents. Therefore, development of simple reactivity and selectivity that cannot be attained in conventional organic solvents is one of the challenging goals of aqueous media [3–6].
Nitrogen and sulfur-containing motifs are ubiquitous in natural products [7,8] biologically active molecules [9], and important synthetic intermediates for various pharmaceuticals and natural products [10–12].
Given the widespread availability of sulfur and nitrogen containing nucleophiles and α,β-unsaturated alkenes, there is substantial interest in developing efficient Michael addition from these simple starting materials. Thus, several efforts have been made to develop newer and simpler methodologies for thia-and aza-Michael addition that lead to the development of various base and acid catalysts and novel reaction media such as water and ionic liquids [13–53].
2 Results and dissociation
As a part of our research, aimed at developing green chemistry by using water as reaction medium or by performing organic transformations under solvent-free conditions [54–58], herein, we describe the use of simple small organic catalysts capable of promoting Michael addition of aromatic, aliphatic thiols and aromatic amines in water.
As a model reaction, benzylideneacetone was reacted with thiophenol with different loading of starting materials. It was found that by simple mixing of benzylideneacetone (1 mmol), and thiophenol (1 mmol), in the presence of squaric acid (5 mg) in water (2 mL) the desired products was obtained in 97% yield (Scheme 1).
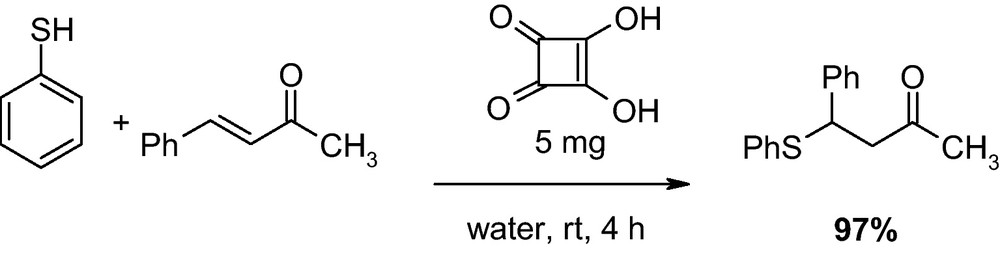
The optimized reaction conditions for Michael addition in water.
With the optimized reaction conditions in hand, the scope of the reaction was explored with different substances. Fortunately, these results proved to be quite general and a wide range of structurally different thiols underwent Michael addition with several Michael acceptors such as α,β-unsaturated ketones, esters and nitriles affording the corresponding products in quantitative yield. cyclohexenone, methyl vinyl ketone, chalcone, methyl acrylate, acrylonitrile, β-nitrostyrene and benzylideneacetone underwent 1,4-addition with a wide range of thiols, such as aliphatic, substituted aromatic thiols bearing an electron-withdrawing and electron-donating groups in the presence of very small amount of squaric acid in water at room temperature to give the corresponding products in high yields. The results are summarized in Table 1.
Reaction of thiols with Michael acceptors in water.
Entry | Thiol | Michael acceptor | Yield [%] | Time [min] |
1 | 2a | 90 | 240 | |
2 | 2b | 92 | 240 | |
3 | 2c | 85 | 240 | |
4 | 2d | 60 | 240 | |
5 | 2e | 78 | 240 | |
6 | 2f | 90 | 240 | |
7 | 2a | 97 | 240 | |
8 | 2b | 90 | 240 | |
9 | 2c | 90 | 240 | |
10 | 2e | 65 | 240 | |
11 | 2f | 82 | 240 | |
12 | 2a | 97 | 60 | |
13 | 2b | 95 | 80 | |
14 | 2d | 90 | 100 | |
15 | 2f | 92 | 80 | |
16 | 2a | 92 | 120 | |
17 | 2b | 84 | 120 | |
18 | 2c | 80 | 120 | |
19 | 2a | 92 | 150 | |
20 | 2b | 97 | 150 | |
21 | 2c | 80 | 150 | |
22 | 2a | 95 | 150 | |
23 | 2b | 95 | 150 | |
24 | 2c | 92 | 150 | |
25 | 2a | 90 | 80 | |
26 | 2b | 85 | 80 | |
27 | 2c | 82 | 80 |
To further explore the scope of this simple protocol, a number of electron-deficient olefins and aromatic amines were also examined under the optimized conditions (Table 2). Several aromatic amines underwent smooth addition with α,β-unsaturated ketones, nitriles and esters in water. In all cases, the reactions proceeded smoothly and gave the corresponding products in good to excellent yield. As evident from the results, anilines bearing either electron-donating or electron-withdrawing groups give the Michael addition products in good yields.
Reaction of aromatic amines and Michael acceptor in water.
Entry | Michael acceptor | Aromatic amine | Yields (%)a | |
1 | a R=H | 90 | ||
2 | b R=n-butyl | 86 | ||
3 | c R=isopropyl | 88 | ||
4 | d R=OMe | 94 | ||
5 | e R=Cl | 70 | ||
6 | f R=Br | 72 | ||
7 | g R=H | 92 | ||
8 | h R=n-butyl | 84 | ||
9 | i R=isopropyl | 82 | ||
10 | j R=OMe | 80 | ||
11 | k X=Cl | 64 | ||
12 | l X=Br | 68 | ||
13 | l R=H | 92 | ||
14 | m=isopropyl | 82 | ||
15 | n R=OMe | 80 | ||
16 | o X=Cl | 64 | ||
17 | p R=H | 88 | ||
18 | q X=Cl | 62 | ||
19 | r R=H | 64b | ||
20 | s X=OMe | 70b |
a NMR yields b In the cases of X=Cl and Br, reaction run at 60 °C.
As shown in Table 2, the yields of the products are affected by the nature of the primary amine. In the case of aromatic amines with an electron-donating group, such as 4-isopropyl aniline, 4-methoxyaniline, the corresponding products were obtained in good to high yields. Aromatic amines with an electron-withdrawing group, such as 4-nitroaniline, are not good substrates for this reaction and give the corresponding products in very low yields.
The role of the squaric acid as catalyst is still not clear [59–61].1 Brønsted acidity is the main factors that influence the reactivity and selectivity of the process. We tentatively propose the mechanism of the present reaction to proceed in a manner similar to that described the analogous Brønsted acid-catalyzed oxa-Michael addition by Johnson's group, is outlined in Scheme 2 [62]. The carbonyl oxygen of 1a accepts one proton from the squaric acid to give the protonated form and undergoes thia-Michael addition with thiophenol to give the desired products.
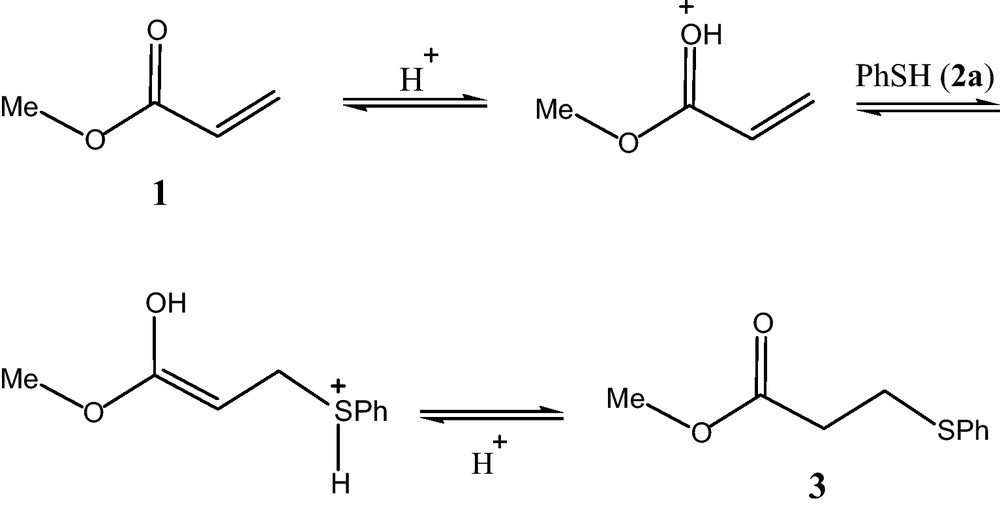
Plausible reaction mechanism in the presence of squaric acid.
3 Conclusion
In summary, an operationally simple and green aza- and thia-Michael addition of aromatic and aliphatic thiols and amines to electron-deficient olefins under solvent free conditions with good to excellent yields has been developed. This new protocol has the advantages of environmental friendliness, higher yields, shorter reaction times, and convenient operation, which only require stirring the reaction mixture at ambient temperature without the use of any additional energy source like heating or sonication. Moreover, the purification process is simple which is just a quick washing of the reaction mixture with water, which allows the isolation of the desired products in good to excellent yields.
4 Experimental
4.1 General methods
1H NMR spectra were recorded on 500 MHz NMR Bruker spectrometer and 13C NMR spectra were recorded on 125 MHz NMR Bruker spectrometer respectively using CDCl3 or DMSO-d6, as solvent. Chemical shifts are given in ppm. All amines, thiols, catalyst and Michael acceptor are commercially available and were purchased and used without further purification; water and other solvent were distilled before used.
4.2 General procedure of Michael addition of enones with thiols and amines in water
To a stirred solution of Michael acceptor (1 mmol), squaric acid (5 mg) and water (2 mL) in the test tube, thiols or amines (1 mmol) was added and the resulting mixture was stirred at room temperature for 60 to 480 min. After completion of reaction, in the cases of solid products, pure products were obtained with only washing with water and ethanol. Further purification was carried out by short column chromatography on silica gel (ethyl acetate/petroleum ether). The product was analyzed by 1H and 13C NMR spectrometry and melting points and all compounds were characterized on the basis of their spectroscopic data by comparison with those reported in the literature (Table 3).
Thia-Michael addition of thiophenol with methyl acrylate with different catalyst system.
Entry | Catalyst | Mol (%) | Solvent | Yields (%) | Ref. |
1 | – | – | Water | 95 | [16] |
2 | Montmorillonite | 10 | SFC | 92 | [17] |
3 | CAN | 50 | SFC | 99 | [18] |
4 | Zn(ClO4) | 1 | SFC | 88 | [26] |
5 | – | – | PEG | 94 | [37] |
6 | Bi(OTf)3 | 1 | CH3CN | 78 | [38] |
7 | SSA | 25 | SFC | 93 | [39] |
8 | HClO4-SiO2 | 1 | SFC | 85 | [44] |
9 | Cu(BF4)2 | 1 | SFC | 88 | [45] |
10 | FeCl3 | 2 | SFC | 88 | [47] |
11 | ZrCl4 | 1 | SFC | 92 | [48] |
12 | IL | 100 | IL | 93 | [49] |
13 | β-CD | 100 | Water/acetone | 98 | [50] |
14 | Silica nanoparticle | 2 | SFC | 88 | [51] |
15 | B(OH)3 | 10 | Water | 89 | [52] |
16 | n-(Bu)4Br | 15 | SFC | 92 | [53] |
17 | SQ | 0.1 | Water | 95 | – |
Acknowledgment
Financial support of this work by Chemistry and Chemical Research Center of Iran is gratefully appreciated.
1 Although squaric acid has been known as a commercially available strong acid, squaric acid itself has attracted little attention from the synthetic community mainly due to its extremely poor solubility in organic solvents [59,60]. Recently, some groups have developed a new chiral Brønsted acid from the squaric acid scaffold and successfully used in asymmetric organic synthesis [61].