1 Introduction
Ionic liquids have been mainly considered as new reaction media, as catalysts, and as electrolytes for various battery systems because of their unique electrochemical and physical properties [1,2]. Recently, considerable research on nanomaterials has been attempted for room temperature ionic liquids (RTILs) because RTILs generally feature a good stability to air and water, a wide liquid range and unique stability properties to various organic and inorganic substances [3–5]. ILs are ionic compounds with relatively low melting point. They are characterized by very low vapor pressure and consequently, are considered as environmentally benign solvents. In addition, ILs possess interesting properties such as tunable polarity, Lewis acidity, coordinating ability, hydrophilic and miscibility with various compounds [6–8].
Ionic liquids have been used in the synthesis of nanostructured materials, and identification of appropriate surface capping agent in these new solvents remains an important issue. Several currently used capping agents tend to causes irreversible aggregation of nanoparticles [9,10]. Reverse microemulsions are mostly transparent, isotropic and thermodynamically stable liquid media with nanosized water droplets dispersing in continuous oil phase and stabilized by an adsorbed surfactant film at the liquid–liquid interface [11].
Nanoparticles have a wide range of applications in the field of environmental pollution control, drug delivery systems, material chemistry and so on [12]. There has been extensive research in chemical synthesis of nanoparticles. The chemical synthesis of nanoparticles has several occupational exposure hazards like carcinogenicity, genotoxicity, cytotoxicity and general toxicity [13]. Silver is chosen in part because of its size dependent surface plasmon and other unique properties, which have found increasingly broad applications [14–16]. Generally, metal nanoparticles can be prepared by both physical and chemical methods; however, the chemical approach, such as reduction, is most widely used [17,18]. The recent advances in research on metal nanoparticles appear to revive the use of silver nanoparticles for antibacterial applications. It has been shown that silver Nan particles (SNPs) prepared with a variety of synthetic methods have effective antimicrobial activity [19–27]. Hence, silver SNPs have been applied to wide range of healthcare products, such as burn dressings, scaffolds, water purification systems and medical devices [28,29].
The interest towards preparation of such hybrid materials is driven by their possible application in the biomedical field as antibacterial coating material or in the electrochemical biosensor field as a catalytically active membrane for direct electron transfer [30]. In conclusion, combined results suggested that SNPs may damage the structure of bacterial enzymes, which cause bacterial cells to die eventually. All studies have shown that the size morphology, stability and properties of metal nanoparticles are strongly influenced by experimental conditions, the kinetics of interaction of metal ion with reducing agent, and adsorption process of stabilizing agent with metal nanoparticles. Hence the design of the synthetic pathway in which the properties of nanoparticles are well controlled has become a major field of interest.
In this study, the synthesis of SNPs is done using a simple RTIL which is newly synthesized and which acts as a stabilizing agent and hydrazine hydrate as reducing agent. The role of the ionic liquid stems from the fact that the thermodynamically driven spontaneous process of nanoparticles agglomeration can be prevented. The reducing ability depends on the concentration of reducing agent. Thus by optimizing the experimental conditions such as silver ion concentration, IL concentration, hydrazine concentration, time, monodispersed silver nanoparticles with high antibacterial activity could be obtained.
2 Experimental
2.1 Materials
The chemicals such as silver nitrate, hydrazine hydrate, 1-bromododecane, 2-amino pyridine were obtained from Merck India. All materials were used without further treatment.
The nutrient agar, Type 1 agar and sodium chloride was purchased from Hi-media. These agars were used to grow and maintain the bacterial culture.
2.2 Synthesis of 2-amino-1-dodecylpyridinium bromide
To a stirred solution of 2-amino pyridine (10 mmol) in ethanol (10 mL), 1-bromodo-decane (15 mmol) was added (Scheme 1). The reaction mixture was stirred at 60–65 °C for 48 h. The formed solid product was washed repeatedly with ethyl acetate, filtrated to remove non-ionic residues and dried in vacuum to get the product 2-amino-1-dodecylpyridinium, bromide. The NMR spectroscopic data is used to confirm the formation of ionic liquid.

NMR Data
1H NMR (300 M Hz, DMSO d6): δ = 0.83 (t, 3 H), 1.21 (m, 18 H), 1.69 (m, 2 H), 1.16 (t, 2H) 6.85 (t, 1H), J = 8.4 Hz 7.12 (d, 1 H), J = 8.7 Hz, 7.85 (t, 1 H) J = 6.6 Hz 7.95 (d, 1 H) J = 6.3 Hz 8.59 (bs, –NH2, 2 H).
13 C NMR (75 MHz, DMSO-d6): 14.36, 22.53, 25.79, 27.81, 29.07, 29.15, 29.31, 29.37, 29.45, 31.73, 53.42, 113.30, 115.22, 140.45, 142.54, 153.92.
2.3 Synthesis of silver nanoparticles
In typical synthesis 0.1 g 2-amino (do-decyl) pyridinium bromide was dissolved in 10 mL distilled water in an Erlenmeyer flask. To this solution 1 mmole silver nitrate aqueous solution was added and the whole solution stirred for 10 min. After 10 min of mixing of both these solution, 1 mL dilute hydrazine hydrate was added. The solution turns pale yellow to yellow brown in color, indicating formation of silver nanoparticles. A slight excess of hydrazine hydrate was added to confirm complete reduction. The silver nanoparticles were stabilized by reverse micelle system AgNO3/Water/IL and the product was collected by centrifugation. Reaction conditions were optimized by checking the stability and particle size of SNPs.
The synthesis of silver nanoparticles was confirmed by UV-Visible spectroscopy. The UV-Visible spectra shows a surface plasmon resonance (SPR) at about 420 nm. The UV-Visible spectrum of Water/IL system and IL stabilized silver nanoparticles was recorded. Only IL stabilized silver nanoparticles show SPR indicating formation of SNPs. The kinetic study of reaction time at fixed Ag+ and IL concentration were recorded. All UV-Visible analysis was carried out with Shimadzu UV-Visible NIR spectrophotometer (model-3600). Distilled water was used as blank.
Particle size of synthesized silver nanoparticles was analyzed through photon correlation spectroscopy. Light scattering measurements were carried out at 90°, on a photon correlation spectrometer (PCS)–Zetasizer 3000 HAS equipped with a digital autocorrelation from Malvern Instrument UK. The transmission electron diffraction (selected area electron diffraction [SAED]) pattern was taken for morphological analysis of nanoparticles with JEOL-3010 field emission electron microscope with accelerating voltage of 300 kV. The samples were analyzed by preparing a dilute solution in distilled water, drop casted on a carbon coated copper grid, followed by drying the sample at ambient conditions before it was attached to the sample holder on the microscope. Particle size distribution (PSD) of same sample after 10 months shows no change in particle size which indicates the particles were more stable and do not agglomerate.
2.4 Bactericidal activity (antimicrobial activity)
Three different groups of bacteria Escherichia coli (gram negative bacteria) Staphylococcus aureus (gram positive bacteria), and Pseudomonas aeruginosa (non ferment gram negative bacteria) were investigated in this study. Nutrient agar and type-I agar were used as media to grow bacteria. The bacterial strains were stored in refrigerator. The bacterial solutions were prepared in 0.86% saline. The antibacterial activity of silver nanoparticle was assayed by following standard Nathan's agar well diffusion technique [31]. The bacterial suspension was spread on nutrient agar in petri plates to create confluent lawn of bacterial growth. The wells of 6 mm were prepared by a borer. The solutions of different concentration (10, 15 and 20 μl) were poured into each well. The well without silver nanoparticles was treated as control. These plates were incubated to 24 h at 35 °C. The lowest concentration at which the petri plate did not show any visible growth after microscopic evaluations was considered as the minimum inhibitory concentration (MIC). The susceptibility of test organisms was determined after 24 h by measuring the zone of inhibition around each well to the nearest mm. The results of antimicrobial activity were compared with the control experiment. The control is having 0.1 g IL and 10 mL water (10 μl is used in actual experimentation). This is the same as that used in the synthesis of silver nanoparticles. No zone of inhibition was observed; indicating that the antimicrobial activity is due to IL stabilized SNPs and not due to the IL. All antimicrobial parameters have been studied in triplicate.
3 Results and discussion
Colloidal dispersion of SNPs was successfully prepared by the reduction of silver nitrate by hydrazine hydrate in water ionic liquid micro emulsion. Water-IL micro emulsion containing Ag+ ions is slowly turned brownish yellow in color within 2 h after the hydrazine hydrate reduction was started. The micro emulsion phase containing SNPs was highly dispersed in water IL phase. Fig. 1 shows samples of ionic liquid and IL stabilized SNPs.
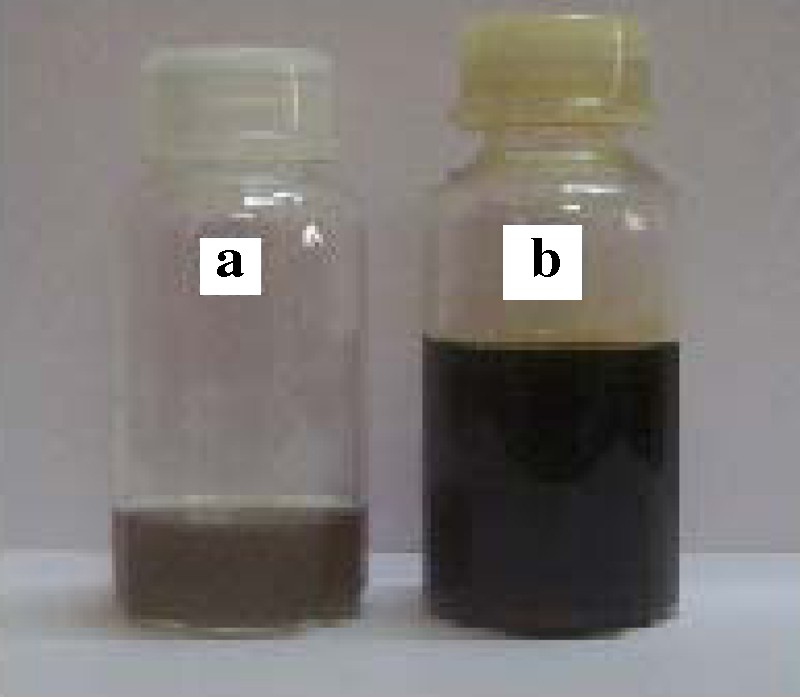
(a) Ionic liquid stabilized silver nanoparticles. (b) Ionic liquid.
Fig. 2 shows a schematic representation of the possible formation of SNPs. The water-IL phase shows clear micro emulsion; it consists of micelle. This micelle will trap the Ag+ ions from aqueous solution of AgNO3. The reduction of Ag+ ions to Ag0 takes place by hydrazine hydrate. This is indicated by the color change from colorless to yellowish brown. This color arises due to surface plasmon vibrations of metal nanoparticles.
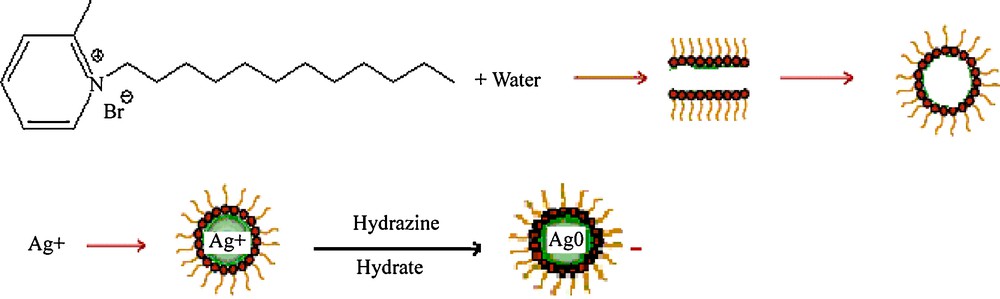
Schematic illustration of probable formation of silver nanoparticles.
Fig. 3a shows UV-Visible spectra of water IL micro emulsion and IL stabilized silver nanoparticles. The IL stabilized SNPs shows SPR at 430 nm indicating formation of silver nanoparticles. The kinetics of reduction reaction can be studied from UV-Visible spectroscopy. Fig. 3b shows UV-Visible measurements at various time intervals that are at 10, 20, 40, 60 and 80, at 60 and at 80 min. Thereafter there is no increase in peak intensity indicating completion of reaction.
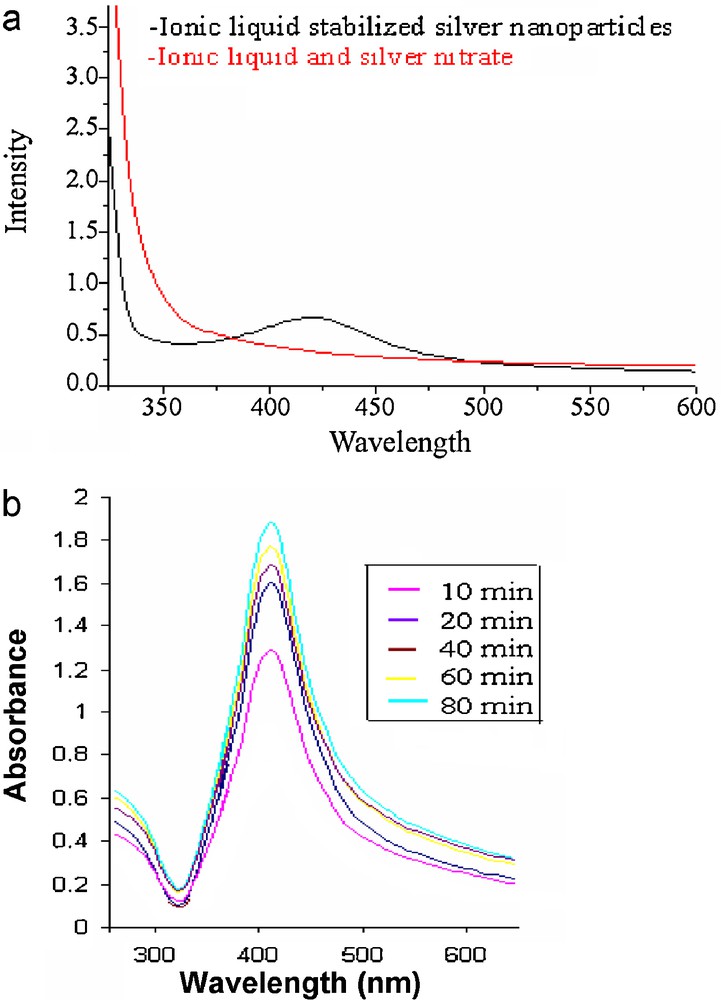
(a) UV-Visible spectra of water-IL micro emulsion and IL stabilized SNPs. (b) Kinetics of formation of SNPs from UV-Visible study.
Further, to get closer insight into SNPs formation, some kinetic parameter can be evaluated. From kinetic point of view, Ag+ reduction by hydrazine in water-IL micro emulsion can be described by following equation:
(1) |
(2) |
(3) |
From equation (3) linear logarithmic ln A∞−At/A∞ vs plot performs that Ag+ reduction reaction obeys first order kinetics.
Fig. 4a and b show TEM and SAED images of synthesized IL stabilized SNPs. The morphology of nanoparticles is highly variable with spherical and occasionally rod like nanoparticles observed on the micrograph. The TEM micrograph suggests that particle diameter ranged from 2 to 20 nm. The scale bar is 100 nm. The selected area electron diffraction pattern shows the particles were highly crystalline with zero valent FCC silver, representing (111), (200), (220), and (311) crystal planes and aggregation of each particle does not noticeably occur.

(a) TEM micrograph of IL stabilized silver Nan particles (SNPs). (b) SAED pattern of IL stabilized SNPs.
Fig. 5 shows PSD of histogram of colloidal dispersion of SNPs. The PSD shows particles are distributed in between 2 to 10 nm. Fig. 6 shows the zeta potential of IL stabilized silver nanoparticles to be 24.6 eV. This shows that particles were having positive charge on their surface.
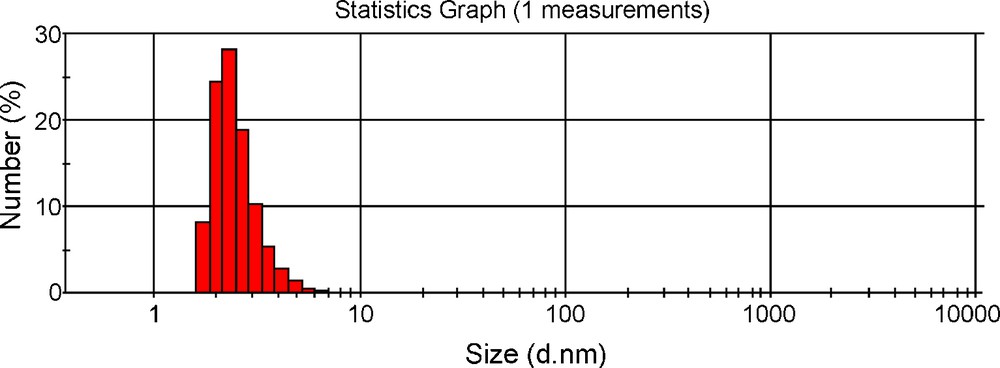
Particle size distribution of IL stabilized silver Nan particles (SNPs) showing histogram.
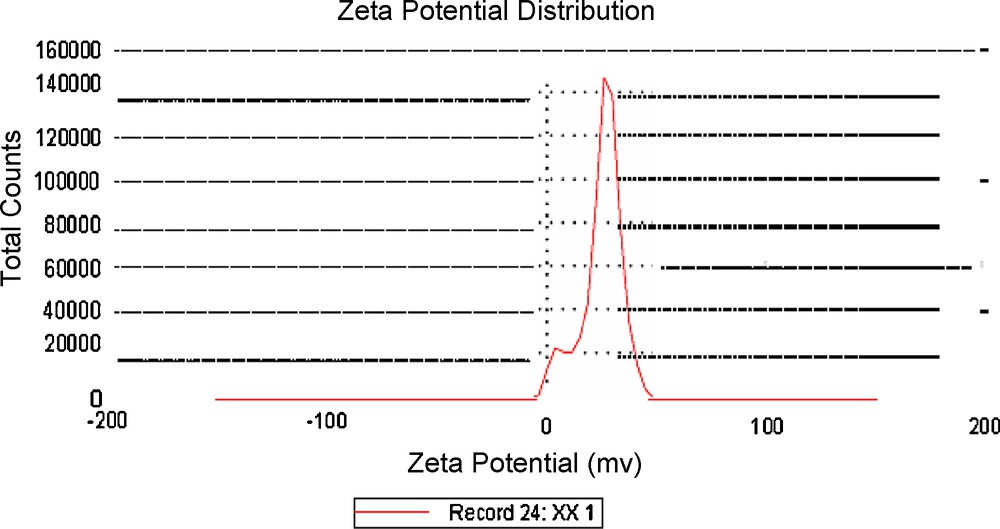
Zeta potential measurement of IL stabilized silver Nan particles (SNPs).
The antibacterial activity of synthesized hybrid materials against etalon strains of three different groups of bacteria E. coli (gram negative bacteria), S. aureus (gram positive bacteria) and P. aeruginosa (non ferment gram negative bacteria) has been studied. The results of antibacterial activity with zone of inhibition are tabulated in Table 1. The MIC is 10 μl. Control shows no zone of inhibition, this indicates the antimicrobial activity is due to IL stabilized SNPs. Fig. 7a, b and c antibacterial effect of E. coli, S. aureus and P. aeruginosa. E. coli shows zones of inhibition of 8, 12 and 15 mm. S. aureus shows zones of inhibition of 10, 15 and 20 mm and P. aeruginosa shows zones of inhibition of 3, 5 and 11 mm for 10, 15 and 20 μl, respectively. This vast difference may be due to the susceptibility of the organism used in the present study. The maximum antibacterial activity was recorded for S. aureus. These results can be interpreted on the basis of the possible mechanisms, all emphasizing the dependence of bacterial action of SNPs on their size, dose, and morphology. Effectively, different mechanisms for bacterial action of SNPs are the following: (i) Ag+ ions are supposed to bind to sulfhydryl groups, which lead to protein denaturation by the reduction of disulfide bonds; (ii) Ag+ can complex with electron donor groups containing sulfur, oxygen, or nitrogen that are normally present as thiols or phosphates on amino acids and nucleic acids. Also, SNPs have been found to attach to the surface of the cell membrane and disturb its function, penetrate bacteria, and release Ag; (iii) SNPs target the bacterial membrane, leading to a dissipation of the proton motive force. Thus, a decrease in the SNPs size can lead to an increase in the specific surface of a bactericidal specimen, inducing an increase in their ability to penetrate cell membrane, and thus improving antibacterial activity. It has also been reported that SNPs with size range of 1–10 nm attach to cell membrane and drastically disturb its proper function, like permeability and respiration, further on penetration, more damage is caused by interacting with sulfur and phosphorous containing compounds like DNA.
The results of antibacterial activity with zone of inhibition.
No. | Bacterial strains | Zone of inhibition by silver Nan particles (SNPs) | |||
Control (mm) | 10 μl (mm) | 15 μl (mm) | 20 μl (mm) | ||
1 | Escherichia coli | 0 | 8 | 12 | 15 |
2 | Staphylococcus aureus | 0 | 10 | 15 | 20 |
3 | Pseudomonas aeruginosa | 0 | 3 | 5 | 11 |
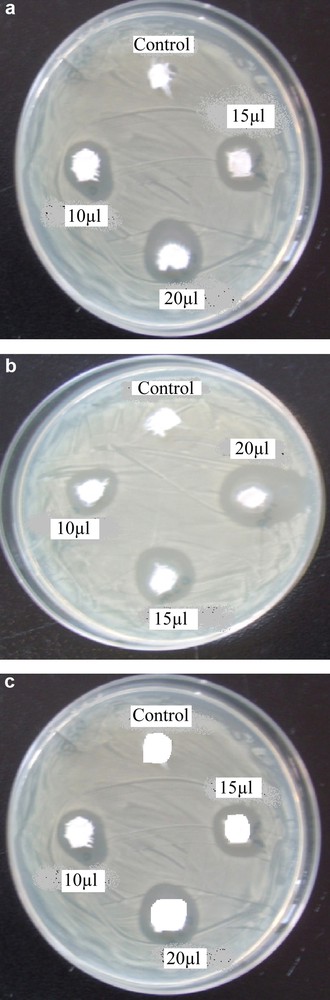
(a) Antimicrobial effect of Silver nanoparticles on Escherichia coli. (b) Antimicrobial effect of silver nanoparticles on Staphylococcus aureus. (c) Antimicrobial effect of Silver nanoparticles on Pseudomonas aeruginosa.
The higher antibacterial activity against S. aureus is probably driven by the difference in the structure of cell walls between gram-negative and gram-positive bacteria. The cell wall of gram-negative bacteria consists of lipids, proteins and lipopolysaccharides (LPS) that ensure more effective defense against biocides in comparison to gram-positive bacteria where the cell wall does not contain an outer membrane of LPS [32,33] thus leading to the higher antibacterial activity of the IL stabilized silver nanoparticle. It should be noted that an insignificant difference is observed in the size of the inhibition zones with increasing the silver concentration from 10, 15 and 20 μl.
The bactericidal activity is presumably due to certain changes in the membrane structure of bacteria cell wall as a result of the interaction with the embedded SNPs which leads to the increased membrane permeability of the bacteria and consequently, leading to their death [34].
4 Conclusion
In summary, the synthesis and kinetics of silver reduction by hydrazine hydrate in a water-IL micro emulsion were investigated. The ionic liquid itself can act as an electronic as well as steric stabilizer and depress particle growth. The highly structured ILs have strong effect on the morphology of particles formed. The appropriate concentration of IL can effectively produce finely dispersed SNPs with average size distribution of 2–20 nm. Silver nanoparticles exhibit excellent antibacterial activity against three bacterial strains S. aureus, E. coli. P. aeruginosa. These enhanced activities can be explained by the fact that silver nanoparticles are monodispersed to attach to the surface of cell and penetrate through the cell membrane which could make SNPs promising candidates for many biomedical applications.
Acknowledgement
One of the author (RSP) is grateful to the Department of Chemistry, Shivaji University, Kolhapur and University Grants Commission, New Delhi for SAP Fellowship. We are also grateful to the Department of Science and Technology, Government of India for DST-FIST programme facilities, Indian Institute of Technology, Mumbai for TEM characterization.